Undrained Shear Strength: Soil Stability Guide
For geotechnical engineers, understanding the undrained shear strength of soil is critical for evaluating soil stability, especially in conditions where rapid loading prevents dissipation of pore water pressure. The American Society for Testing and Materials (ASTM) provides standardized methods for measuring this key parameter, ensuring consistency across different projects. These methods include laboratory tests such as the triaxial test and vane shear test, which help determine the cohesion of the soil under undrained conditions. The late Karl Terzaghi’s principles of effective stress and consolidation are fundamental to interpreting undrained shear strength, guiding engineers in predicting the behavior of saturated soils in various construction and environmental applications.
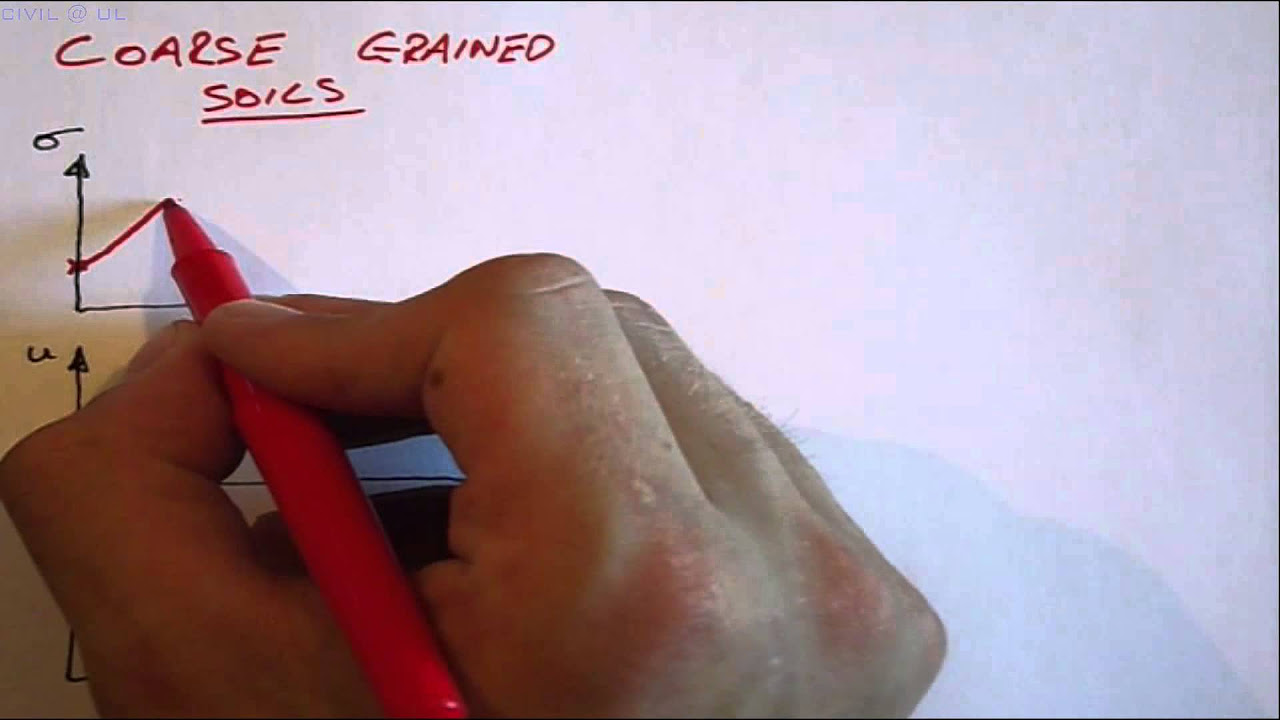
Image taken from the YouTube channel UL Civil Eng , from the video titled Drained and Undrained Soil Shear Strength .
Shear strength is a fundamental concept in geotechnical engineering, representing a soil's ability to resist deformation and failure under applied stress. It is the internal resistance of a soil mass to shearing stresses, essentially dictating how much load a soil can bear before it yields or collapses.
