What is Pyrolysis in Fire? A Comprehensive Guide
Pyrolysis, a critical thermal decomposition process, fundamentally alters the chemical composition of organic materials when subjected to elevated temperatures in the absence of sufficient oxygen. The National Fire Protection Association (NFPA) recognizes pyrolysis as a key stage in the combustion process, wherein solid fuels break down into volatile gases. These gases subsequently mix with oxygen and ignite, leading to flame production, a phenomenon extensively studied at institutions such as the Fire Protection Research Foundation. Understanding what is pyrolysis in fire is crucial for fire investigators, as it allows them to accurately determine the origin and cause of fires by analyzing the char patterns and residue left behind, which are often indicative of the temperatures achieved and the materials involved. Advanced analytical techniques, like Gas Chromatography-Mass Spectrometry (GC-MS), are often employed to identify specific pyrolytic products, thereby providing detailed insights into the nature and progression of the fire.
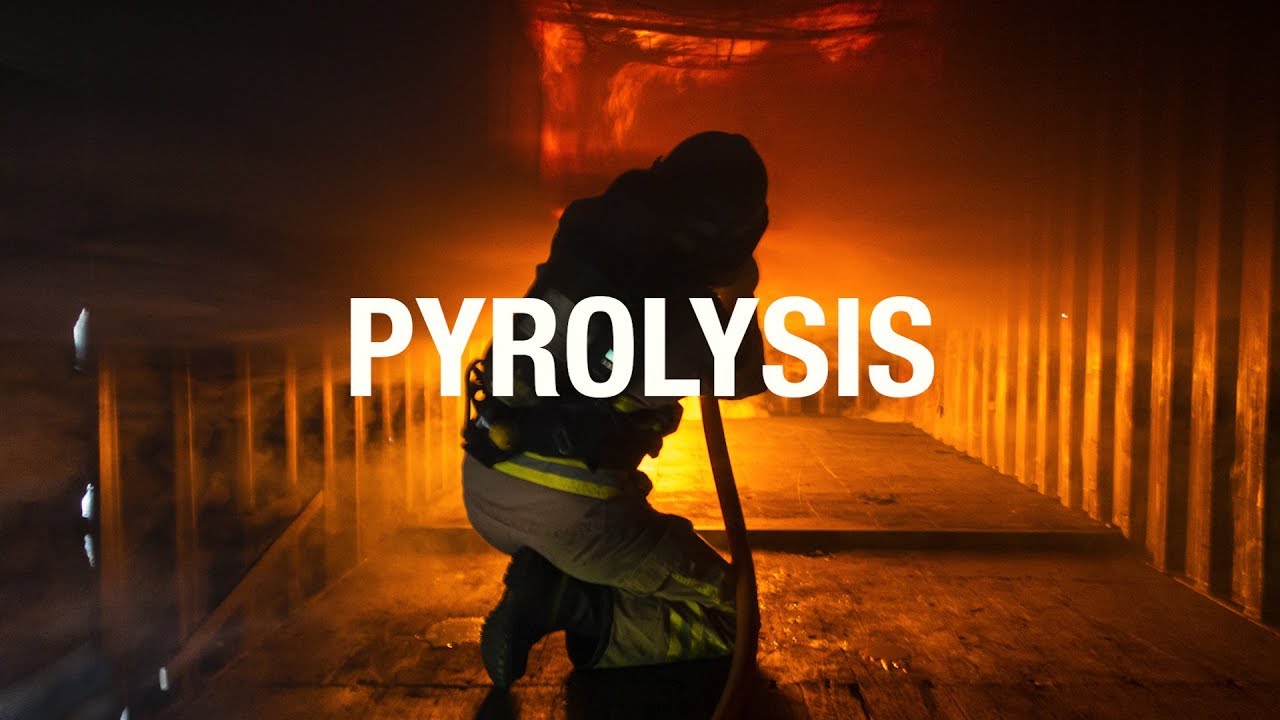
Image taken from the YouTube channel Matt Davis , from the video titled Pyrolysis - Turning solid fuels to smoke - Episode 2 .
Pyrolysis: The Unseen Foundation of Fire
Pyrolysis, at its core, is the thermal decomposition of organic materials.
This process occurs when a substance is subjected to high temperatures.
Crucially, it happens in an environment devoid of sufficient oxygen to support complete combustion.
In essence, pyrolysis is a transformative process. It breaks down complex molecules into simpler, more volatile components.
The Primacy of Pyrolysis in Fire Science
Within the intricate domain of fire science, pyrolysis reigns supreme as the initiating event in the combustion of solid fuels.
Consider a log in a fireplace: before it can erupt into flames, it must first undergo pyrolysis.
The heat causes it to release flammable gases.
These gases then mix with oxygen and ignite, creating the visible fire we observe.
Without this initial pyrolytic breakdown, combustion would be impossible for most solid materials.
Scope and Roadmap: Delving Deeper into Pyrolysis
This exploration will navigate the multi-faceted world of pyrolysis, dissecting its fundamental processes and illuminating the factors that govern its behavior.
We will explore the key parameters influencing it.
