Tied Arch Bridge: Beauty, Engineering & Design
Tied arch bridges, celebrated for their distinctive aesthetic and structural efficiency, represent a pinnacle in bridge engineering. The "bowstring arch bridge," a common variant, exemplifies how the arch's horizontal thrust is contained by a tension tie, negating the need for substantial abutments and enabling construction on less stable ground. This design principle is crucial in projects overseen by organizations such as the American Institute of Steel Construction (AISC), where material specifications and construction standards are meticulously defined. Finite Element Analysis (FEA) plays a vital role in the design process, allowing engineers to accurately model and predict the structural behavior of the tied arch bridge under various loading conditions. Pioneers like Ammann & Whitney, the firm responsible for the Bayonne Bridge's original design, have significantly contributed to the evolution and refinement of tied arch bridge technology, setting benchmarks for future infrastructure projects.
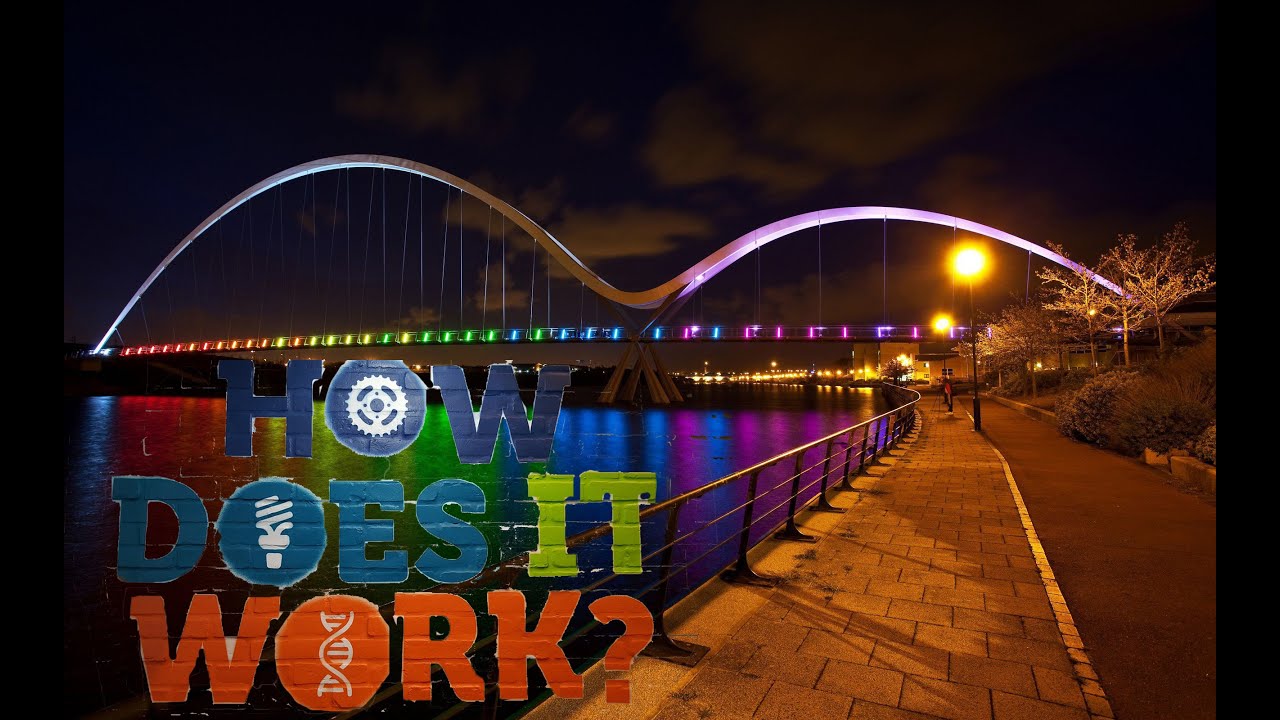
Image taken from the YouTube channel The Structures Guy , from the video titled How Do Tied-arch Bridges Work? .
Tied arch bridges, structures of captivating beauty and impressive engineering, stand as testaments to human ingenuity. This section serves as an introduction to these remarkable feats of engineering, laying the foundation for a comprehensive exploration of their design, mechanics, and historical significance.
Defining the Tied Arch Bridge
At its core, a tied arch bridge is an arch bridge in which the outward horizontal forces (thrust) of the arch(es) are borne as tension by a contained tie rather than by the ground or bridge foundations. This critical distinction allows tied arch bridges to be constructed even where the ground is of insufficient strength to resist horizontal thrust, making them suitable for various geological conditions.
Key Characteristics of a tied arch bridge include:
- A distinct arch rib, which is the primary compression element.
