Stored Energy: Types, Uses, and Future Trends
Stored energy plays a crucial role in modern energy systems, enabling a more reliable and efficient use of resources, while the need for such systems is growing as society's demand for energy is ever increasing. Electrochemical batteries represent one critical type of stored energy, utilizing chemical reactions to store electrical energy for later use. Power grids benefit significantly from stored energy solutions, enhancing grid stability and enabling better integration of renewable energy sources. Organizations such as the U.S. Department of Energy are actively involved in researching and promoting advanced stored energy technologies to improve energy efficiency and reduce carbon emissions. In transportation, electric vehicles (EVs) rely on stored energy to provide a clean and sustainable alternative to traditional combustion engines, marking a significant trend in the evolution of stored energy applications.
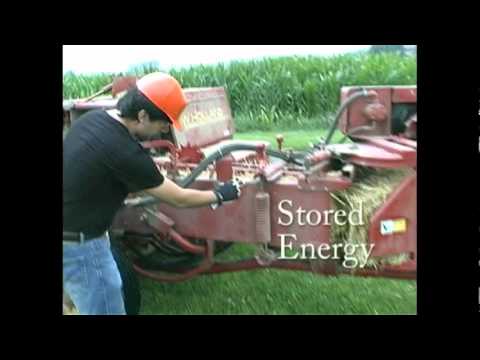
Image taken from the YouTube channel AgSafety4u , from the video titled Mechanical Hazards - Stored Energy .
The Dawn of Energy Storage: Powering a Sustainable Tomorrow
Energy storage is rapidly transitioning from a niche technology to a central pillar of modern energy systems. Its accelerating importance stems from the urgent need to address the intermittency of renewable energy sources and enhance grid resilience. The integration of energy storage technologies is no longer an option but a necessity for a stable and sustainable energy future.
The Critical Role of Energy Storage
Energy storage systems play a pivotal role in balancing energy supply and demand, addressing the inherent variability of renewable energy sources like solar and wind power.
By storing excess energy generated during periods of high production and low demand, energy storage ensures that this energy is available when needed. This capability is critical for maintaining grid stability and reliability, preventing blackouts and ensuring a continuous power supply.
A Spectrum of Energy Storage Technologies
A diverse range of energy storage technologies are available, each with its unique characteristics and applications. These technologies can broadly be categorized into:
- Mechanical: Pumped hydro storage and compressed air energy storage are examples of large-scale mechanical storage solutions.
- Electrochemical: This includes batteries (lithium-ion, flow batteries, etc.) that convert chemical energy into electricity.
- Thermal: Thermal energy storage utilizes heat or cold storage mediums to store and release thermal energy.
- Chemical: Hydrogen energy storage is one example of chemical energy storage.
Each of these technologies plays a vital role in enabling a flexible and resilient energy system. They contribute significantly to the integration of renewable energy and the efficient management of energy resources.
Decarbonizing the Energy Sector: The Transformative Potential
Energy storage holds immense potential for decarbonizing the energy sector. By enabling the widespread adoption of renewable energy sources, energy storage helps reduce reliance on fossil fuels.
This shift is crucial for mitigating climate change and achieving global sustainability goals.
Furthermore, energy storage supports the electrification of various sectors, including transportation and heating, further reducing carbon emissions. The transformative impact of energy storage extends beyond environmental benefits, fostering economic growth and energy independence. It is a key enabler of a cleaner, more sustainable, and resilient energy future.
Energy Storage Technologies: A Comprehensive Overview
[The Dawn of Energy Storage: Powering a Sustainable Tomorrow Energy storage is rapidly transitioning from a niche technology to a central pillar of modern energy systems. Its accelerating importance stems from the urgent need to address the intermittency of renewable energy sources and enhance grid resilience. The integration of energy storage technologies is key to unlocking a cleaner, more reliable, and sustainable energy future, and in this section, we will delve into the specifics.]
This section provides an in-depth exploration of various energy storage technologies, shedding light on their operational principles, advantages, and disadvantages. Understanding these technologies is crucial for navigating the complex landscape of energy storage and making informed decisions about their implementation.
Pumped Hydro Storage (PHS)
Pumped Hydro Storage (PHS) stands as one of the oldest and most widely implemented forms of large-scale energy storage.