Defining Shear Strength and Its Significance
In geotechnical design, shear strength is a critical parameter. This strength is used to assess the stability of slopes, the bearing capacity of foundations, and the earth pressure against retaining walls.
Inadequate shear strength can lead to catastrophic failures, resulting in structural damage, economic losses, and even loss of life. Therefore, accurate determination and appropriate application of shear strength values are paramount.
Understanding Undrained Shear Strength
Undrained shear strength (Su) specifically refers to the shear strength of a saturated soil when loaded rapidly. The load is applied so quickly that there is insufficient time for water to drain from the soil pores. This is particularly relevant in saturated cohesive soils like clays.
Under undrained conditions, the total stress applied is borne by both the soil skeleton and the pore water. This causes an increase in pore water pressure and reduces the effective stress within the soil. As a result, the soil's resistance to shear is governed by the initial effective stress state and the soil's inherent properties.
The undrained shear strength is a critical design parameter in saturated soils under short-term loading conditions.
Critical Scenarios Requiring Undrained Shear Strength Analysis
Several common geotechnical scenarios require careful consideration of undrained shear strength:
- Rapid Loading: Situations involving rapidly applied loads, such as the construction of embankments or the sudden drawdown of water levels in reservoirs, necessitate undrained shear strength analysis. The sudden load application does not allow for water drainage, leading to changes in pore pressure and affecting stability.
- Construction on Soft Clay: Building foundations, roads, or other structures on soft clay deposits poses significant challenges. Soft clays typically have low shear strength, and any rapid construction activity can induce undrained conditions, potentially causing instability and settlement issues.
- Excavations: Deep excavations often require temporary support systems to prevent collapse. Analyzing the undrained shear strength of the surrounding soil is crucial for designing stable excavation support and ensuring worker safety.
- Rapid Slope Loading: Slope stability can be affected greatly under rapid loading.
The Role of Effective Stress
Effective stress is the stress carried by the soil skeleton, calculated by subtracting pore water pressure from the total stress. Understanding effective stress is crucial because it governs the soil's strength and deformation characteristics.
Under drained conditions, pore water pressure dissipates, and the effective stress increases, leading to a higher shear strength.
However, under undrained conditions, the effective stress remains relatively constant or decreases due to the inability of pore water to escape. The effective stress principle underscores the importance of drainage conditions in determining the appropriate shear strength parameter to use in geotechnical design.
Fundamental Concepts: Effective Stress and Drainage Conditions
Shear strength is a fundamental concept in geotechnical engineering, representing a soil's ability to resist deformation and failure under applied stress. It is the internal resistance of a soil mass to shearing stresses, essentially dictating how much load a soil can bear before it yields or collapses. Understanding the principles that govern soil behavior under different loading and drainage conditions is paramount for ensuring the stability and safety of geotechnical structures. In this section, we will delve into the core concepts of effective stress and drainage conditions, which are foundational to comprehending undrained shear strength.
The Effective Stress Principle
The effective stress principle, first articulated by Karl Terzaghi, is a cornerstone of soil mechanics. It revolutionized our understanding of how soils respond to applied loads.
Defining Total Stress, Pore Water Pressure, and Effective Stress
When a soil mass is subjected to external forces, the total stress (σ) represents the entire load acting on a plane within the soil. This total stress is carried by both the soil solids (the mineral grains) and the pore water within the voids between the grains.
Pore water pressure (u) is the pressure exerted by the water filling these voids. It acts equally in all directions.
The effective stress (σ') is the portion of the total stress that is actually carried by the soil skeleton. It represents the intergranular stress between soil particles. This is the stress that controls the soil's mechanical behavior, including its strength and compressibility.