- A tie, typically constructed of high-strength steel, that connects the arch ends and resists the arch's thrust.
- Spandrel columns or hangers, which transfer the deck load to the arch.
Structurally, the arch functions by transferring vertical loads into compressive forces along its curved profile. The tie then absorbs the horizontal component of these forces, preventing the arch from spreading. This clever design distributes the loads efficiently, enabling the bridge to support significant weight.
Advantages over other bridge types are numerous. They include:
- Suitability for sites with poor soil conditions.
- Reduced need for massive abutments, lowering construction costs.
- Aesthetically pleasing designs, enhancing the visual appeal of their surroundings.
A Glimpse into History
The evolution of the tied arch bridge is a fascinating journey marked by innovation and refinement. While rudimentary arch bridges have existed for millennia, the modern tied arch bridge began to emerge in the late 19th and early 20th centuries.
Notable milestones in tied arch bridge design include:
- The development of high-strength steel, which enabled the construction of longer spans.
- Advances in structural analysis techniques, allowing engineers to predict bridge behavior with greater accuracy.
- The introduction of new construction methods, such as segmental construction, which facilitated the building of complex arch shapes.
Key innovations include the use of prestressed concrete in tie construction and the development of more efficient arch geometries.
Influential figures such as Gustav Eiffel (though known more for truss and frame design) and later bridge engineers who refined arch theory contributed significantly to the development and widespread adoption of tied arch bridge technology.
Purpose and Roadmap
This overview aims to provide a comprehensive understanding of tied arch bridges, suitable for a broad audience.
It is designed for:
- Civil engineering students.
- Practicing engineers.
- Architects.
- Anyone with an interest in bridge design and construction.
This article will delve into the structural mechanics, design considerations, analysis techniques, and construction methods associated with tied arch bridges. Furthermore, the overview will examine case studies of iconic tied arch bridges around the world, showcasing their unique features and engineering achievements. By the end, readers will gain a solid appreciation for the elegance, efficiency, and enduring appeal of tied arch bridges.
Deconstructing the Structure: Key Components and Mechanics
Tied arch bridges achieve their structural prowess through a carefully orchestrated interplay of distinct components. Understanding the role and mechanics of each element is crucial to appreciating the overall elegance and efficiency of this bridge design. This section will dissect the essential structural elements of a tied arch bridge, explaining their individual roles and how they interact to ensure stability and load-bearing capacity.
The Arch: The Primary Compression Element
The arch is the defining visual and structural element of a tied arch bridge. It acts as the primary compression member, efficiently transferring vertical loads into compressive forces.
Arch Geometry and Types
The geometry of the arch significantly influences the bridge's structural behavior. Common arch forms include parabolic, circular, and catenary curves. Each geometry distributes compressive forces differently, impacting the overall efficiency and stability of the structure.
A parabolic arch, for instance, ideally distributes load evenly under uniformly distributed vertical loads. While other geometries may be chosen for aesthetic reasons or site constraints, understanding their effect on force distribution is paramount.
Compression Forces
The arch's curved shape allows it to convert vertical loads into compressive forces directed along its axis. This compressive force is highest at the arch's base and decreases towards the crown. The magnitude of the compressive force is directly proportional to the applied load and the arch's curvature.
The arch must be designed to withstand these compressive forces without failure. Material selection and cross-sectional dimensions are crucial considerations.
Buckling Prevention
A significant concern in arch design is buckling – a form of structural instability where the arch deflects laterally under compressive load. Engineers employ various strategies to mitigate this risk.
These strategies include: increasing the arch's cross-sectional area, providing lateral bracing, optimizing the arch's shape to reduce stress concentrations.
Advanced buckling analysis is performed to ensure the arch's stability under all anticipated loading conditions.
The Tie: Resisting Horizontal Thrust
The tie is a critical component that distinguishes a tied arch bridge from a traditional arch bridge. It resists the outward horizontal thrust generated by the arch, preventing the arch from spreading.
Material Selection
High-strength steel is the most common material for tie construction due to its exceptional tensile strength. Other materials, such as steel cables or even fiber-reinforced polymers, can also be used.
The chosen material must possess sufficient tensile capacity to withstand the substantial tension forces generated by the arch's thrust.
Tension Forces
The tie is subjected to significant tensile forces, equal in magnitude and opposite in direction to the arch's horizontal thrust. Accurately calculating these tension forces is essential for ensuring structural integrity.
These forces are greatest at the tie's ends, where it connects to the arch supports. The tie must be designed to resist these tensile forces without yielding or fracturing.
Anchorage
Properly anchoring the tie ends is paramount to the bridge's overall stability. The anchorage system must securely transfer the tensile forces from the tie to the supports or foundations.