It works on a simple yet effective principle: using surplus electricity to pump water from a lower reservoir to a higher reservoir, effectively storing energy in the form of gravitational potential energy. When energy demand is high, the water is released, flowing back down to the lower reservoir and driving turbines to generate electricity.
Advantages: PHS boasts mature technology, long lifespan (often exceeding 50 years), and the ability to provide significant grid-stabilization services.
Disadvantages: However, PHS requires specific geographical conditions (elevation differences and suitable reservoir locations) and has a significant environmental impact due to land inundation and alteration of river ecosystems.
Its construction and operation are also capital-intensive and can face regulatory hurdles.
Compressed Air Energy Storage (CAES)
Compressed Air Energy Storage (CAES) is another large-scale energy storage technology that stores energy by compressing air and holding it in underground caverns or reservoirs.
During periods of low electricity demand, air is compressed and stored. When demand increases, the compressed air is released, heated, and expanded through a turbine to generate electricity.
Different CAES configurations exist, including:
-
Diabatic CAES: Uses natural gas to heat the compressed air before expansion, resulting in lower energy efficiency.
-
Adiabatic CAES: Recovers the heat generated during compression and stores it for later use in the expansion phase, significantly improving efficiency.
-
Isothermal CAES: Aims to maintain a constant temperature during compression and expansion, further enhancing efficiency but increasing system complexity.
Advantages: CAES can offer large storage capacities and relatively long discharge durations.
Disadvantages: CAES requires suitable geological formations for underground storage, and diabatic configurations have environmental concerns related to greenhouse gas emissions. Adiabatic and isothermal CAES systems are still under development and face technological and cost challenges.
Flywheel Energy Storage
Flywheel Energy Storage systems store energy in the form of kinetic energy by accelerating a rotor (flywheel) to a very high speed. Energy is stored as rotational kinetic energy.
When energy is needed, the flywheel's rotational energy is converted back into electricity using a generator.
Advantages: Flywheels have a rapid response time, high power density, and long lifespan with minimal degradation.
Disadvantages: However, they typically have limited energy storage capacity and high self-discharge rates due to friction. They are best suited for short-duration applications like frequency regulation and power quality improvement.
Gravity Energy Storage
Gravity Energy Storage (GES) is an emerging concept that leverages the principles of gravitational potential energy storage, similar to PHS, but with greater flexibility in location.
GES systems typically involve lifting solid masses (e.g., concrete blocks, rocks) to a higher elevation using electric motors during periods of excess electricity.
When energy is needed, the masses are lowered, and the potential energy is converted back into electricity via generators.
Advantages: GES systems can be located in various terrains and offer potential environmental benefits compared to PHS.
Disadvantages: They face challenges related to efficiency, cost, and scalability, as well as the visual impact of large-scale installations.
Batteries (Electrochemical Energy Storage)
Electrochemical Energy Storage, commonly known as batteries, is a cornerstone of modern energy storage systems, providing versatile solutions for a wide range of applications.
Batteries store energy through reversible chemical reactions involving the flow of ions between electrodes. Various battery chemistries exist, each with unique characteristics.
Lithium-ion Batteries
Lithium-ion batteries are currently the dominant technology for portable electronics and electric vehicles, owing to their high energy density, high power density, and relatively long cycle life.
However, they have concerns about cost, thermal management, and the use of critical materials.
Lead-acid Batteries
Lead-acid batteries are a mature and cost-effective technology widely used in automotive applications and backup power systems.
They have lower energy density and shorter cycle life than lithium-ion batteries but are more tolerant of overcharging and deep discharging.
Nickel-Metal Hydride (NiMH) Batteries
Nickel-Metal Hydride (NiMH) batteries have been used in hybrid electric vehicles.
They offer better energy density than lead-acid batteries but are heavier and more expensive than lithium-ion batteries.
Sodium-ion Batteries
Sodium-ion batteries are emerging as a potential alternative to lithium-ion batteries, offering the advantage of using more abundant and less expensive materials.
However, they currently have lower energy density and cycle life compared to lithium-ion batteries.
Flow Batteries
Flow batteries offer scalable energy and power capabilities, making them suitable for grid-scale energy storage applications.
They store energy in liquid electrolytes contained in external tanks, allowing for independent scaling of energy capacity and power rating.