The Effective Stress Formula
The relationship between these three stresses is expressed by the formula:
σ' = σ - u
Where:
- σ' = Effective Stress
- σ = Total Stress
- u = Pore Water Pressure
This seemingly simple equation has profound implications. It emphasizes that the strength and deformation characteristics of a soil are primarily governed by the effective stress, not the total stress.
Significance of Effective Stress
The effective stress principle dictates that changes in pore water pressure directly influence the effective stress, and consequently, the soil's behavior. For instance, an increase in pore water pressure reduces the effective stress, potentially leading to a decrease in shear strength and an increased risk of failure. This principle underpins our ability to analyze and predict soil behavior under various loading conditions.
Total Stress Analysis (TSA) vs. Effective Stress Analysis (ESA)
Geotechnical engineers employ two primary approaches to analyze soil behavior: Total Stress Analysis (TSA) and Effective Stress Analysis (ESA). The selection of an appropriate method depends on the drainage conditions and the nature of the problem.
When to Use TSA vs. ESA
TSA is typically used for short-term stability analyses in situations where drainage is impeded, such as during rapid loading of saturated clay soils. This is because the pore water pressure changes induced by the loading do not have sufficient time to dissipate.
ESA, on the other hand, is applied to long-term stability analyses where drainage is allowed to occur, and pore water pressures have reached equilibrium. This approach considers the effective stresses acting within the soil mass.
Relationship between TSA and Undrained Shear Strength
In TSA, the undrained shear strength (sᵤ) is used as the failure criterion. The analysis assumes that the soil fails when the shear stress reaches sᵤ, without explicitly considering the pore water pressure. The undrained shear strength (sᵤ) is a soil parameter obtained from laboratory or in-situ tests conducted under undrained conditions. This parameter reflects the soil's resistance to shear when drainage is prevented.
Relationship between ESA, Effective Stress, and Drained Shear Strength
ESA uses the drained shear strength parameters, effective cohesion (c') and effective friction angle (φ'), to define the soil's shear strength. The Mohr-Coulomb failure criterion is then applied, using the calculated effective stresses, to determine if the soil will fail. This approach explicitly accounts for the influence of pore water pressure and the resulting effective stresses on the soil's strength. The drained shear strength parameters (c' and φ') represent the soil's resistance to shear when drainage is allowed, and pore water pressures have reached equilibrium.
Importance of Drainage Conditions
Drainage conditions play a pivotal role in determining the shear strength of soils. Soils can exhibit significantly different strengths depending on whether they are allowed to drain freely or if drainage is prevented.
In drained conditions, pore water pressures dissipate as quickly as they are generated. This allows the soil to consolidate and increase its effective stress, resulting in higher shear strength.
In undrained conditions, pore water pressures cannot dissipate, leading to changes in effective stress that can either increase or decrease the soil's resistance to shear. This underscores the importance of understanding and appropriately modeling drainage conditions when assessing soil stability.
Key Figures in Undrained Shear Strength Development
Shear strength is a fundamental concept in geotechnical engineering, representing a soil's ability to resist deformation and failure under applied stress. It is the internal resistance of a soil mass to shearing stresses, essentially dictating how much load a soil can bear before it yields. Before exploring the practical implications and testing methods, it's crucial to acknowledge the individuals who laid the groundwork for our current understanding of undrained shear strength. Their research and insights have shaped modern geotechnical engineering practice, and understanding their contributions provides valuable context for appreciating the complexities of soil behavior.
Karl Terzaghi: The Father of Soil Mechanics
Karl Terzaghi is widely regarded as the father of soil mechanics, and his development of the principle of effective stress is foundational to understanding undrained shear strength.
The effective stress principle, expressed as σ' = σ - u (where σ' is effective stress, σ is total stress, and u is pore water pressure), revolutionized the way engineers analyze soil behavior.
Terzaghi recognized that the strength and deformation characteristics of soil are primarily controlled by the effective stress, which is the stress carried by the soil skeleton.