Common anchorage methods include: bolted connections, welded connections, post-tensioned anchor blocks.
The design of the anchorage must account for stress concentrations and prevent premature failure.
The Deck: The Roadway
The deck provides the roadway or pathway for traffic and pedestrians. It is supported by the arch and tie via spandrel columns or hangers.
Materials and Construction
Reinforced concrete is a commonly used material for deck construction due to its durability, strength, and ability to be cast into various shapes. Steel decks are also used, particularly for longer spans or where weight is a critical factor.
The deck may be constructed in situ (cast in place) or precast in segments and then assembled on site.
Load Distribution
The deck distributes the applied loads – from vehicles, pedestrians, and other sources – to the arch and tie members. The load distribution is influenced by the deck's stiffness, the spacing of the spandrel columns/hangers, and the overall bridge geometry.
An efficient load distribution ensures that the arch and tie are loaded evenly, maximizing their structural capacity.
Spandrel Columns/Hangers: Connecting Arch to Deck
Spandrel columns or hangers act as vertical connectors between the deck and the arch. They transfer vertical loads from the deck to the arch, allowing the arch to effectively carry the weight of the deck and the traffic it supports.
Function
The primary function of spandrel columns/hangers is to transfer vertical loads. They act as compression members (columns) or tension members (hangers), depending on the specific design and the relative positions of the deck and arch. These components are carefully designed to withstand the applied forces.
Configuration
The spacing and arrangement of spandrel columns are critical considerations in bridge design. Closely spaced columns result in a more uniform load distribution but may increase construction costs. The column spacing must be optimized to balance load distribution with economic factors.
The material properties of spandrel columns must also be carefully considered. Steel and reinforced concrete are common choices.
Lateral Bracing: Ensuring Stability
Lateral bracing is an essential element for ensuring the overall stability of the tied arch bridge, particularly against lateral loads such as wind and seismic forces.
Design Principles
Lateral bracing enhances the bridge's torsional rigidity and provides stability against wind loads. These are typically installed between the arches and/or between the tie girders. These bracing systems prevent the arches and tie girders from buckling or twisting under load.
Effective lateral bracing is crucial for maintaining the bridge's structural integrity and preventing catastrophic failure under extreme conditions.
Blueprint for Success: Design Considerations
The design of a tied arch bridge demands meticulous attention to a multitude of factors to guarantee safety, longevity, and efficiency. This phase goes beyond mere aesthetics, delving into the complex interplay of forces, materials, and environmental conditions. Successful design hinges on a comprehensive understanding of these elements and their intricate relationships.
Load Distribution Analysis: Understanding the Impact of Various Loads
Accurate load distribution analysis is the cornerstone of a robust tied arch bridge design. Engineers must meticulously account for all potential loads the structure will bear throughout its lifespan. This encompasses a wide range of forces, each with unique characteristics and implications.
Dead Load
Dead load refers to the static weight of the bridge itself, including all structural components, roadway surfacing, and permanent fixtures. This load is constant and must be precisely calculated. Accurate estimation of dead load is essential, as it forms the baseline for all subsequent load calculations and structural design decisions.
Live Load
Live load encompasses the dynamic and variable forces imposed by traffic, pedestrians, and other transient elements. This load varies significantly over time and must be determined based on anticipated traffic volume, vehicle types, and pedestrian density. Code specifications provide guidelines for minimum live load requirements, but engineers must also consider potential future increases in traffic volume.
Wind Load
Wind load represents the forces exerted by wind pressure on the bridge structure. These forces can be significant, particularly on bridges with large exposed surfaces. Aerodynamic effects must be carefully considered, as wind can induce vibrations, oscillations, and even catastrophic failure. Wind tunnel testing and computational fluid dynamics (CFD) simulations are often employed to assess wind load effects accurately.
Seismic Load
Seismic load accounts for the dynamic forces generated by earthquakes. These forces can be particularly challenging to design for, as they are unpredictable and can induce complex structural responses. Seismic design requires a thorough understanding of earthquake dynamics, soil conditions, and the bridge's natural frequencies. Advanced analysis techniques, such as time history analysis, are used to simulate the bridge's response to earthquake ground motions.
Bending Moment and Shear Force: Understanding Stress Distribution Patterns
Understanding the distribution of bending moment and shear force is paramount in ensuring structural integrity. These internal forces arise within the bridge members as a result of applied loads. Careful analysis of these patterns dictates the appropriate size and reinforcement of structural elements, preventing overstress and potential failure. Accurate calculation of bending moments and shear forces is crucial for optimizing material usage and enhancing the bridge's load-carrying capacity.