Flow batteries have long lifespans and can tolerate deep discharging, but they have lower energy density and higher system complexity than other battery technologies.
Hydrogen Energy Storage
Hydrogen Energy Storage involves storing energy in the form of hydrogen, which can be produced through electrolysis using excess electricity. The hydrogen can then be stored in tanks, underground caverns, or pipelines.
When energy is needed, the hydrogen can be used in fuel cells to generate electricity or burned in combustion turbines.
Advantages: Hydrogen offers high energy density and the potential for long-duration energy storage.
Disadvantages: Hydrogen energy storage faces challenges related to production efficiency, storage infrastructure, and transportation costs.
Thermal Energy Storage (TES)
Thermal Energy Storage (TES) stores energy in the form of heat or cold.
-
Sensible Heat Storage utilizes the heat capacity of materials like water, rocks, or soil to store thermal energy.
-
Latent Heat Storage uses phase-change materials (PCMs) that absorb or release heat during melting or solidification.
-
Thermochemical Energy Storage involves reversible chemical reactions that store and release heat.
Advantages: TES can improve energy efficiency in buildings, industrial processes, and district heating/cooling systems.
Disadvantages: TES faces challenges related to energy density, heat loss, and material stability.
Capacitors/Supercapacitors/Ultracapacitors
Capacitors, supercapacitors, and ultracapacitors store energy electrostatically by accumulating electric charge on electrodes.
They offer very high power density, rapid charge/discharge rates, and long cycle life.
Advantages: Capacitors are suitable for applications requiring short bursts of energy, such as regenerative braking in vehicles and power quality improvement.
Disadvantages: Capacitors have relatively low energy density compared to batteries.
Solid-State Batteries
Solid-State Batteries are being developed as a safer and more energy-dense alternative to lithium-ion batteries.
They replace the liquid electrolyte with a solid electrolyte, eliminating the risk of leakage and thermal runaway.
Advantages: Solid-state batteries promise higher energy density, improved safety, and longer lifespan.
Disadvantages: Solid-state batteries are currently under development and face challenges related to manufacturing scalability and cost.
Applications of Energy Storage: Powering the Future
Energy storage technologies, as detailed previously, unlock a spectrum of applications that extend far beyond simply holding electrical charge.
This section delves into the diverse ways these technologies are reshaping various sectors, underscoring their versatility and profound impact on how we generate, distribute, and utilize energy. From stabilizing the electrical grid to powering electric vehicles and ensuring backup power, energy storage is a vital catalyst for a more resilient and sustainable future.
Grid-Scale Energy Storage: Stabilizing the Electrical Backbone
Grid-scale energy storage is revolutionizing how we manage and operate electrical grids. These large-scale installations provide essential services that enhance grid stability, improve efficiency, and facilitate the integration of renewable energy sources.
Frequency Regulation: Maintaining Grid Equilibrium
One of the critical roles of grid-scale storage is frequency regulation. Electrical grids must maintain a consistent frequency (e.g., 60 Hz in North America) to ensure the stable operation of connected devices. Energy storage systems can rapidly inject or absorb power to counteract fluctuations in frequency, thereby preventing blackouts and equipment damage.
Peak Shaving: Flattening Demand Curves
Peak demand periods often strain grid infrastructure, leading to higher electricity prices and potential reliability issues. Energy storage systems enable peak shaving by storing energy during off-peak hours and releasing it during peak demand. This reduces the need for expensive and often polluting peaking power plants, resulting in cost savings and environmental benefits.
Renewable Energy Integration: Smoothing the Intermittency
Renewable energy sources like solar and wind are inherently intermittent, posing challenges for grid operators. Energy storage plays a pivotal role in integrating these variable resources by storing excess energy generated during periods of high production and releasing it when demand exceeds supply. This ensures a more reliable and consistent supply of renewable energy to the grid.
Black Start Capability: Restoring Power After Outages
In the event of a blackout, energy storage systems with black start capability can initiate the restoration of power without relying on external grid connections. This is particularly valuable in remote areas or during widespread outages caused by natural disasters or cyberattacks, significantly reducing recovery times.
Transportation: Fueling the Electric Vehicle Revolution
Energy storage is at the heart of the electric vehicle (EV) revolution, enabling the widespread adoption of clean and efficient transportation. Batteries, primarily lithium-ion, are the dominant energy storage technology in EVs and hybrid electric vehicles (HEVs).
