Hydrogen Charge: Fuel Cell EV Charging Guide
Hydrogen fuel cell electric vehicles (FCEVs), exemplified by models like the Toyota Mirai, represent a growing segment in the zero-emission vehicle market. These vehicles rely on hydrogen charge, a process involving the refueling of hydrogen gas into high-pressure tanks. Stations, such as those supported by organizations like the California Fuel Cell Partnership, facilitate this hydrogen charge, employing specialized dispensers engineered to safely and efficiently deliver hydrogen fuel. Moreover, the pressure at which hydrogen is delivered, often at 700 bar (10,000 psi), is a critical parameter governed by standards developed in collaboration with experts like those at the U.S. Department of Energy, ensuring optimal vehicle performance and safety.
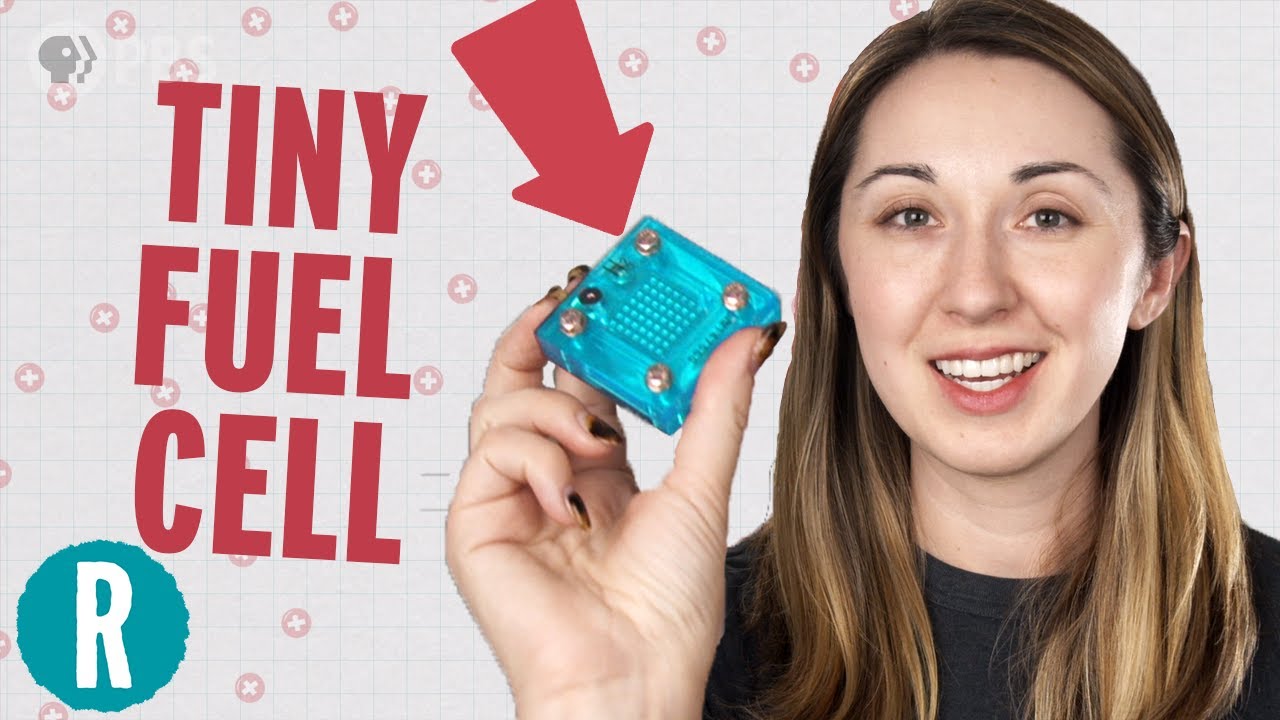
Image taken from the YouTube channel Reactions , from the video titled How Do Hydrogen Fuel Cells Work? .
Unlocking the Potential of Hydrogen as an Energy Carrier
Hydrogen stands poised to be a cornerstone of a sustainable energy future.
Its inherent versatility and clean-burning characteristics offer a compelling alternative to traditional fossil fuels.
This section will explore the transformative potential of hydrogen, its key stakeholders, and the landscape of challenges and opportunities that define its adoption.
Hydrogen's Role in a Sustainable Energy Future
Hydrogen's appeal lies in its abundance and its capacity to be produced from diverse sources, including renewable energy.
Unlike fossil fuels, the combustion of hydrogen produces primarily water vapor, significantly reducing greenhouse gas emissions.
This clean-burning property is crucial in mitigating climate change and improving air quality, especially in urban environments.
Moreover, hydrogen’s versatility extends beyond transportation.
It can be used in power generation, industrial processes (such as steel and chemical production), and even residential heating.
This multifaceted application makes hydrogen a vital component of a diversified energy portfolio, enhancing energy security and resilience.
Key Stakeholders in the Hydrogen Economy
The emerging hydrogen economy is a collaborative effort, driven by a diverse range of stakeholders.
Governments play a critical role in establishing supportive policies, setting emissions targets, and investing in research and infrastructure development.
Government incentives and regulations can accelerate the deployment of hydrogen technologies and stimulate market demand.
Industry is at the forefront of innovation, developing advanced hydrogen production technologies, fuel cell systems, and storage solutions.
Companies across various sectors, from energy and automotive to manufacturing and technology, are actively investing in hydrogen-related initiatives.