Stability and Stiffness: Key Parameters for Structural Integrity
Stability and stiffness are two fundamental parameters that govern the overall structural integrity of a tied arch bridge. Stability refers to the bridge's ability to resist buckling and other forms of instability under load. Stiffness, on the other hand, relates to the bridge's resistance to deformation and deflection. A bridge must possess adequate stability and stiffness to ensure its safety and serviceability.
Buckling Analysis
Buckling analysis is a crucial aspect of stability design, particularly for the arch, which is primarily subjected to compressive forces. This analysis identifies the critical buckling load, which is the load at which the arch becomes unstable and deflects laterally. Advanced methods, such as eigenvalue buckling analysis and nonlinear buckling analysis, are used to accurately determine the buckling load and to design the arch to prevent buckling.
Material Properties: Selecting the Right Materials
The selection of appropriate materials is a critical decision in tied arch bridge design. The chosen materials must possess adequate strength, durability, and resistance to environmental degradation. Careful consideration must be given to the material's mechanical properties, such as yield strength, tensile strength, and fatigue resistance.
Yield Strength and Tensile Strength
Yield strength and tensile strength define the material's performance limits and the safety factors that must be applied. Yield strength is the stress at which the material begins to deform permanently. Tensile strength is the maximum stress the material can withstand before fracturing. Engineers must ensure that the stresses in the bridge members remain below the yield strength under normal operating conditions and below the tensile strength under extreme loading conditions.
Fatigue
Fatigue is the weakening of a material due to repeated loading. Bridges are subjected to continuous cycles of loading and unloading from traffic, wind, and other sources. Over time, these cycles can lead to the formation of cracks and eventual failure. Fatigue analysis is used to evaluate the effects of repeated loading on structural components and to ensure that the bridge has a sufficient fatigue life.
Stress Concentration
Stress concentrations occur at geometric discontinuities, such as corners, holes, and welds. At these locations, the stress is significantly higher than the average stress in the member. Stress concentrations can lead to premature failure if not properly addressed. Engineers use finite element analysis (FEA) and other techniques to identify stress concentrations and to design the bridge to mitigate their effects.
Bridge Design Codes and Standards: Ensuring Adherence to Regulations
Adherence to relevant bridge design codes and standards is paramount for ensuring the safety and reliability of tied arch bridges. These codes and standards provide guidelines for load calculations, material selection, structural analysis, and construction practices. Compliance with these regulations is essential for obtaining permits and approvals and for ensuring that the bridge meets minimum safety requirements. Examples of such standards include the AASHTO LRFD Bridge Design Specifications.
Modeling the Marvel: Analysis and Simulation Techniques
The design and construction of a tied arch bridge rely heavily on sophisticated analysis and simulation techniques. These tools allow engineers to predict structural behavior, optimize designs, and ensure safety before construction even begins. By employing these methods, potential issues can be identified and addressed early in the design process, leading to more efficient and reliable bridge structures.
Finite Element Analysis (FEA): A Deep Dive into Computational Modeling
Finite Element Analysis (FEA) has become an indispensable tool in modern bridge engineering. FEA allows engineers to divide a complex structure into smaller, discrete elements and analyze their interaction under various loading conditions.
This approach provides a highly detailed understanding of stress distribution, deformation patterns, and overall structural response. The accuracy and precision offered by FEA are essential for optimizing designs and ensuring structural integrity.
Software Tools for FEA
Several powerful software tools are available for conducting FEA of tied arch bridges.
SAP2000 is a widely used structural analysis program known for its versatility and robust capabilities. It can handle complex bridge geometries, material properties, and loading scenarios, making it suitable for a wide range of FEA applications.
ANSYS is another industry-leading FEA software that offers advanced simulation capabilities. With its extensive element library and nonlinear analysis features, ANSYS is particularly useful for analyzing complex phenomena such as buckling, fatigue, and seismic response.
The selection of the appropriate software depends on the specific requirements of the project and the complexity of the analysis.
CAD (Computer-Aided Design): Precision in Design and Visualization
Computer-Aided Design (CAD) software plays a crucial role in creating accurate and detailed models of tied arch bridges. CAD tools enable engineers to visualize the structure in three dimensions, refine design parameters, and generate precise drawings for fabrication and construction.
The use of CAD enhances collaboration among designers, fabricators, and contractors, improving overall project efficiency and minimizing errors.
Software Tools for CAD
Various CAD software packages are employed in bridge design, each offering unique features and capabilities.
AutoCAD Civil 3D is a comprehensive civil engineering design software that provides tools for creating intelligent 3D models of bridges and other infrastructure projects. It supports Building Information Modeling (BIM) workflows, allowing for seamless integration of design, analysis, and construction data.