This principle is especially critical in understanding undrained conditions, where changes in total stress are initially borne by the pore water, leading to changes in pore water pressure and, consequently, affecting the effective stress and undrained shear strength. His work provided the framework for differentiating between total stress analysis and effective stress analysis.
Arthur Casagrande: Soil Classification and Testing
Arthur Casagrande made significant contributions to soil mechanics through his work on soil classification systems and soil testing methods.
His development of the Atterberg limits tests, specifically the liquid limit and plastic limit, provides a means to classify fine-grained soils and estimate their behavior under different moisture conditions.
These tests, when combined, give the Plasticity Index (PI), a crucial parameter for estimating the undrained shear strength of cohesive soils.
Casagrande's contributions extended beyond soil classification to include the development and refinement of laboratory testing techniques. He emphasized the importance of carefully controlled laboratory experiments to accurately determine soil properties, including shear strength parameters.
W. Skempton: Pore Pressure Parameters
A.W. Skempton is renowned for his research on pore pressure parameters, specifically the A and B parameters, which are crucial for understanding the behavior of soils under undrained conditions.
Skempton's pore pressure parameters quantify how changes in applied stress induce changes in pore water pressure.
The B parameter relates the change in pore water pressure to a change in the cell pressure during a triaxial test, while the A parameter relates the change in pore water pressure to a change in deviator stress.
These parameters are essential for predicting the undrained shear strength of soils, as they allow engineers to estimate the pore water pressure response to loading, and consequently, the effective stress changes and the available shear strength.
His work emphasized the importance of considering pore water pressure in analyzing the stability of slopes and foundations, especially in situations involving rapid loading or undrained conditions.
Lambe and Whitman: Bridging Theory and Practice
T. William Lambe and Robert V. Whitman, through their influential textbook "Soil Mechanics," made significant contributions to solidifying soil mechanics theories and their practical applications.
They emphasized the importance of understanding the fundamental principles of soil behavior and applying them to solve real-world engineering problems.
Their work helped bridge the gap between theoretical concepts and practical design considerations, making soil mechanics more accessible to practicing engineers. They are noted to have presented soil mechanics in the context of stress path method, which allowed geotechnical engineers to predict soil behavior under various loading conditions.
Soil Properties Influencing Undrained Shear Strength
[Key Figures in Undrained Shear Strength Development Shear strength is a fundamental concept in geotechnical engineering, representing a soil's ability to resist deformation and failure under applied stress. It is the internal resistance of a soil mass to shearing stresses, essentially dictating how much load a soil can bear before it yields. Before...]
Understanding the undrained shear strength of soils is crucial for a wide range of geotechnical applications. However, this strength is not a fixed property; it is significantly influenced by several intrinsic soil characteristics. Let's delve into some of the most influential soil properties.
Plasticity Index (PI) and Undrained Shear Strength
The Plasticity Index (PI) is a fundamental indicator of the plasticity of fine-grained soils. It represents the range of water content over which the soil exhibits plastic behavior. It's calculated as the difference between the Liquid Limit (LL) and the Plastic Limit (PL) of the soil.
Generally, there is a positive correlation between PI and undrained shear strength in cohesive soils. Soils with higher PI values tend to have a greater capacity to resist deformation under undrained conditions.
This is because higher PI values indicate a greater proportion of clay minerals. These minerals contribute to increased cohesion within the soil matrix.
Estimating Undrained Shear Strength Using PI
While not a direct measurement, PI can provide a useful estimate of undrained shear strength, particularly for preliminary assessments. Empirical correlations exist that relate PI to undrained shear strength. These correlations should be used cautiously and validated with site-specific testing. The applicability depends heavily on soil mineralogy and other site-specific factors.
Overconsolidation Ratio (OCR) and Its Effects
The Overconsolidation Ratio (OCR) is a measure of the stress history of a soil. It is defined as the ratio of the maximum past effective vertical stress to the current effective vertical stress. OCR significantly impacts the soil's behavior, including its undrained shear strength.