Research institutions provide the scientific foundation for technological advancements, pushing the boundaries of hydrogen production, storage, and utilization.
Universities and national laboratories are conducting cutting-edge research to improve the efficiency, durability, and cost-effectiveness of hydrogen technologies.
The successful development and deployment of hydrogen relies on effective collaboration among these key stakeholders, fostering a synergistic ecosystem that drives innovation and accelerates market adoption.
Challenges and Opportunities in Hydrogen Adoption
While hydrogen offers tremendous potential, its widespread adoption faces significant challenges.
High production costs remain a major barrier, particularly for "green hydrogen" produced from renewable energy sources.
Developing robust and cost-effective hydrogen storage and transportation infrastructure is also crucial.
Additionally, public awareness and acceptance are essential for overcoming perceptions and building confidence in hydrogen as a safe and reliable energy carrier.
Despite these challenges, the opportunities are substantial.
Advancements in electrolysis technologies, economies of scale in production, and strategic investments in infrastructure are paving the way for a more competitive and accessible hydrogen market.
The transition to a hydrogen economy represents a significant step towards a cleaner, more sustainable energy future, with the potential to transform industries, create jobs, and improve the environment.
Key Players Driving the Hydrogen Revolution: Innovators and Leaders
The momentum behind the hydrogen revolution is not solely technological. It's driven by a diverse cast of individuals and organizations dedicated to realizing hydrogen's potential. From the laboratory to the boardroom and halls of government, these key players are pushing the boundaries of what's possible.
This section will focus on the individuals and organizations who are at the forefront of hydrogen technology. They include policy and infrastructure development, from researchers to corporate executives and government officials.
Research and Development: The Foundation of Progress
The quest for efficient and sustainable hydrogen solutions starts in the research lab. Scientists and engineers are constantly striving to improve hydrogen production, storage, and utilization technologies. Their breakthroughs are crucial to making hydrogen a viable energy carrier.
Advancing Hydrogen Production Efficiency
Electrolysis, the process of using electricity to split water into hydrogen and oxygen, holds immense promise for green hydrogen production. Researchers are working on enhancing the efficiency and reducing the cost of various electrolysis methods. These include Proton Exchange Membrane (PEM), alkaline, and solid oxide electrolysis.
Scientists are refining Steam Methane Reforming (SMR) techniques. The aim is to minimize carbon emissions through carbon capture and storage.
Fuel Cell Design: Performance and Durability
The efficiency and durability of fuel cells are critical to their widespread adoption. Engineers are continuously refining fuel cell designs. This includes optimizing materials, improving catalyst performance, and enhancing thermal management.
These efforts are directly impacting the performance and lifespan of fuel cells. The hope is that they will make them more competitive with traditional combustion engines.
Safe and Efficient Storage Solutions
Hydrogen storage remains a significant challenge. Researchers are exploring various approaches to overcome this barrier. These include compressed gas storage, liquid hydrogen storage, and material-based storage methods. Materials-based solutions, such as metal hydrides and advanced sorbents, offer the potential for safer and more compact storage.
Corporate Leadership: Investing in the Future
Beyond the lab, visionary corporate leaders are investing heavily in hydrogen technologies. They are driving the commercialization of hydrogen solutions and shaping the future of the energy landscape.
Plug Power, Ballard Power Systems, Hyundai, and Toyota
Companies like Plug Power and Ballard Power Systems are at the forefront of fuel cell technology development and deployment. They are providing fuel cell solutions for various applications, including transportation, stationary power, and material handling.
Automotive giants like Hyundai and Toyota are heavily invested in Fuel Cell Electric Vehicles (FCEVs). Their commitment signals a strong belief in hydrogen's potential as a transportation fuel. Their strategies involve not only developing FCEVs, but also investing in hydrogen refueling infrastructure.
Corporate Strategies and Investments
These companies are pursuing diverse strategies to capitalize on the growing hydrogen market. This includes developing partnerships, acquiring innovative technologies, and expanding their product portfolios. Their investments are crucial to scaling up hydrogen production, distribution, and utilization.
Government and Advocacy: Shaping the Policy Landscape
Government policies and advocacy efforts play a critical role in creating a supportive ecosystem for hydrogen adoption. Policymakers are enacting regulations, providing incentives, and investing in research and infrastructure to accelerate the transition to a hydrogen economy.
Policy Frameworks and Incentives
Government officials are developing comprehensive policy frameworks that address the challenges and opportunities associated with hydrogen. These frameworks include carbon pricing mechanisms, tax incentives, and subsidies to encourage investment in hydrogen technologies.
Public Awareness and Acceptance
Advocates are working to raise public awareness and acceptance of hydrogen as a viable energy source. They are educating the public about the benefits of hydrogen. They are also addressing concerns about safety and environmental impact. These advocates play a crucial role in shaping public opinion and fostering support for hydrogen initiatives.
Technical Experts: Overcoming Practical Challenges
The successful deployment of hydrogen technologies requires overcoming a range of practical challenges. Skilled engineers are essential for developing safe, efficient, and cost-effective hydrogen infrastructure.
Addressing Infrastructure Barriers
Engineers are tackling the technical barriers to hydrogen infrastructure development head-on. They are working on optimizing the design and operation of hydrogen refueling stations, pipelines, and storage facilities. Safety is paramount, and engineers are developing and implementing robust safety protocols to ensure the safe handling and use of hydrogen.