Revit Structure is another popular BIM software that focuses on structural design and documentation. It allows engineers to create parametric models of bridges, perform clash detection, and generate detailed construction drawings.
The choice of CAD software depends on the specific needs of the project, the level of detail required, and the integration with other software tools.
By combining FEA and CAD, engineers can create highly accurate and detailed models of tied arch bridges, enabling them to optimize designs, predict performance, and ensure the safety and durability of these iconic structures.
Lessons from Landmarks: Case Studies of Iconic Tied Arch Bridges
The study of existing tied arch bridges provides invaluable insight into successful design principles, innovative construction techniques, and long-term performance characteristics. By examining notable examples from around the world, engineers and designers can glean critical knowledge applicable to future projects. These case studies serve as tangible demonstrations of theoretical concepts, highlighting both the strengths and potential challenges associated with this elegant structural form.
Tyne Bridge: A Detailed Examination as an Iconic Example
The Tyne Bridge, spanning the River Tyne in Newcastle upon Tyne, England, stands as a quintessential example of a tied arch bridge. Completed in 1928, its robust design and striking aesthetics have made it a symbol of the city. The bridge features a parabolic arch, constructed from steel, which efficiently distributes compressive forces.
The tie, also made of high-strength steel, effectively resists the horizontal thrust generated by the arch. The bridge’s load-bearing capacity and durability have been proven over decades of heavy use, a testament to its sound engineering principles.
Key Design Features and Lessons
The Tyne Bridge’s success can be attributed to several key design features. The parabolic arch ensures optimal stress distribution, minimizing bending moments within the arch itself. The robust tie system, securely anchored at both ends, effectively contains the arch’s thrust, preventing outward movement.
Furthermore, the bridge’s steel construction provides both strength and ductility, allowing it to withstand significant loads and environmental stresses. Lessons learned from the Tyne Bridge include the importance of selecting appropriate arch geometry, ensuring adequate tie capacity, and utilizing high-quality materials.
Burrard Street Bridge: Analysis of its Design and Structural Characteristics
Vancouver's Burrard Street Bridge, completed in 1932, provides another compelling case study in tied arch bridge design. This elegant structure showcases a balanced blend of form and function, seamlessly integrating into the city's urban landscape.
Its distinctive Art Deco detailing, along with its robust structural components, underscore the importance of aesthetics in bridge design.
Structural Analysis and Aesthetic Integration
The Burrard Street Bridge demonstrates how tied arch bridges can be aesthetically pleasing without compromising structural integrity. The bridge's arch geometry is carefully chosen to minimize material usage while maximizing load-carrying capacity.
The tie system, concealed within the bridge deck, maintains a clean and uncluttered appearance. The bridge’s Art Deco embellishments, such as the decorative pylons and intricate metalwork, enhance its visual appeal. This case study highlights the importance of considering both structural performance and aesthetic integration in bridge design.
Daniel Hoan Memorial Bridge (Hoan Bridge): Insightful Review of a Prominent Tied Arch Bridge
The Daniel Hoan Memorial Bridge, more commonly known as the Hoan Bridge, in Milwaukee, Wisconsin, offers a valuable perspective on long-span tied arch bridge construction. This bridge, which spans the Milwaukee River, is a significant transportation artery for the city.
Its design incorporates several innovative features that address the challenges associated with supporting a large volume of traffic over a considerable distance.
Innovation and Span Considerations
The Hoan Bridge's design showcases innovative solutions for managing the stresses inherent in long-span tied arch structures. The bridge's arch is constructed from high-strength steel, allowing it to support the weight of the deck and traffic loads efficiently.
The tie system is designed to withstand significant tensile forces, ensuring the stability of the entire structure. This case study highlights the importance of carefully considering span length and load requirements when designing tied arch bridges, as well as the value of employing advanced materials and construction techniques.
Sydney Harbour Bridge: Comparative Analysis to a Similar Structure (Suspension Bridge)
While the Sydney Harbour Bridge is often mistakenly classified as a tied arch, it is actually an arch bridge that transfers its load via supports down to the ground and water, rather than a true tied arch design, which is self-supporting. However, a comparative analysis with suspension bridges can offer valuable insights into the relative advantages and disadvantages of each structural system.
Distinguishing Arch Bridges from Suspension Bridges
Unlike suspension bridges, which rely on cables suspended between towers to support the deck, arch bridges utilize a curved structure to transfer loads to abutments at either end. While both types of bridges can span considerable distances, they differ significantly in their structural behavior and aesthetic appearance.
The Sydney Harbour Bridge, as an arch bridge, exemplifies the strength and stability of this structural form. A comparison with suspension bridges highlights the importance of selecting the appropriate bridge type based on site conditions, load requirements, and aesthetic considerations.