The Influence of OCR on Undrained Shear Strength
Overconsolidated soils (OCR > 1) typically exhibit higher undrained shear strength compared to normally consolidated soils (OCR = 1) at the same effective stress. This is because the soil structure in overconsolidated soils is more compact and interlocked.
Stress-Strain Behavior and OCR
The stress-strain behavior of soils is also greatly influenced by OCR. Overconsolidated soils tend to exhibit a more brittle failure mode, with a more pronounced peak strength and a more rapid decrease in strength after peak. This behavior must be considered in design.
Sensitivity of Clay Soils
The sensitivity of a clay refers to the reduction in its undrained shear strength upon remolding at constant water content. Some clays exhibit a significant loss of strength when disturbed, while others are relatively unaffected.
Impact of Remolding
Remolding destroys the original soil structure. This destruction reduces interparticle bonding, resulting in a weaker soil.
Implications for Design
Highly sensitive clays pose challenges in geotechnical design. Large deformations or failures can occur if the soil is disturbed during construction. Geotechnical engineers must carefully consider the sensitivity of the clay when designing foundations, slopes, and other earthworks.
Anisotropy and Directional Dependence
Anisotropy refers to the directional dependence of soil properties. In other words, the soil's strength and stiffness may vary depending on the direction in which they are measured.
Undrained Shear Strength in Different Directions
The undrained shear strength of a soil can be significantly different in the vertical and horizontal directions, or any other direction. This is often due to the depositional history of the soil. The orientation of clay particles and the presence of bedding planes can lead to anisotropic behavior.
Considering Anisotropy in Design
Ignoring anisotropy can lead to inaccurate stability analyses and potentially unsafe designs. Geotechnical investigations should include tests that capture the anisotropic nature of the soil. Sophisticated numerical models can incorporate anisotropic soil parameters.
In conclusion, a thorough understanding of these key soil properties is essential for accurately assessing and predicting undrained shear strength, leading to safer and more reliable geotechnical designs.
Laboratory and In-Situ Testing Methods for Undrained Shear Strength
Shear strength is a fundamental concept in geotechnical engineering, representing a soil's ability to resist deformation and failure under applied stress. It is the internal resistance of a soil mass to shearing stresses, essentially dictating the stability of earth structures and foundations. Different testing methodologies have been developed to accurately measure and evaluate undrained shear strength. These methods each possess unique advantages and are applicable under specific conditions, requiring a thorough understanding of their underlying principles.
Triaxial Test
The triaxial test is a versatile and widely used laboratory method for determining the shear strength parameters of soil. This test involves subjecting a cylindrical soil specimen to a confining pressure and then applying an axial load until failure.
Types of Triaxial Tests
There are three main types of triaxial tests: Consolidated Drained (CD), Consolidated Undrained (CU), and Unconsolidated Undrained (UU). Each test differs in terms of the drainage conditions allowed during the consolidation and shearing phases.
-
Consolidated Drained (CD) Test: Allows for complete drainage during both consolidation and shearing. This is suitable for long-term stability analyses, but not for undrained conditions.
-
Consolidated Undrained (CU) Test: Allows drainage during consolidation but prevents drainage during shearing. Pore water pressure is typically measured.
-
Unconsolidated Undrained (UU) Test: No drainage is allowed during either the consolidation or shearing phases, simulating rapid loading conditions.
Determining Undrained Shear Strength with the UU Test
The UU test, also known as the Q test, is specifically designed for determining the undrained shear strength of cohesive soils. This test is performed quickly, without allowing drainage, simulating conditions where pore water pressures cannot dissipate. The resulting shear strength is referred to as the undrained shear strength, denoted as cu.
Unconfined Compression Test (UC)
The Unconfined Compression (UC) test is a simplified version of the triaxial test, performed without any confining pressure. It is one of the most economical and quickest methods for estimating undrained shear strength.