Electric Vehicles (EVs): A Battery-Powered Future
EVs rely entirely on batteries for propulsion, offering significant advantages over traditional internal combustion engine vehicles. EVs produce zero tailpipe emissions, reducing air pollution and greenhouse gas emissions. Continuous improvements in battery technology are extending the driving range of EVs, making them increasingly practical for everyday use.
Hybrid Electric Vehicles (HEVs): Bridging the Gap
HEVs combine an internal combustion engine with an electric motor and battery pack. The battery provides supplemental power, improving fuel efficiency and reducing emissions. HEVs offer a transitional solution towards full electrification, appealing to consumers seeking a balance between environmental benefits and driving range.
Portable Electronics: Powering Our Mobile Lives
The proliferation of smartphones, laptops, tablets, and other portable devices would be impossible without efficient and compact energy storage. Lithium-ion batteries are the workhorse of the portable electronics industry, delivering high energy density and long cycle life in a small form factor.
Backup Power Systems: Ensuring Continuity During Outages
Uninterruptible power supplies (UPS) provide backup power in the event of a power outage, ensuring the continued operation of critical equipment and systems. UPS systems are widely used in data centers, hospitals, and other facilities where power interruptions can have severe consequences. Battery-based UPS systems provide immediate and reliable backup power, preventing data loss, equipment damage, and disruptions to critical services.
Off-Grid Power Systems: Empowering Remote Communities
Energy storage is essential for providing reliable and affordable electricity to communities without access to the grid. Off-grid power systems, often incorporating solar panels and battery storage, enable remote communities to access electricity for lighting, cooking, healthcare, and education. This empowers communities, fosters economic development, and improves the overall quality of life.
Key Players in Energy Storage: Organizations and Companies Leading the Charge
Energy storage technologies, as detailed previously, unlock a spectrum of applications that extend far beyond simply holding electrical charge. This section delves into the diverse ways these technologies are reshaping various sectors, underscoring their versatility and profound impact on how we generate, distribute, and consume energy. Examining the critical players that are essential in accelerating energy storage solutions.
The Pillars of Progress: A Multifaceted Ecosystem
The advancement of energy storage isn't the result of a single entity's efforts. Instead, it arises from a collaborative ecosystem. This ecosystem includes government bodies, research institutions, industry associations, and pioneering companies.
Their combined actions are propelling innovation and deployment across the globe.
Government and Research Organizations: Foundations for Innovation
Government organizations play a crucial role in funding research, establishing standards, and enacting policies. These actions create a conducive environment for energy storage technologies to thrive.
The U.S. Department of Energy (DOE), for example, invests heavily in R&D through its various offices and national laboratories. Their efforts span basic science to demonstration projects.
The National Renewable Energy Laboratory (NREL) provides cutting-edge research and analysis, accelerating the commercialization of energy storage solutions. Similarly, ARPA-E supports high-risk, high-reward projects. These projects have the potential to transform the energy landscape.
International entities, such as the European Commission and the International Renewable Energy Agency (IRENA), foster global collaboration. They also promote the adoption of sustainable energy technologies.
Academic Institutions: The Cradle of Breakthroughs
Universities are at the forefront of energy storage research, driving fundamental scientific discoveries and educating the next generation of experts.
MIT, Stanford University, and the University of California, Berkeley, stand out. Their faculty and researchers are pushing the boundaries of battery technology, materials science, and grid integration. These institutions contribute significantly to the pool of knowledge and talent.
This is essential for continued progress.
Industry Associations: Catalysts for Collaboration and Standardization
Industry associations serve as crucial platforms for collaboration, advocacy, and standardization within the energy storage sector. They represent the collective interests of their members, promoting best practices and driving market growth.
The Energy Storage Association (ESA) advocates for policies that support energy storage deployment. It also facilitates knowledge sharing among industry stakeholders. Battery Council International (BCI) focuses on lead-acid batteries and represents the interests of manufacturers and recyclers.
These groups collectively ensure responsible and sustainable practices.
Leading Companies: Translating Innovation into Reality
Ultimately, it is the leading companies that translate research and development into tangible products and services.
These companies are investing billions of dollars in manufacturing capacity, technology innovation, and project deployment, thereby driving the widespread adoption of energy storage.