Building the Hydrogen Highway: Refueling Infrastructure
The vision of a hydrogen-powered future hinges significantly on establishing a robust and accessible refueling infrastructure. Currently, hydrogen refueling stations are far less prevalent than conventional gas stations or even electric vehicle charging stations. This scarcity poses a considerable barrier to the widespread adoption of Fuel Cell Electric Vehicles (FCEVs). Overcoming this hurdle requires strategic planning, technological advancements, and significant investment.
Global Landscape of Hydrogen Refueling Stations
The global distribution of hydrogen refueling stations is uneven, with certain regions taking the lead. Asia, particularly Japan and South Korea, boasts a significant number of operational stations, driven by strong government support and ambitious hydrogen strategies. Europe, especially Germany, also has a growing network, spurred by initiatives to decarbonize transportation. In North America, California leads the way, incentivizing station development to support its growing FCEV market.
However, even in these leading regions, the density of refueling stations is still relatively low. The need for a more extensive and geographically diverse network is paramount to alleviate range anxiety and encourage consumer confidence in FCEV technology. Planned expansion involves strategic placement along major transportation corridors and in urban centers, but progress needs acceleration.
Case Study: Shell Hydrogen Station, Sacramento, CA
Examining a real-world hydrogen refueling station offers valuable insights into the practicalities and challenges of operating such a facility. The Shell hydrogen station in Sacramento, CA, serves as a pertinent example. It represents a pioneering effort to provide convenient refueling options for FCEV drivers in the region.
The station utilizes a combination of hydrogen delivery methods and on-site compression technology to ensure a reliable supply. However, challenges remain. The cost of hydrogen production and delivery, along with the operational expenses of maintaining the station, impact the price of hydrogen fuel. Addressing these economic factors is crucial to make hydrogen refueling more competitive with gasoline and electricity.
Understanding Pressure Levels: 350 bar vs. 700 bar
Hydrogen refueling stations typically operate at two primary pressure levels: 350 bar and 700 bar. The choice of pressure level directly impacts the range and fuel capacity of FCEVs.
-
350 bar: This lower pressure is suitable for vehicles with smaller hydrogen tanks. While less demanding on storage technology, it can limit the vehicle's overall range.
-
700 bar: This higher pressure allows for greater hydrogen storage capacity within the same tank volume, resulting in increased driving range. However, 700 bar systems require more robust and expensive tank technology, as well as more energy intensive compression.
The industry trend is moving towards 700 bar systems to maximize vehicle range and meet consumer expectations. But the infrastructure needs to support this higher pressure must be robust and reliable.
The Refueling Process: Time and Safety
Refueling Time: A Key Competitive Factor
One of the primary advantages of FCEVs over Battery Electric Vehicles (BEVs) is their refueling time. Hydrogen refueling can typically be completed in approximately 3-5 minutes, comparable to the time it takes to fill a gasoline tank.
This significantly faster refueling time addresses a major pain point for EV drivers. The ability to quickly replenish fuel is a compelling advantage for consumers who value convenience and minimal downtime.
Safety Protocols: Prioritizing Public and Environmental Wellbeing
Safety is paramount in hydrogen refueling operations. Stringent safety protocols are implemented to mitigate any potential risks associated with hydrogen's flammability.
These protocols include:
-
Leak detection systems: Continuously monitor for hydrogen leaks, triggering alarms and shutdown procedures if necessary.
-
Flame detectors: Identify and respond to any potential fires.
-
Emergency shutdown systems: Allow for immediate shutdown of the station in the event of an emergency.
-
Proper ventilation: Prevents the accumulation of hydrogen in enclosed spaces.
Furthermore, rigorous training programs are essential for station personnel to ensure they are equipped to handle any situation safely and effectively. Continuous improvement and adherence to strict safety standards are critical for building public trust in hydrogen technology.
From Production to Power: Hydrogen Production and Storage Methods
Building a hydrogen economy requires not only efficient fuel cells and robust infrastructure but also, critically, effective methods for producing and storing hydrogen. The source of hydrogen and how it's held until use significantly impacts its overall sustainability and economic viability.
Hydrogen Production Methods: A Diverse Landscape
Hydrogen production isn't a monolith. Different methods exist, each with its own advantages, disadvantages, and environmental footprint. Understanding these methods is crucial to evaluating the true potential of hydrogen as a clean energy carrier.
Electrolysis: Splitting Water with Electricity
Electrolysis uses electricity to split water (H₂O) into its constituent elements: hydrogen and oxygen. If the electricity comes from renewable sources like solar or wind, the resulting hydrogen is considered "green hydrogen," representing a truly carbon-free fuel source.
There are several types of electrolyzers, each with varying efficiencies and suitability for different applications:
-
Proton Exchange Membrane (PEM) Electrolysis: PEM electrolyzers are known for their high efficiency and ability to operate at varying power loads, making them suitable for integration with intermittent renewable energy sources.
-
Alkaline Electrolysis: A more mature technology, alkaline electrolysis is generally less expensive but also less efficient than PEM. It's often used in large-scale hydrogen production facilities.
-
Solid Oxide Electrolysis Cells (SOEC): SOECs operate at high temperatures, which can increase efficiency, but also present challenges in terms of material durability and system complexity. SOEC is an emerging technology that can utilize waste heat to boost the electrolysis process.
Steam Methane Reforming (SMR): A Bridge to the Future?