Bayonne Bridge: Discussion of its Modification and Design Evolution
The Bayonne Bridge, connecting Bayonne, New Jersey, with Staten Island, New York, presents a unique case study in bridge modification and design evolution. Originally constructed in 1931, the bridge underwent a major reconstruction project to increase its vertical clearance, allowing larger ships to pass beneath it.
This project involved raising the bridge deck while maintaining the integrity of the existing arch structure.
Adapting to Changing Needs
The Bayonne Bridge reconstruction project demonstrates the adaptability of tied arch bridges to changing needs and requirements. The project involved careful planning and execution to minimize disruption to traffic flow and ensure the safety of workers and the public.
The successful completion of this project highlights the importance of considering future needs and potential modifications when designing bridges. Furthermore, it demonstrates the feasibility of adapting existing structures to meet evolving demands, extending their lifespan and maximizing their value.
Building the Dream: Construction Techniques and Innovations
The construction of a tied arch bridge represents a significant engineering undertaking, demanding meticulous planning, precise execution, and innovative solutions to overcome inherent challenges. The process involves a complex interplay of logistical considerations, material handling, and specialized erection methods, all orchestrated to bring the design vision to life. From the initial fabrication of individual components to the final connection of the structure, each stage requires careful attention to detail and adherence to stringent safety protocols.
This section delves into the diverse construction techniques employed in building tied arch bridges, shedding light on the ingenuity and resourcefulness required to realize these iconic structures.
Erection Methods: Assembling the Arch
The erection method chosen for a tied arch bridge significantly impacts the overall construction process, influencing everything from equipment requirements to project timelines. Several approaches are commonly employed, each with its own advantages and limitations, depending on site conditions, span length, and other project-specific factors.
Segmental Construction: This technique involves fabricating the arch in discrete segments, which are then transported to the construction site and assembled in place. Segmental construction offers several benefits, including the ability to prefabricate components off-site, minimizing disruption to traffic and the surrounding environment.
The segments are typically lifted into position using cranes or other specialized lifting equipment and then connected using bolted or welded connections. This method is particularly well-suited for long-span bridges, where the size and weight of the arch make it impractical to erect the entire structure in one piece.
Cantilever Erection: In this approach, the arch is erected in a cantilevered fashion, with each segment supported by temporary supports until it is connected to the adjacent segment. This method allows for the construction of the arch without the need for extensive falsework or scaffolding.
Cantilever erection requires careful monitoring of stresses and deflections to ensure the stability of the structure throughout the construction process. It is often used in situations where access to the construction site is limited or where the bridge spans a deep gorge or waterway.
Full Arch Erection: This method involves assembling the entire arch on temporary supports before lifting it into its final position. This approach is typically used for smaller tied arch bridges, where the weight and dimensions of the arch allow for it to be transported and erected as a single unit.
Full arch erection requires significant lifting capacity and precise alignment to ensure a proper fit. However, it can be a faster and more efficient method than segmental construction for suitable projects.
Material Transport: Logistics of Scale
The transport of massive structural components to the construction site poses a significant logistical challenge, requiring careful planning and coordination. The size and weight of these components often necessitate the use of specialized transportation equipment and routes.
For components fabricated off-site, transportation options include trucks, barges, and railcars. The choice of transportation method depends on factors such as distance, accessibility, and the size and weight of the components.
Once at the construction site, the components must be carefully unloaded and staged for erection. This often requires the use of heavy-lift cranes and specialized rigging equipment.
Temporary Supports: The Unsung Heroes
Temporary supports play a critical role in the construction of tied arch bridges, providing stability and load-bearing capacity during the erection process. These temporary structures, often consisting of steel towers, falsework, or cable systems, are designed to support the weight of the arch and deck until the bridge is fully self-supporting.
The design of temporary supports requires careful consideration of the loads they will be subjected to, including the weight of the structure, wind loads, and seismic loads. The supports must be strong enough to withstand these loads without buckling or collapsing.
The installation and removal of temporary supports also require careful planning and execution. The supports must be installed in a manner that does not damage the permanent structure, and they must be removed in a controlled sequence to avoid inducing excessive stresses in the bridge.
The selection and design of temporary supports are critical to ensuring the safety and stability of the bridge during construction. They represent an essential, albeit often overlooked, element of the overall construction process.
Beyond Structure: Sustainability and Aesthetics
Tied arch bridges, while celebrated for their structural efficiency and load-bearing capacity, also present opportunities to integrate sustainable practices and aesthetic considerations into their design and construction. Moving beyond pure functionality, a holistic approach acknowledges the environmental impact and visual harmony these structures can achieve. This section explores how sustainability and aesthetics can be woven into the fabric of tied arch bridge projects.