Procedure for the UC Test
In this test, a cylindrical soil specimen is subjected to an axial load until failure. The unconfined compressive strength (qu) is defined as the axial stress at failure. The undrained shear strength, cu, is then calculated as half of the unconfined compressive strength:
cu = qu / 2
Limitations of the UC Test
Despite its simplicity, the UC test has limitations.
It is only applicable to cohesive soils that can stand unsupported.
It is unreliable for fissured or very soft clays because they tend to crumble or fail prematurely due to the absence of confining pressure.
Vane Shear Test (VST)
The Vane Shear Test (VST) is an in-situ test used to determine the undrained shear strength of soft, saturated clays. The VST involves inserting a four-bladed vane into the soil and rotating it at a constant rate.
The torque required to shear the soil is measured, and the undrained shear strength is calculated based on the vane geometry and the measured torque.
The VST is particularly useful for sensitive clays, where sample disturbance during laboratory testing can significantly alter the measured strength.
Cone Penetration Test (CPT)
The Cone Penetration Test (CPT) is an in-situ test that involves pushing a cone-shaped penetrometer into the soil at a constant rate. Measurements of cone resistance (qc) and sleeve friction (fs) are recorded continuously.
CPT Correlations with Undrained Shear Strength
The cone resistance (qc) is empirically correlated with the undrained shear strength (cu) using the following general equation:
cu = (qc - σv0) / Nk
Where:
- σv0 is the total overburden stress.
- Nk is an empirical cone factor that depends on soil type, plasticity, and overconsolidation ratio (OCR). The value of Nk typically ranges from 10 to 20.
Standard Penetration Test (SPT)
The Standard Penetration Test (SPT) is a widely used in-situ test that involves driving a thick-walled sampler into the soil using a hammer. The number of blows required to drive the sampler a specific distance (usually 300 mm or 12 inches) is recorded as the N-value.
Indirect Estimation of Undrained Shear Strength Using SPT
Although the SPT is primarily used for cohesionless soils, it can provide indirect estimates of undrained shear strength for cohesive soils. Empirical correlations exist between the N-value and cu, but these correlations are generally less reliable than those obtained from CPT or laboratory tests.
A common correlation is:
cu = K N*
Where K is an empirical factor that varies depending on soil type and plasticity. This correlation should be used with caution and validated with other test data whenever possible.
Analysis and Design Considerations Using Undrained Shear Strength
[Laboratory and In-Situ Testing Methods for Undrained Shear Strength Shear strength is a fundamental concept in geotechnical engineering, representing a soil's ability to resist deformation and failure under applied stress. It is the internal resistance of a soil mass to shearing stresses, essentially dictating the stability of earth structures and...]
The determination of undrained shear strength is crucial; however, it is only the first step. The real value lies in its application to geotechnical analysis and design. This section examines how undrained shear strength is integrated into various analytical methods and software platforms, bridging the gap between theoretical values and practical engineering solutions.
Limit Equilibrium Methods and Undrained Shear Strength
Limit equilibrium methods have long been a cornerstone of slope stability analysis. These methods, such as Fellenius, Bishop's Simplified, and Janbu's method, provide a relatively straightforward approach to assessing the factor of safety of slopes. The undrained shear strength, denoted as cu, plays a central role in these calculations, particularly in total stress analyses for short-term stability assessments.
The factor of safety (FS) is calculated as the ratio of resisting forces to driving forces along a potential failure surface. When dealing with undrained conditions, the undrained shear strength is directly used to quantify the resisting forces.
For instance, in the Fellenius method (also known as the Swedish circle method), the undrained shear strength is assumed to be constant along the failure surface, simplifying the calculations considerably. The stability is evaluated by summing up the moments of the driving and resisting forces about the center of the assumed circular slip surface.
Bishop's Simplified method, while more complex, still relies on cu for undrained conditions, but it also considers the effect of pore water pressure on the effective normal stress along the failure surface. This iterative method provides a more refined estimation of the factor of safety.