Steam methane reforming (SMR) is the most common method of hydrogen production today. It involves reacting natural gas (methane) with high-temperature steam to produce hydrogen and carbon dioxide.
While SMR is a relatively inexpensive and well-established process, it also generates significant CO₂ emissions, making it a less desirable long-term solution for a sustainable hydrogen economy.
Carbon capture and storage (CCS) technologies can be integrated with SMR to reduce its carbon footprint, but these technologies are still under development and add to the overall cost. "Blue Hydrogen" is produced from SMR with CCS technology.
Hydrogen Storage: Taming a Volatile Element
Storing hydrogen presents unique challenges due to its low density and tendency to leak. Safe and efficient storage is essential for widespread adoption.
Compressed Hydrogen Gas: A Widely Used Approach
Compressing hydrogen gas is a relatively straightforward method for increasing its density for storage. However, even at high pressures (e.g., 700 bar), the energy density of compressed hydrogen is still lower than that of gasoline.
- Challenges: High-pressure tanks require robust materials and careful design to prevent leaks and ensure safety. The energy required for compression also reduces the overall efficiency of the hydrogen fuel cycle.
Beyond Compression: Exploring Alternative Storage Methods
Researchers are actively exploring alternative storage methods to overcome the limitations of compressed gas:
-
Liquid Hydrogen: Cooling hydrogen to extremely low temperatures (-253°C) allows it to be stored as a liquid, significantly increasing its density. However, liquefaction is an energy-intensive process, and boil-off losses (evaporation of hydrogen) can occur during storage and transportation.
-
Materials-Based Storage (Hydrides): This approach involves using materials like metal hydrides or chemical hydrides to absorb and release hydrogen. Materials-based storage offers the potential for higher energy density and improved safety compared to compressed gas, but research is ongoing to develop materials with suitable properties for practical applications.
Selecting the optimal method for hydrogen production and storage is vital to creating a hydrogen value chain that is both economically practical and environmentally responsible.
Harnessing Hydrogen's Energy: Fuel Cell Technology Explained
From production to power: Hydrogen production and storage methods Building a hydrogen economy requires not only efficient fuel cells and robust infrastructure but also, critically, effective methods for producing and storing hydrogen. The source of hydrogen and how it's held until use significantly impacts its overall sustainability and economic viability. Let's dive into fuel cell technology and discuss some of the most common types.
Types of Fuel Cells
Fuel cells are electrochemical devices that convert the chemical energy of a fuel (in this case, hydrogen) directly into electricity. This process is remarkably efficient and produces only water and heat as byproducts, when using pure hydrogen.
Several types of fuel cells exist, each with its own characteristics and optimal applications. These include:
- Proton Exchange Membrane Fuel Cells (PEMFCs)
- Solid Oxide Fuel Cells (SOFCs)
- Alkaline Fuel Cells (AFCs)
For automotive applications and portable power, however, PEMFCs are generally favored.
Proton Exchange Membrane Fuel Cells (PEMFCs)
PEMFCs operate at relatively low temperatures (around 80°C), allowing for quick start-up times and dynamic response. This makes them well-suited for vehicles where power demand fluctuates rapidly.
At the heart of a PEMFC is a proton-conducting membrane, typically made of a polymer material. Hydrogen gas flows over the anode, where it is separated into protons and electrons.
The protons pass through the membrane to the cathode, while the electrons travel through an external circuit, generating electricity. At the cathode, the protons, electrons, and oxygen from the air combine to form water.
The overall reaction is: 2H2 + O2 → 2H2O + electricity + heat.
PEMFCs are known for their high power density, compact size, and relatively simple design, contributing to their popularity in FCEVs. They are also used in stationary power generation and backup power systems.
Applications: Fuel Cell Electric Vehicles (FCEVs)
Fuel Cell Electric Vehicles (FCEVs) represent a compelling alternative to Battery Electric Vehicles (BEVs) in the quest for zero-emission transportation. FCEVs use PEMFCs to generate electricity to power an electric motor.
Unlike internal combustion engines, FCEVs produce no tailpipe emissions other than water vapor. This makes them a key technology in reducing greenhouse gas emissions and improving air quality in urban areas.
Operation of FCEVs
The operation of an FCEV is relatively straightforward:
-
Hydrogen gas is stored onboard in a high-pressure tank.
-
The hydrogen is fed into the fuel cell stack, where it reacts with oxygen from the air to produce electricity.
-
The electricity powers an electric motor, which drives the wheels.
-
The only byproduct is water, which is emitted as vapor.
FCEVs also typically include a small battery pack to provide supplemental power during acceleration and to capture energy during regenerative braking. This hybrid approach enhances efficiency and performance.
FCEV Market: Advantages and Disadvantages Compared to Battery Electric Vehicles (BEVs)
FCEVs and BEVs represent two distinct approaches to electric mobility, each with its own set of advantages and disadvantages.
Advantages of FCEVs:
- Longer Range: FCEVs generally offer a longer driving range compared to BEVs.
- Faster Refueling: Refueling an FCEV with hydrogen takes only a few minutes, comparable to gasoline vehicles, while charging a BEV can take significantly longer.
- Reduced Weight: FCEV powertrains can be lighter than BEV powertrains.
Disadvantages of FCEVs:
- Infrastructure: The hydrogen refueling infrastructure is currently limited compared to the established network of charging stations for BEVs.