Sustainability: Minimizing Environmental Footprint
Sustainable bridge design focuses on minimizing the environmental impact throughout the bridge's lifecycle, from material sourcing to decommissioning. This requires a multi-faceted approach, considering material selection, construction practices, and long-term operational efficiency.
Material Selection and Life Cycle Assessment
Choosing materials with lower embodied energy and environmental impact is paramount. High-strength steel, while essential for the tie, should be sourced from manufacturers with sustainable practices. Concrete mixes can incorporate recycled aggregates and supplementary cementitious materials, reducing the carbon footprint associated with cement production.
Life Cycle Assessment (LCA) is a valuable tool for evaluating the environmental impact of different material choices and construction methods. LCA considers the entire lifecycle of a material or process, from raw material extraction to end-of-life disposal, providing a comprehensive understanding of its environmental footprint. LCA helps guide decision-making towards more sustainable options.
Construction Practices and Waste Reduction
Construction activities can have a significant environmental impact, including air and water pollution, noise pollution, and habitat disruption. Implementing best practices to minimize these impacts is crucial.
This includes employing erosion and sediment control measures, using low-emission construction equipment, and minimizing waste generation. Effective waste management strategies, such as recycling and reuse, can significantly reduce the amount of waste sent to landfills.
Innovative construction techniques, such as prefabrication, can also contribute to sustainability by reducing on-site construction time, minimizing disruption to the surrounding environment, and improving material efficiency.
Durability and Long-Term Performance
A durable bridge is a sustainable bridge. Designing for long-term performance reduces the need for frequent repairs and replacements, minimizing the consumption of resources and the associated environmental impacts.
This requires careful consideration of factors such as corrosion resistance, fatigue strength, and resistance to environmental degradation. Using high-quality materials and implementing robust protection systems are essential for ensuring long-term durability.
Aesthetics: Integrating Visual Appeal
Aesthetics play a crucial role in the acceptance and integration of a tied arch bridge within its environment. A well-designed bridge can enhance the beauty of its surroundings and become a source of pride for the community.
Harmony with the Environment
The visual impact of a bridge should be carefully considered in relation to its surroundings. The bridge's form, color, and texture should complement the landscape and architectural context. Consideration should be given to the bridge's silhouette against the skyline and its interaction with natural elements, such as water and vegetation.
Form and Proportions
The form and proportions of a tied arch bridge can significantly influence its aesthetic appeal. A well-proportioned arch, with graceful curves and balanced spans, can create a sense of elegance and harmony. The spacing and arrangement of spandrel columns or hangers can also contribute to the overall visual aesthetic.
Lighting and Visual Effects
Lighting can be used to enhance the visual appeal of a tied arch bridge, particularly at night. Strategic lighting can highlight the bridge's architectural features, create dramatic effects, and improve safety for pedestrians and vehicles. The choice of lighting fixtures and colors should be carefully considered to complement the bridge's design and the surrounding environment.
Colored lights can enhance the aesthetic, but light pollution needs to be mitigated by directing the source lights downward to avoid undesirable spill.
Community Engagement
Involving the community in the design process can help ensure that the bridge reflects local values and preferences. Public consultations and design workshops can provide valuable feedback and foster a sense of ownership and pride in the project. Community engagement can help ensure that the bridge is not only functional and safe but also aesthetically pleasing and culturally sensitive.
Guiding the Future: Professional Organizations and Standards
Tied arch bridge design and construction are governed by a complex web of professional organizations and industry standards. These entities play a vital role in ensuring the safety, durability, and overall quality of these critical infrastructure assets. By setting benchmarks for engineering practice, promoting research, and fostering collaboration, they shape the future of tied arch bridge technology.
American Society of Civil Engineers (ASCE): A Cornerstone of Engineering Standards
The American Society of Civil Engineers (ASCE) stands as a prominent figure in the civil engineering domain. It has a significant impact on bridge engineering through its development and dissemination of standards, guidelines, and best practices.
ASCE's role extends far beyond simply providing technical documents. The society provides a framework for ethical conduct and promotes professional development for civil engineers. This ensures that practitioners adhere to the highest standards of competence and integrity.
ASCE's Manuals and Reports on Engineering Practice are particularly relevant to tied arch bridge engineering. These publications offer comprehensive guidance on design, construction, and maintenance procedures. They reflect the current state-of-the-art and incorporate lessons learned from past projects.
Furthermore, ASCE hosts numerous conferences and workshops that bring together engineers, researchers, and policymakers to share knowledge and discuss emerging challenges in the field. This collaborative environment fosters innovation and drives continuous improvement in tied arch bridge technology.