In each of these methods, the accuracy of the undrained shear strength value is paramount. An overestimation can lead to unsafe designs, while an underestimation can result in overly conservative and costly solutions.
Finite Element Analysis (FEA) and Advanced Soil Modeling
Finite Element Analysis (FEA) offers a more sophisticated approach to slope stability and geotechnical design. Unlike limit equilibrium methods, FEA can model complex soil behavior, including non-linearity, anisotropy, and stress-dependent properties.
When using FEA, the undrained shear strength can be incorporated through various constitutive models, such as the Mohr-Coulomb model (in a total stress analysis approach). These models allow engineers to simulate the stress-strain response of soil under undrained conditions, providing a more realistic representation of soil behavior.
FEA enables the analysis of complex geometries, loading conditions, and soil profiles. It can predict deformations, stresses, and pore water pressures within the soil mass, offering insights beyond the capabilities of limit equilibrium methods. Furthermore, FEA can simulate construction sequences, allowing engineers to assess the stability of slopes during different stages of construction.
The effectiveness of FEA, however, hinges on the accuracy of the input parameters, including the undrained shear strength. Careful calibration and validation of the FEA model with field data or laboratory results are essential to ensure reliable predictions.
Slope Stability Software and Practical Implementation
Numerous software tools are available to facilitate slope stability analysis, many of which incorporate both limit equilibrium and FEA capabilities. Programs like GeoStudio (Slope/W), Slide, and Plaxis are widely used in the geotechnical engineering profession.
These software packages allow engineers to define soil properties, including undrained shear strength, and perform stability analyses using various methods. They often include features for generating slip surfaces, calculating factors of safety, and visualizing results.
GeoStudio, for example, allows the user to define different material models, including those suitable for undrained conditions, and perform both limit equilibrium and finite element analyses within the same environment.
Slide offers a range of limit equilibrium methods and provides tools for sensitivity analysis, allowing engineers to assess the impact of variations in soil parameters on the factor of safety.
Plaxis, a finite element software, is particularly well-suited for complex geotechnical problems, including slope stability analysis, and allows for advanced constitutive models.
These software tools streamline the analysis process and provide valuable insights into slope stability. However, it's crucial to remember that software is merely a tool. Engineers must possess a thorough understanding of soil mechanics principles and the limitations of the software to interpret the results correctly and make informed design decisions. The user should never blindly trust software-generated outputs without proper verification and validation.
Practical Applications of Undrained Shear Strength
Analysis and Design Considerations Using Undrained Shear Strength Laboratory and In-Situ Testing Methods for Undrained Shear Strength
Shear strength is a fundamental concept in geotechnical engineering, representing a soil's ability to resist deformation and failure under applied stress. It is the internal resistance of a soil mass to shearing str... Understanding undrained shear strength is paramount in various real-world geotechnical applications, especially where soils are saturated and subjected to rapid loading or undrained conditions. This section illustrates how the proper assessment and application of undrained shear strength is essential for ensuring the safety, stability, and long-term performance of diverse engineering projects.
Construction Site Stability
Construction sites often encounter soft soil conditions, particularly during excavation and building construction. Undrained shear strength is crucial for maintaining stability. Excavations in clayey soils can lead to slope failures if the soil's undrained shear strength is insufficient to support the newly exposed faces. Geotechnical engineers use undrained shear strength values to design appropriate shoring systems, such as sheet piles or soil nailing, to prevent collapses and ensure worker safety.
The stability of temporary slopes and embankments during construction is directly related to the undrained shear strength of the soil. Accurate assessment allows for the determination of safe slope angles, reducing the risk of landslides and ensuring the integrity of the construction site.
Slope and Embankment Stability
Natural slopes and engineered embankments must maintain long-term stability to prevent landslides and other forms of soil failure. The undrained shear strength is a critical parameter in slope stability analysis, especially for short-term conditions where rapid loading or changes in water levels occur.