- Cost: Hydrogen fuel can be more expensive than electricity, depending on the region and production method.
- Hydrogen Production: The environmental benefits of FCEVs depend on how the hydrogen is produced; "grey" hydrogen produced from natural gas can have a significant carbon footprint.
- Durability: Ensuring the longevity and durability of fuel cell stacks remains a key challenge.
Emissions: A nuanced comparison
While both FCEVs and BEVs produce zero tailpipe emissions, it’s crucial to consider the entire lifecycle. The production of batteries for BEVs has a considerable environmental impact, and the source of electricity used to charge them matters.
Similarly, the production method of hydrogen significantly affects the overall emissions profile of FCEVs. Green hydrogen, produced from renewable energy sources through electrolysis, offers the greatest environmental benefits.
The choice between FCEVs and BEVs depends on a variety of factors, including infrastructure availability, cost, driving range requirements, and environmental priorities. Both technologies have a role to play in the transition to a sustainable transportation future.
Maintaining Purity: Fuel Quality and International Standards
Harnessing Hydrogen's Energy: Fuel Cell Technology Explained From production to power: Hydrogen production and storage methods Building a hydrogen economy requires not only efficient fuel cells and robust infrastructure but also, critically, effective methods for producing and storing hydrogen. The source of hydrogen and how it's held until use sig...
Hydrogen fuel quality is paramount for the reliable and efficient operation of hydrogen fuel cell systems. Just as gasoline requires specific octane levels and diesel must meet certain sulfur content limits, hydrogen must adhere to stringent purity standards. This is not merely an academic exercise but a fundamental requirement for ensuring the longevity and performance of fuel cells and protecting the significant investments made in hydrogen technologies.
The Imperative of Hydrogen Purity
The reason purity is so crucial lies in the delicate nature of fuel cell catalysts. These catalysts, often made of platinum or other precious metals, are highly susceptible to poisoning or deactivation by even trace amounts of contaminants.
For instance, sulfur compounds, carbon monoxide, and ammonia can irreversibly bind to the catalytic sites, reducing the fuel cell's ability to facilitate the electrochemical reaction that generates electricity.
This degradation leads to reduced power output, increased fuel consumption, and ultimately, premature failure of the fuel cell stack.
ISO 14687: The Global Benchmark for Hydrogen Quality
To address these concerns, the International Organization for Standardization (ISO) developed ISO 14687, a comprehensive standard that specifies the acceptable limits for various contaminants in hydrogen fuel. This standard acts as a global benchmark, ensuring that hydrogen dispensed at refueling stations and used in industrial applications meets minimum quality requirements.
ISO 14687 outlines permissible levels for various contaminants, including:
- Water: Excessive water content can lead to corrosion and freezing within the fuel cell system.
- Total hydrocarbons: These can form deposits on the catalyst surface, hindering its activity.
- Carbon monoxide: Even small amounts of CO can severely poison platinum catalysts.
- Sulfur compounds: These are notorious catalyst poisons, leading to rapid performance degradation.
- Nitrogen: While generally inert, high concentrations of nitrogen can dilute the hydrogen fuel, reducing its energy density.
- Oxygen: Can contribute to the oxidation of fuel cell components.
Ensuring Compliance and Quality Assurance
Adhering to ISO 14687 requires a rigorous approach to quality control throughout the entire hydrogen supply chain.
This includes:
- Monitoring hydrogen production processes: Implementing real-time monitoring systems to detect and mitigate contamination during hydrogen generation.
- Employing purification technologies: Utilizing advanced purification techniques, such as pressure swing adsorption (PSA) and membrane separation, to remove impurities.
- Conducting regular fuel quality testing: Implementing routine fuel quality checks at production facilities, storage facilities, and refueling stations to ensure compliance with ISO 14687.
The Economic Impact of Fuel Quality
The importance of fuel quality extends beyond technical considerations and impacts the economic viability of hydrogen as a transportation fuel. Poor fuel quality can lead to increased maintenance costs, reduced vehicle range, and premature replacement of fuel cell stacks. These factors erode consumer confidence and hinder the widespread adoption of hydrogen technologies.
By ensuring consistent fuel quality, we can maximize the lifespan and performance of fuel cell systems, reducing operational costs and improving the overall economic competitiveness of hydrogen.
Looking Ahead: Harmonization and Continuous Improvement
As the hydrogen economy continues to evolve, it is essential to promote harmonization of fuel quality standards across different regions and industries. This will facilitate international trade, promote technology transfer, and create a more level playing field for hydrogen stakeholders.
Furthermore, continuous research and development efforts are needed to improve hydrogen purification technologies, develop more robust fuel cell catalysts, and establish more accurate and reliable fuel quality testing methods. By prioritizing fuel quality, we can unlock the full potential of hydrogen as a clean, sustainable, and reliable energy carrier.
The Bottom Line: Economic Considerations of Hydrogen Fuel
Building a hydrogen economy requires not only efficient fuel cells and robust infrastructure but also, critically, effective methods for producing and storing hydrogen affordably. The economic viability of hydrogen, particularly as a transportation fuel, hinges on overcoming significant cost barriers that currently hinder widespread adoption. This section examines the current cost landscape of hydrogen fuel, the key factors influencing these costs, and the pathways toward achieving competitive pricing.