Tesla, led by Elon Musk, is a household name and a driving force in the electric vehicle and battery storage markets. Their innovative products, such as the Powerwall and Megapack, have revolutionized the residential and grid-scale storage sectors, emphasizing the importance of aggressive innovation.
LG Chem and Panasonic are major battery manufacturers that supply cells for EVs and energy storage systems. Their scale of production and technological expertise is vital for meeting the growing demand for batteries. BYD, a Chinese company, is a vertically integrated manufacturer. They produce batteries, electric vehicles, and energy storage systems.
Fluence, a joint venture between Siemens and AES, focuses on grid-scale energy storage solutions. They deploy systems around the world. Stem provides intelligent energy storage solutions for commercial and industrial customers. It optimizes energy usage and reduces costs.
The Interconnected Web of Progress
These organizations and companies do not operate in isolation. They form an interconnected web, where research findings are translated into commercial products. Policies and regulations are crafted to promote innovation, and market demand drives further investment.
This collaborative ecosystem is essential for realizing the full potential of energy storage and building a more sustainable energy future.
Influential Figures in Energy Storage
Energy storage, as detailed previously, unlocks a spectrum of applications that extend far beyond simply holding electrical charge. The advancements are driven by the brilliant minds behind the technology. This section spotlights key individuals who have made, and continue to make, significant contributions to the field, shaping its trajectory and realizing its potential.
The Pioneers of Lithium-Ion Technology
The development of the lithium-ion battery, arguably the most transformative energy storage technology of our time, is a story of collaborative innovation. Three figures stand out as pivotal contributors, and were rightfully awarded the Nobel Prize in Chemistry in 2019 for their groundbreaking work.
John Goodenough (Deceased)
John Goodenough's contribution was fundamental to the lithium-ion battery's success. His work at Oxford University in the 1970s led to the identification of lithium cobalt oxide as a viable cathode material.
This discovery was a turning point, enabling the creation of batteries with significantly higher energy density than previous iterations. His insight paved the way for smaller, lighter, and more powerful devices, revolutionizing portable electronics and setting the stage for the electric vehicle revolution.
Stanley Whittingham
M. Stanley Whittingham's early work at Exxon in the 1970s laid the initial groundwork for lithium-ion batteries.
He pioneered the concept of using lithium ions to intercalate within a layered material, specifically titanium disulfide. This research was revolutionary because it enabled a reversible electrochemical reaction and facilitated the realization of high energy densities.
Although his initial battery design had limitations, Whittingham's work provided a crucial foundation for future advancements.
Akira Yoshino
Akira Yoshino built upon the discoveries of Goodenough and Whittingham to create the first commercially viable lithium-ion battery. Working at Asahi Kasei, Yoshino replaced the lithium metal anode with a carbonaceous material.
This replacement significantly improved safety and stability, making the batteries practical for mass production. His invention was a commercial breakthrough, transforming portable electronics and ultimately enabling the widespread adoption of electric vehicles.
Modern Visionaries: Driving Adoption and Innovation
Beyond the fundamental scientific discoveries, the field of energy storage benefits from those with the vision to translate research into real-world applications. These are the individuals who champion the technology and drive the industry forward.
Elon Musk
Elon Musk, as CEO of Tesla, has been instrumental in popularizing electric vehicles and driving demand for advanced battery technology. His company's commitment to long-range, high-performance EVs has pushed battery manufacturers to innovate and improve their products.
Tesla's Gigafactories, massive battery production facilities, have also played a key role in reducing battery costs through economies of scale. Musk's vision and leadership have accelerated the transition to electric mobility and positioned energy storage as a cornerstone of a sustainable energy future.
While Elon Musk deserves credit for popularizing EV adoption, he has also been accused of overpromising and underdelivering on certain claims. It's vital to maintain a balanced perspective regarding his role in the energy storage narrative.
The figures highlighted above represent just a fraction of the talented individuals contributing to the ongoing evolution of energy storage. Their collective efforts are critical in shaping a future powered by clean, reliable, and accessible energy.