These factors include temperature, heating rate, and the composition of the pyrolyzing material itself.
Furthermore, we will connect pyrolysis to real-world fire phenomena.
This connection will be made by illustrating how it contributes to smoke production, flammability, and the escalation of fire events.
Finally, we will touch upon the analytical methods used to study and characterize pyrolysis, providing a comprehensive overview of this critical process.
Unpacking the Process: Fundamental Reactions in Pyrolysis
Having established pyrolysis as the essential first step in the combustion of solid fuels, it's now crucial to dissect the fundamental chemical reactions that underpin this process. Understanding these reactions allows us to predict and potentially control fire behavior.
Thermal Decomposition: The Molecular Breakdown
At its core, pyrolysis is a process of thermal decomposition. This means that heat energy is the driving force that breaks down complex organic molecules into smaller, simpler compounds.
The heat provides the energy necessary to overcome the intramolecular bonds holding the material together.
Think of it like disassembling a complex machine piece by piece. The 'heat' is your toolkit, and the molecules are the machine.
Endothermic Nature: Energy Input is Key
It’s imperative to remember that pyrolysis is an endothermic reaction.
This signifies that it requires a continuous input of energy (heat) to proceed.
Unlike exothermic reactions, which release energy in the form of heat, pyrolysis absorbs heat from its surroundings.
If the heat source is removed, the pyrolysis process will slow down or even cease entirely.
Pyrolysis vs. Combustion: A Critical Distinction
While often confused, pyrolysis and combustion are distinctly different processes. The key differentiator lies in the presence (combustion) or effective absence (pyrolysis) of sufficient oxygen.
Pyrolysis occurs with limited or no oxygen, leading to incomplete decomposition.
Combustion, on the other hand, requires ample oxygen to fully oxidize the fuel, resulting in the release of significant energy as heat and light.
In simpler terms:
-
Pyrolysis: Heat + Organic Material → Gases + Tars + Char (Limited Oxygen)
-
Combustion: Heat + Organic Material + Oxygen → Heat + Light + Carbon Dioxide + Water
The Triad of Products: Gases, Tars, and Char
Pyrolysis yields three primary product streams: gases, tars (or bio-oils), and char (or coke). The relative proportions and specific compositions of these products depend heavily on factors such as temperature, heating rate, and the chemical makeup of the original material.
Pyrolysis Gases: The Ignitable Component
The gaseous fraction typically consists of a mixture of flammable and non-flammable gases, including:
- Methane (CH4)
- Hydrogen (H2)
- Carbon Monoxide (CO)
- Carbon Dioxide (CO2)
- Ethylene (C2H4)
These flammable gases contribute significantly to the fire's propagation. They readily ignite when exposed to an ignition source.
Tars/Bio-Oils: A Complex Mixture
Tars, also known as bio-oils, represent a complex mixture of condensable organic compounds.
They are often viscous and can contribute to the overall fuel load in a fire.
Upon further heating, tars can undergo secondary cracking reactions, producing additional flammable gases and contributing to soot formation.
Char/Coke: The Solid Residue
Char, or coke, is the solid carbonaceous residue left behind after the volatile components have been driven off.
It possesses a high carbon content and can continue to burn if exposed to sufficient oxygen and heat.
The presence of char can influence the rate of fire spread and the overall duration of the burning process.
VOCs: The Invisible Threat
A critical aspect of pyrolysis is the generation of Volatile Organic Compounds (VOCs). These are organic chemicals that have a high vapor pressure at ordinary room temperature.
VOCs released during pyrolysis can significantly impact fire spread and intensity in several ways:
-
Increased Flammability: VOCs contribute to the overall flammability of the environment, lowering the ignition temperature and increasing the rate of flame propagation.
-
Enhanced Fire Intensity: VOCs provide additional fuel for the fire, leading to a higher heat release rate and increased fire intensity.
-
Toxic Smoke Production: Many VOCs are toxic and contribute to the harmful nature of smoke, posing a significant risk to human health.
Understanding the formation and behavior of VOCs is crucial for developing effective fire safety strategies and mitigating the risks associated with pyrolysis-driven fires.
The Pyrolysis Recipe: Key Influencing Factors
Having established pyrolysis as the essential first step in the combustion of solid fuels, it's now crucial to dissect the factors influencing the pyrolysis process.
These factors dictate the speed, intensity, and products of pyrolysis, directly affecting fire behavior.
The Dominant Role of Temperature
Temperature is the prime mover of pyrolysis. It dictates when pyrolysis begins and how vigorously it proceeds.
A material will not undergo pyrolysis until it reaches its specific pyrolysis temperature range, which varies based on its chemical composition.
As temperature increases, the rate of decomposition accelerates, releasing more volatile gases and contributing to a self-sustaining or escalating fire.
Heating Rate: The Speed of Transformation
The rate at which a material is heated significantly impacts the yield and composition of pyrolysis products.
A slower heating rate often promotes char formation, providing more time for secondary reactions to occur within the solid phase.
Conversely, rapid heating favors the production of volatile gases, leading to quicker flame ignition and increased fire spread potential. This is due to rapid release of volatile flammable compounds.