Understanding the Current Cost of Hydrogen
The price consumers pay at the hydrogen refueling station is a critical factor in determining the appeal of Fuel Cell Electric Vehicles (FCEVs). Currently, the cost of hydrogen fuel varies considerably depending on location, production method, and infrastructure maturity.
Several studies and reports indicate that hydrogen fuel can range from $13 to $30 per kilogram at retail stations.
This price range is substantially higher than the equivalent energy cost of gasoline or electricity for Battery Electric Vehicles (BEVs), making it a significant impediment to broader consumer adoption. This high cost necessitates a deeper examination of the factors driving the price and the potential for cost reduction.
Factors Influencing Hydrogen Fuel Cost
Several interconnected elements contribute to the overall cost of hydrogen fuel, each requiring targeted strategies for optimization.
These factors can be broadly categorized into production costs, distribution expenses, and station-related charges.
Production Costs
The method of hydrogen production has a direct impact on its cost. Electrolysis using renewable energy sources ("green hydrogen") is generally more expensive than steam methane reforming (SMR), a process that uses natural gas. SMR is a more established and efficient technology, but it also generates carbon dioxide emissions, unless paired with carbon capture and storage (CCS) technologies.
Distribution and Transportation
Transporting hydrogen from production facilities to refueling stations is another significant cost driver. Hydrogen can be transported via pipelines, trucks, or ships, with each method having its own infrastructure requirements and logistical challenges. The cost of compression, liquefaction, or conversion to other forms (e.g., ammonia) for transport further adds to the overall expense.
Refueling Station Costs
The cost of building and operating hydrogen refueling stations is considerable. This includes the expense of specialized equipment such as compressors, dispensers, and storage tanks, as well as ongoing maintenance and operational costs. Low utilization rates at many existing stations also contribute to higher per-kilogram costs.
Pathways to Cost Reduction
Achieving cost competitiveness with conventional fuels and BEVs requires a multi-pronged approach focused on technological advancements, economies of scale, and policy support.
Advancing Production Technologies
Continued research and development into more efficient and cost-effective hydrogen production methods are crucial. This includes improving the efficiency of electrolyzers, scaling up renewable energy production, and exploring alternative production pathways such as biomass gasification or solar thermochemical processes.
Optimizing Distribution and Infrastructure
Investments in hydrogen pipeline infrastructure can reduce transportation costs in regions with high demand. Developing innovative storage solutions and optimizing logistics can further lower expenses associated with distribution. Public and private partnerships can play a vital role in deploying refueling infrastructure and improving station utilization rates.
Policy and Incentives
Government policies, such as tax credits, subsidies, and mandates, can help to accelerate the adoption of hydrogen technologies and drive down costs. Supporting research and development, incentivizing the deployment of refueling infrastructure, and creating a level playing field for hydrogen in the energy market are essential steps.
The Path Forward
While hydrogen fuel currently faces economic challenges, the long-term potential for cost reduction is significant. As technology advances, infrastructure expands, and economies of scale are realized, hydrogen can become a competitive and sustainable transportation fuel, contributing to a cleaner and more resilient energy future.
The Hydrogen Ecosystem: Key Organizations and Initiatives Shaping the Future
Building a hydrogen economy requires not only efficient fuel cells and robust infrastructure but also, critically, a collaborative ecosystem of organizations pushing the boundaries of innovation and deployment. From industry consortia setting standards to automotive giants investing billions and energy companies retooling their operations, the hydrogen revolution is a collective effort. This section delves into the roles and initiatives of these key players, critically assessing their contributions to a hydrogen-powered future.
Industry Associations: Orchestrating Collaboration
Industry associations play a crucial role in fostering collaboration, setting standards, and advocating for policies that support the hydrogen economy. They provide a platform for stakeholders to share knowledge, address common challenges, and collectively promote the benefits of hydrogen as a clean energy carrier.
Hydrogen Council: A Global Advocate
The Hydrogen Council stands out as a leading global initiative, bringing together CEOs from across the energy, transportation, and industrial sectors. With a membership representing a significant portion of the hydrogen value chain, the Council aims to accelerate the deployment of hydrogen solutions by:
- Advocating for supportive policies and regulations.
- Promoting investment in hydrogen infrastructure.
- Raising awareness of the potential of hydrogen to decarbonize various sectors.
While the Hydrogen Council has undoubtedly played a vital role in raising the profile of hydrogen, it faces scrutiny regarding the diversity of its membership and the potential for greenwashing. Ensuring that all stakeholders, including smaller companies and environmental groups, have a voice is essential for the Council's continued credibility and effectiveness.
Automotive Manufacturers: Driving the Transition
Automotive manufacturers are at the forefront of the hydrogen revolution, investing heavily in the development of fuel cell electric vehicles (FCEVs). By offering viable alternatives to gasoline-powered cars and battery electric vehicles (BEVs), these companies are helping to drive the demand for hydrogen fuel and infrastructure.
Toyota: A Pioneer of Fuel Cell Technology
Toyota has been a long-time proponent of hydrogen technology, with its Mirai FCEV serving as a flagship model for the company's commitment to sustainable transportation.
Toyota's investment in hydrogen extends beyond vehicle production, encompassing research and development in fuel cell technology, hydrogen production, and infrastructure development.
However, the limited availability of hydrogen refueling stations remains a significant challenge for Mirai owners and a barrier to wider adoption of FCEVs.