Policies and Regulations Shaping the Energy Storage Landscape
Influential Figures in Energy Storage Energy storage, as detailed previously, unlocks a spectrum of applications that extend far beyond simply holding electrical charge. The advancements are driven by the brilliant minds behind the technology. This section spotlights key individuals who have made, and continue to make, significant contributions to the field of energy storage. But technology alone cannot propel the widespread adoption of energy storage. Government policies and regulations play a crucial, and often underestimated, role in shaping the energy storage landscape. These policies create the market conditions, incentives, and regulatory frameworks that either accelerate or hinder the deployment of energy storage technologies. This section will examine some of the most influential policies and regulations currently impacting the energy storage sector.
Energy Storage Mandates: Driving Deployment
Energy storage mandates are government regulations that require utilities or other entities to deploy a specific amount of energy storage capacity within a defined timeframe. These mandates are designed to overcome market barriers and kickstart the adoption of energy storage technologies. By creating a guaranteed market for energy storage, mandates incentivize investment and drive down costs.
For example, several states in the United States, including California, have established energy storage mandates. These mandates have spurred significant investment in grid-scale energy storage projects and have helped to accelerate the transition to a cleaner energy system. However, the effectiveness of mandates depends on their design. They must be carefully crafted to avoid unintended consequences, such as favoring specific technologies or creating market distortions.
Incentive Programs: Fueling Growth
Incentive programs, such as tax credits, subsidies, and financial incentives, are another powerful tool for promoting energy storage deployment. These programs reduce the upfront cost of energy storage systems, making them more economically attractive to consumers and businesses. The federal Investment Tax Credit (ITC) in the United States, for instance, has been instrumental in driving down the cost of solar energy and has also been extended to energy storage projects.
Similarly, many states and local governments offer their own incentive programs for energy storage. These programs can take various forms, including rebates, grants, and low-interest loans. The key to successful incentive programs is to design them in a way that is transparent, predictable, and targeted at the most impactful applications of energy storage.
Grid Interconnection Standards: Facilitating Integration
Grid interconnection standards define the technical requirements and procedures for connecting energy storage systems to the electrical grid. These standards are essential for ensuring the safe and reliable operation of the grid. Without clear and consistent interconnection standards, energy storage projects can face significant delays and uncertainties, hindering their deployment.
Many jurisdictions are now updating their grid interconnection standards to better accommodate energy storage. These updates often involve streamlining the interconnection process, reducing interconnection costs, and establishing clear guidelines for the technical requirements that energy storage systems must meet. A well-defined interconnection process is essential for creating a level playing field and encouraging the integration of energy storage into the grid.
FERC and Order 841: Federal Oversight
The Federal Energy Regulatory Commission (FERC) plays a critical role in regulating the transmission of electricity in the United States. FERC's decisions have a significant impact on the development and deployment of energy storage. One of the most important FERC orders related to energy storage is Order 841.
Order 841: Leveling the Playing Field
FERC Order 841 requires regional transmission organizations (RTOs) and independent system operators (ISOs) to remove barriers to the participation of energy storage resources in wholesale electricity markets. This order aims to create a level playing field for energy storage by allowing it to compete fairly with other resources, such as traditional power plants.
Order 841 has the potential to unlock significant value for energy storage by allowing it to provide a wide range of grid services, including frequency regulation, capacity, and energy arbitrage. However, the implementation of Order 841 has been uneven across different RTOs and ISOs, and further work is needed to fully realize its potential.
Net Metering Policies: The Solar-Plus-Storage Nexus
Net metering policies allow customers with solar panels to receive credit on their electricity bills for the excess electricity they send back to the grid. These policies have been a key driver of solar energy adoption. As battery storage becomes more affordable, there is growing interest in combining solar with battery storage.
Net metering policies can be adapted to accommodate solar-plus-storage systems. For example, some jurisdictions allow customers to receive net metering credit for the electricity they send back to the grid from their batteries, even if that electricity was originally generated by solar panels. However, the design of net metering policies for solar-plus-storage systems can be complex, and there is a need for clear and consistent rules to ensure that these systems are properly valued.
Ultimately, policies and regulations that thoughtfully encourage energy storage development are essential to unlock the full potential of this transformative technology. The combined effects of thoughtfully conceived, properly implemented, and consistently enforced policies could be a catalyst for a more sustainable and efficient energy future.
Future Trends in Energy Storage: What's on the Horizon?