Analyzing slope stability involves assessing the balance between driving forces (e.g., gravity) and resisting forces (e.g., soil shear strength). Geotechnical engineers use various methods, such as limit equilibrium or finite element analysis, incorporating undrained shear strength parameters to calculate the factor of safety.
The factor of safety indicates the margin of stability: a higher factor indicates a more stable slope. Understanding and applying undrained shear strength allows for informed decisions regarding slope reinforcement, drainage improvements, or slope flattening to enhance stability.
Dam Foundation Integrity
Dam foundations must withstand significant loads from the dam structure and the impounded water. The undrained shear strength of the foundation soils is particularly critical under rapid drawdown conditions, where the water level behind the dam decreases quickly.
Rapid drawdown reduces the support provided by the water, increasing the effective stress on the soil. This can lead to a decrease in stability if the undrained shear strength is not sufficient to resist the increased stress. Geotechnical engineers perform detailed analyses to ensure the dam foundation can withstand rapid drawdown scenarios and prevent catastrophic failures.
Techniques such as soil improvement (e.g., consolidation, grouting) may be employed to increase the undrained shear strength of the foundation soils, further enhancing the stability of the dam.
Roadway and Railway Foundations
The design of stable foundations for roadways and railways requires careful consideration of the undrained shear strength of the underlying soils. Transportation infrastructure is subjected to repetitive loading from traffic, which can cause settlement and instability if the soil is not adequately strong.
Soft soils with low undrained shear strength can undergo significant deformation under traffic loads. Geotechnical engineers assess the soil properties and design appropriate foundation treatments, such as soil stabilization or the use of geosynthetics, to improve the soil's bearing capacity and reduce settlement.
Proper assessment and mitigation strategies are essential for ensuring the long-term performance and safety of roadways and railways.
Challenges in Coastal Engineering
Coastal areas often present unique geotechnical challenges due to the presence of soft, saturated clay deposits. These soils typically have low undrained shear strength and high compressibility, making them difficult to build on.
Coastal engineering projects, such as port facilities, seawalls, and artificial islands, require careful evaluation of the undrained shear strength of the marine clays. Geotechnical engineers must consider the effects of tidal fluctuations, wave action, and potential erosion on the stability of coastal structures.
Techniques such as soil improvement methods (e.g., vertical drains, ground improvement) may be used to enhance the undrained shear strength and reduce the compressibility of the marine clays, enabling the construction of stable and durable coastal infrastructure.
Video: Undrained Shear Strength: Soil Stability Guide
FAQs: Undrained Shear Strength: Soil Stability Guide
What does "undrained" mean in the context of undrained shear strength?
"Undrained" refers to conditions where water cannot escape from the soil during loading. This typically happens during rapid loading of saturated clay soils. Consequently, pore water pressure increases, and the soil strength is determined by the undrained shear strength.
Why is undrained shear strength important for soil stability?
Undrained shear strength is crucial because it represents the soil's resistance to failure under short-term, critical loading scenarios, such as during construction. Knowing the undrained shear strength helps engineers design safe and stable foundations, slopes, and excavations.
How is undrained shear strength typically measured?
Common laboratory tests include the unconfined compression test (UC) and the unconsolidated-undrained triaxial test (UU). Field tests, like the vane shear test, also provide valuable data. These tests aim to determine the undrained shear strength of cohesive soils.
What types of soils are most affected by undrained conditions?
Fine-grained soils, particularly saturated clays and silts, are most susceptible to undrained conditions. These soils have low permeability, preventing water drainage during rapid loading, making the undrained shear strength a primary factor in their stability.
So, there you have it! Understanding undrained shear strength is a cornerstone of ensuring stability in your soil mechanics projects. Hopefully, this guide has given you a solid foundation to build upon. Now go forth and confidently tackle those challenging soil stability issues!