Hyundai: Expanding the FCEV Ecosystem
Hyundai has also emerged as a major player in the FCEV market, with its Nexo SUV offering a compelling combination of range, performance, and zero-emission operation. Beyond vehicle development, Hyundai is actively investing in hydrogen infrastructure, partnering with governments and other companies to build refueling stations and promote the adoption of hydrogen technology.
Hyundai's efforts extend beyond passenger vehicles, with the company exploring the use of fuel cells in trucks, buses, and other commercial vehicles. This diversified approach to hydrogen adoption positions Hyundai as a key player in the broader hydrogen ecosystem.
Energy Companies: Building the Supply Chain
Energy companies play a critical role in building the hydrogen supply chain, from producing hydrogen to transporting it to refueling stations. These companies are leveraging their expertise in energy infrastructure and distribution to develop the technologies and systems needed to support a hydrogen economy.
Shell: Investing in Refueling Infrastructure
Shell is one of the leading energy companies investing in hydrogen refueling infrastructure, with stations operating in Europe and North America. Shell's involvement in hydrogen extends beyond refueling, with the company exploring opportunities in hydrogen production and transportation.
Shell's commitment to hydrogen reflects a broader strategy of transitioning to a cleaner energy future, but the pace of infrastructure development needs to accelerate to meet the growing demand for hydrogen fuel.
Linde and Air Liquide: Enabling the Hydrogen Value Chain
Linde and Air Liquide are global gas companies with extensive experience in hydrogen production, storage, and distribution. These companies play a critical role in the hydrogen value chain, supplying hydrogen to industrial customers and refueling stations.
Linde and Air Liquide are also investing in new technologies for hydrogen production and storage, helping to drive down the cost of hydrogen and improve its competitiveness with other fuels.
The continued collaboration and innovation of these key organizations will be essential for realizing the full potential of hydrogen as a clean and sustainable energy solution. While challenges remain, their commitment to the hydrogen economy provides a strong foundation for a future powered by this versatile energy carrier.
Under the Hood: Essential Tools, Safety Measures, and Quality Control
The Hydrogen Ecosystem: Key Organizations and Initiatives Shaping the Future Building a hydrogen economy requires not only efficient fuel cells and robust infrastructure but also, critically, a collaborative ecosystem of organizations pushing the boundaries of innovation and deployment. From industry consortia setting standards to automotive giants, the successful integration of hydrogen hinges on a reliable network supported by meticulous safety and quality protocols. Ensuring the safe and reliable operation of hydrogen systems necessitates a deep dive into the essential components of hydrogen infrastructure, emphasizing rigorous safety standards and uncompromising quality control measures.
Essential Components of Hydrogen Infrastructure
The hydrogen infrastructure comprises specialized equipment tailored to the unique properties of this energy carrier. From compressing hydrogen to dispensing it safely into vehicles, each component plays a critical role.
Compressors: The Heart of Hydrogen Delivery
Compressors are vital for increasing the pressure of hydrogen gas, enabling efficient storage and transportation. These devices must be designed to handle hydrogen’s low density and prevent leaks.
Different compressor technologies are employed, including mechanical, electrochemical, and thermal compressors. Each type presents trade-offs in efficiency, cost, and scalability. Selecting the right compressor for a specific application is essential for operational success.
Dispensers: Precise and Safe Refueling
Hydrogen dispensers are responsible for safely and accurately delivering hydrogen fuel to vehicles. These sophisticated units meter the fuel flow and monitor temperature and pressure to ensure optimal refueling conditions.
Safety features are paramount in dispenser design, including leak detection systems, emergency shut-off valves, and grounding mechanisms to prevent static electricity buildup. Standardization of connectors and protocols is also crucial for seamless operation across different vehicle types.
Storage Tanks: Safe Containment of Hydrogen
Hydrogen storage tanks are engineered to contain hydrogen gas or liquid under high pressure. These tanks must meet stringent safety standards to prevent leaks and ruptures.
Materials used in their construction, such as carbon fiber composites and high-strength steel, are carefully selected for their durability and resistance to hydrogen embrittlement. Regular inspections and maintenance are critical to ensure long-term integrity.
Navigating Safety Standards in Hydrogen Infrastructure
The inherent flammability of hydrogen necessitates stringent safety standards to mitigate risks. These standards govern the design, construction, and operation of hydrogen systems, ensuring the safety of personnel and the public.
NFPA 2 Hydrogen Technologies Code: A U.S. Standard
The NFPA 2 Hydrogen Technologies Code provides comprehensive safety requirements for hydrogen production, storage, distribution, and utilization in the United States. It covers a wide range of topics, including:
- Ventilation requirements
- Electrical safety
- Fire protection
- Emergency response procedures
Adherence to NFPA 2 is essential for minimizing fire and explosion hazards associated with hydrogen.
CSA Group Standards: Canadian Safety Benchmarks
The CSA Group develops and publishes safety standards for hydrogen infrastructure in Canada. These standards address various aspects of hydrogen safety, including:
- Fuel cell systems
- Hydrogen storage
- Hydrogen dispensing stations
Compliance with CSA Group standards is mandatory for ensuring the safe deployment of hydrogen technologies in Canada.
Quality Measurement: Ensuring Fuel Integrity
Maintaining hydrogen fuel quality is crucial for the optimal performance and longevity of fuel cell systems. Impurities in the fuel can degrade fuel cell performance and damage critical components.