AASHTO: Setting the Standard for Bridge Design
The American Association of State Highway and Transportation Officials (AASHTO) plays a vital role in standardizing bridge design practices in the United States. AASHTO doesn't focus solely on tied arch bridges, but the AASHTO Load and Resistance Factor Design (LRFD) Bridge Design Specifications directly impacts how engineers approach critical design elements, material selection, and load calculations.
While ASCE provides broad guidelines and general engineering practices, AASHTO focuses on the specifics of bridge design and construction for transportation infrastructure. The AASHTO LRFD specifications provide detailed requirements for load factors, resistance factors, and material properties. These are critical components for ensuring the structural integrity and safety of bridges subjected to highway traffic loads.
The AASHTO LRFD specifications are continually updated to reflect advances in engineering knowledge and construction techniques. This includes addressing new materials, improved analytical methods, and lessons learned from bridge performance and failures. Adherence to AASHTO standards is often a legal requirement for bridge projects funded by federal or state transportation agencies.
International Association for Bridge and Structural Engineering (IABSE): A Global Perspective
The International Association for Bridge and Structural Engineering (IABSE) offers a global perspective on bridge engineering. It promotes the exchange of knowledge and experience among engineers, researchers, and practitioners from around the world.
IABSE's conferences, publications, and working groups provide a platform for discussing innovative design concepts, construction techniques, and maintenance strategies for tied arch bridges. By bringing together experts from diverse backgrounds, IABSE fosters cross-cultural learning and promotes the adoption of best practices worldwide.
IABSE's publications, such as the journal Structural Engineering International, feature cutting-edge research and case studies of notable tied arch bridge projects from around the globe. These resources offer valuable insights into the challenges and opportunities associated with designing and building these complex structures.
IABSE also plays a key role in developing international standards and guidelines for bridge design and construction. While these standards may not be directly enforceable in all countries, they serve as a valuable reference for engineers seeking to adopt best practices and ensure the safety and durability of their projects.
Other Key Organizations
While ASCE, AASHTO, and IABSE are particularly prominent, other organizations also contribute to the advancement of tied arch bridge engineering. These include:
- The American Institute of Steel Construction (AISC): Develops standards and specifications for steel structures, including those used in tied arch bridges.
- The American Concrete Institute (ACI): Provides guidance on the design and construction of concrete structures, including bridge decks and foundations.
- National Highway Institute (NHI): Provides training and education programs for bridge engineers and inspectors.
These organizations work collaboratively to ensure that tied arch bridges are designed, constructed, and maintained to the highest standards of safety and performance.
The Importance of Continuing Education and Professional Development
The field of bridge engineering is constantly evolving, with new materials, technologies, and design methods emerging regularly. It is essential for engineers to engage in continuing education and professional development to stay abreast of these advancements and maintain their competence.
Organizations such as ASCE, IABSE, and NHI offer a wide range of courses, workshops, and conferences that provide opportunities for engineers to expand their knowledge and skills. These programs cover topics such as advanced structural analysis, seismic design, bridge inspection, and rehabilitation techniques.
By actively participating in continuing education and professional development, engineers can ensure that they are equipped to meet the challenges of designing, constructing, and maintaining safe and durable tied arch bridges for future generations.
Video: Tied Arch Bridge: Beauty, Engineering & Design
Frequently Asked Questions
What makes a tied arch bridge different from other arch bridges?
Tied arch bridges are unique because their arch ends are connected by a tension-resisting tie, often the bridge deck itself. This tie counteracts the horizontal thrust generated by the arch, meaning the bridge doesn't need massive abutments to resist spreading. Other arch bridges rely on the ground for that resistance.
Why are tied arch bridges considered aesthetically pleasing?
The design of a tied arch bridge allows for a clean, open appearance. Because the arch's thrust is contained internally, the supporting structure can be more slender. This elegance, combined with the graceful curve of the arch, often makes a tied arch bridge visually appealing.
Where are tied arch bridges typically used?
Tied arch bridges are frequently used where strong foundations are unavailable or undesirable. This can be in situations with poor soil conditions or when crossing waterways that require a wide, unobstructed span without intermediate piers.
What are some of the key engineering considerations in designing a tied arch bridge?
Key considerations when designing a tied arch bridge include the strength and stability of the tie member, the load distribution throughout the arch, and preventing buckling of the arch. Precise calculations are needed to ensure the tied arch bridge can safely withstand traffic and environmental loads.
So, the next time you're crossing a tied arch bridge, take a moment to appreciate the elegant dance of tension and compression, the thoughtful engineering, and the sheer beauty of the design. It's more than just a way to get from point A to point B; it's a testament to human ingenuity and a striking addition to the landscape.