Energy storage, as detailed previously, unlocks a spectrum of applications that extend far beyond simply holding electrical charge. The advancements are driven by the brilliant minds behind the technology. However, the full potential of energy storage is still unfolding, propelled by ongoing research, technological innovation, and evolving market demands. This section will explore the emerging trends and future directions that will shape the energy storage landscape in the coming years.
The Relentless Pursuit of Higher Energy Density
One of the primary drivers of innovation in the battery sector is the quest for increased energy density. This translates to storing more energy in the same volume or weight, leading to longer ranges for electric vehicles, extended runtimes for portable devices, and improved grid stability.
Next-generation battery technologies, such as solid-state batteries and lithium-sulfur batteries, promise to significantly improve upon the energy density of current lithium-ion batteries.
These advancements are essential for enabling the widespread adoption of electric transportation and realizing the full potential of renewable energy sources.
Driving Down Costs: The Key to Widespread Adoption
The high cost of energy storage has historically been a barrier to its widespread deployment. However, significant progress has been made in reducing battery costs over the past decade, driven by economies of scale, technological advancements, and increased competition.
Continued cost reductions are crucial for making energy storage accessible to a broader range of consumers and businesses.
This includes utility-scale projects, residential installations, and electric vehicle applications. Further research and development into cheaper materials, streamlined manufacturing processes, and innovative battery designs will play a vital role in achieving these goals.
Second-Life Batteries: A Circular Economy Approach
As the electric vehicle market matures, a growing number of batteries will reach the end of their useful life in automotive applications. Second-life batteries offer a sustainable and cost-effective solution by repurposing these batteries for less demanding applications, such as grid-scale energy storage.
By extending the lifespan of batteries, this approach reduces waste, conserves resources, and lowers the overall cost of energy storage.
Innovative business models and technologies are being developed to facilitate the collection, testing, and repurposing of second-life batteries.
This promotes a circular economy approach to energy storage.
Optimizing Performance with AI and Machine Learning
AI and machine learning are revolutionizing the way energy storage systems are managed and optimized. These technologies can be used to predict energy demand, optimize battery charging and discharging cycles, and improve overall system performance.
By analyzing vast amounts of data, AI and machine learning algorithms can identify patterns and trends that would be impossible for humans to detect. This enables more efficient and reliable operation of energy storage systems, leading to cost savings and improved grid stability.
The integration of AI and machine learning is expected to play an increasingly important role in the future of energy storage.
The Rise of Long-Duration Energy Storage (LDES)
While short-duration energy storage solutions, such as lithium-ion batteries, are well-suited for many applications, long-duration energy storage (LDES) is essential for providing reliable and affordable power during extended periods of low renewable energy generation.
LDES technologies, such as pumped hydro storage, compressed air energy storage, and advanced flow batteries, can store energy for hours, days, or even weeks.
This makes them ideal for balancing the variability of renewable energy sources and ensuring grid resilience.
As the penetration of renewable energy increases, the demand for LDES will continue to grow, driving innovation and investment in this critical area.
Video: Stored Energy: Types, Uses, and Future Trends
FAQ: Stored Energy
What are the main types of stored energy?
The main types of stored energy include mechanical (e.g., springs, flywheels), chemical (e.g., batteries, fuel), thermal (e.g., hot water tanks), and electrical (e.g., capacitors, supercapacitors). Each method uses different principles to accumulate and release energy.
How is stored energy used in everyday life?
Stored energy is essential. Batteries power our phones and cars. Dams store potential energy as water for hydroelectric power. Hot water tanks utilize thermal stored energy for showers. Even a wound-up toy uses mechanical stored energy to move.
What are some examples of future trends in energy storage?
Future trends focus on improving efficiency and sustainability. This includes developing advanced battery technologies like solid-state batteries, exploring thermal energy storage using phase-change materials, and creating larger, more efficient pumped hydro stored energy systems.
Why is energy storage becoming increasingly important?
Energy storage addresses the intermittent nature of renewable energy sources like solar and wind. Stored energy allows us to use clean energy when the sun isn't shining or the wind isn't blowing, leading to a more reliable and sustainable energy grid. It also facilitates electric vehicle adoption.
So, there you have it – a glimpse into the fascinating world of stored energy! From the batteries powering our phones to the pumped hydro keeping our grids stable, it's clear that this technology is vital. And with all the exciting innovations on the horizon, we can expect stored energy to play an even bigger role in shaping a cleaner and more sustainable future.