Hydrogen Analyzers: Guardians of Purity
Hydrogen analyzers are used to monitor the purity of hydrogen fuel, detecting and quantifying contaminants such as water, carbon monoxide, and sulfur compounds. These analyzers play a vital role in ensuring that hydrogen fuel meets stringent quality standards, such as ISO 14687.
Real-time monitoring and analysis allows for swift identification and mitigation of contamination issues, preserving the integrity and reliability of hydrogen fuel cell systems.
Looking Ahead: Challenges, Opportunities, and the Future of Hydrogen
The Hydrogen Ecosystem: Key Organizations and Initiatives Shaping the Future Under the Hood: Essential Tools, Safety Measures, and Quality Control Building a hydrogen economy requires not only efficient fuel cells and robust infrastructure but also a clear vision for the future. While the potential of hydrogen as a clean energy carrier is undeniable, significant hurdles remain before it can achieve widespread adoption and truly transform the energy landscape. This section delves into these challenges, explores emerging technological advancements, and provides a perspective on the long-term potential of hydrogen.
Addressing Current Obstacles
The widespread adoption of hydrogen faces significant roadblocks, primarily related to infrastructure and cost. Overcoming these obstacles is crucial for realizing hydrogen's potential as a viable alternative to fossil fuels.
Infrastructure Deficiencies: The current hydrogen refueling infrastructure is severely limited, creating a "chicken and egg" scenario. Without sufficient refueling stations, consumer adoption of Fuel Cell Electric Vehicles (FCEVs) will remain slow. Investment in infrastructure development is paramount, requiring coordinated efforts from governments, industry, and research institutions.
High Production and Distribution Costs: The cost of producing and distributing hydrogen remains a significant barrier. Current hydrogen production methods, particularly those relying on natural gas, can be energy-intensive and produce greenhouse gas emissions. Reducing the cost of hydrogen production, especially through renewable energy-powered electrolysis, is essential for making hydrogen economically competitive.
Public Perception and Acceptance: Addressing concerns about safety and dispelling misconceptions surrounding hydrogen are crucial for gaining public trust and acceptance. Open communication and educational initiatives can help build confidence in hydrogen technologies.
Future Technologies and Research
Continuous innovation and technological advancements are vital for overcoming the challenges associated with hydrogen and unlocking its full potential. Ongoing research efforts are focused on improving hydrogen production methods, storage solutions, and fuel cell technologies.
Advancements in Hydrogen Production
Green Hydrogen Production: Electrolysis powered by renewable energy sources (solar, wind, hydro) offers a pathway to produce green hydrogen, a truly sustainable and carbon-neutral fuel. Further advancements in electrolysis technologies, such as solid oxide electrolysis cells (SOECs), hold the promise of increased efficiency and reduced costs.
Bio-Hydrogen Production: Exploring biological methods of hydrogen production, such as using algae or bacteria, offers a potentially sustainable and environmentally friendly alternative to traditional methods.
Innovations in Hydrogen Storage
Solid-State Storage: Solid-state hydrogen storage, using materials that absorb hydrogen, offers a safer and more compact alternative to compressed or liquid hydrogen storage. Research efforts are focused on developing materials with high hydrogen storage capacity, fast absorption/desorption kinetics, and long-term stability.
Advanced Compression Techniques: Developing more efficient and cost-effective compression techniques can reduce the energy consumption and cost associated with compressing hydrogen for storage and transportation.
Enhancements in Fuel Cell Technology
Increased Efficiency and Durability: Improving the efficiency and durability of fuel cells is critical for maximizing the performance and lifespan of FCEVs and other applications. Research is focused on developing new materials and designs that can withstand harsh operating conditions and extend fuel cell lifespan.
Reduced Platinum Content: Platinum is a key component of PEM fuel cells, but its high cost is a barrier to widespread adoption. Reducing the platinum content or developing alternative catalyst materials can significantly lower the cost of fuel cells.
Video: Hydrogen Charge: Fuel Cell EV Charging Guide
FAQ: Hydrogen Charge and Fuel Cell EVs
What is a Fuel Cell Electric Vehicle (FCEV)?
A Fuel Cell Electric Vehicle (FCEV) is an electric vehicle that uses a fuel cell powered by hydrogen to generate electricity, rather than relying solely on a battery. This electricity powers the car's electric motor. The only emission from an FCEV is water vapor.
How does hydrogen charge work for FCEVs?
Hydrogen charge, also known as hydrogen refueling, involves filling the vehicle's hydrogen storage tank with compressed hydrogen gas. Specialized hydrogen stations use pumps and nozzles, similar to gasoline stations, to safely and quickly replenish the hydrogen supply.
How long does it take to hydrogen charge an FCEV?
Hydrogen refueling is typically much faster than charging a battery electric vehicle (BEV). Filling an FCEV's hydrogen tank usually takes only 3-5 minutes, providing a driving range comparable to gasoline vehicles.
Where can I find hydrogen charging stations?
Hydrogen charging stations, while still limited in number compared to gasoline stations or electric vehicle charging stations, are becoming more available. You can find them using online mapping tools and mobile apps that specialize in locating alternative fuel stations, including those offering hydrogen charge.
So, there you have it! Hopefully, this guide clarifies the ins and outs of hydrogen charge and how it works in the fuel cell EV world. It might seem complex at first, but understanding the process can really empower you to make informed decisions about your future vehicle choices. Keep exploring and stay charged up about hydrogen!