The heating rate is also deeply intertwined with the physical properties of the material, especially its thermal conductivity and thermal inertia.
Materials with low thermal conductivity will pyrolyze differently compared to materials with high thermal conductivity, under the same heating conditions.
Fuel Composition: A Material-Specific Pyrolysis Pathway
Different materials exhibit unique pyrolysis pathways due to their distinct chemical structures.
Wood, primarily composed of cellulose, hemicellulose, and lignin, undergoes complex decomposition. This decomposition yields a range of flammable gases, tars, and a carbonaceous char.
Plastics, with their diverse polymer structures, produce pyrolysis products that vary dramatically. Some plastics produce copious amounts of flammable gases while others create less or produce molten residues.
Textiles, whether natural or synthetic, have their own distinct decomposition characteristics. Natural textiles often behave similar to wood-based materials, and synthetics often behave similar to plastics.
Paper, largely cellulose-based, shares similarities with wood, but its lower density often leads to faster pyrolysis rates.
Moisture Content: A Pyrolysis Inhibitor
Moisture content significantly influences pyrolysis by acting as a heat sink.
Water absorbs energy during vaporization, which delays the material reaching its pyrolysis temperature.
Higher moisture content also increases the energy required to sustain pyrolysis, as water must be continuously evaporated. This reduces the overall intensity of the process.
However, it's important to note that steam produced during this process can also contribute to fire spread under certain conditions.
Fuel Load: Scaling the Pyrolysis Process
The amount of combustible material, or fuel load, directly affects the scale and intensity of pyrolysis in a fire.
A larger fuel load provides more material for pyrolysis, leading to a greater release of flammable gases and increased fire intensity.
Larger fuel loads also create a positive feedback loop. The heat generated by the initial pyrolysis fuels the decomposition of additional material.
This can lead to faster flame spread and greater overall fire damage.
This effect is also deeply dependent on the distribution of the fuel load.
Pyrolysis in Action: Connecting to Real-World Fire Phenomena
Having established pyrolysis as the essential first step in the combustion of solid fuels, it's now crucial to dissect the factors influencing the pyrolysis process. These factors dictate the speed, intensity, and products of pyrolysis, directly affecting fire behavior.
Smoke Composition and its Behavioral Implications
Smoke, a complex aerosol and gas mixture, is a direct byproduct of pyrolysis. Its composition heavily depends on the pyrolyzing material and the conditions of the thermal decomposition. Smoke comprises uncombusted hydrocarbons, soot particles, and various toxic gases such as carbon monoxide, hydrogen cyanide, and acrolein.
The presence of particulate matter drastically reduces visibility, hindering escape and rescue efforts in fire scenarios. Perhaps more insidiously, the toxic gases present in smoke pose a significant threat to human health, causing incapacitation and potentially leading to fatality. Understanding smoke production is paramount in devising effective suppression and ventilation strategies.
Pyrolysis: The Bedrock of Flammability
The flammability of a material is intrinsically linked to its propensity to undergo pyrolysis. Materials that readily decompose into flammable gases when exposed to heat sources will exhibit higher flammability. These gases mix with ambient air to form a combustible mixture.
The lower the temperature required to initiate pyrolysis and the greater the yield of flammable volatiles, the more readily the material will ignite and sustain combustion. Conversely, materials that resist pyrolysis or produce primarily non-flammable byproducts will be more fire-resistant.
Pyrolysis as a Cornerstone of Fire Models
The fire triangle (fuel, heat, and oxygen) and the fire tetrahedron (fuel, heat, oxygen, and chemical chain reaction) are fundamental models in fire science. Pyrolysis firmly resides within the fuel component of these models. It is the process by which solid fuels are transformed into the gaseous state necessary for combustion.
Without pyrolysis, solid materials cannot participate in the chain reaction that sustains a fire. Therefore, understanding pyrolysis is not merely an academic exercise; it is crucial for comprehending the very essence of fire.
Pyrolysis and the Flashover Phenomenon
Flashover represents one of the most dangerous scenarios in structural firefighting. It is the near-simultaneous ignition of all combustible materials within an enclosed space. Pyrolysis plays a pivotal role in the development of flashover.
As a fire develops, the temperature within the compartment rises. This elevated temperature accelerates the pyrolysis of all exposed combustible materials, resulting in a rapid accumulation of flammable gases in the upper layer of the compartment.
When the concentration of these gases reaches the lower flammability limit and the temperature reaches the autoignition temperature, sudden and complete ignition occurs. This rapid transition from a localized fire to a fully developed fire characterizes the flashover event.
Backdraft Explosions: Pyrolysis in Oxygen-Deprived Environments
Backdrafts are explosive events that occur when oxygen is suddenly introduced into a compartment containing a smoldering fire. Pyrolysis, once again, is a key contributor to this phenomenon.
In an oxygen-deprived environment, incomplete combustion occurs, leading to the accumulation of pyrolysis products, particularly flammable gases like carbon monoxide and unburned hydrocarbons. The heat from the smoldering fire sustains the pyrolysis process, further enriching the atmosphere with fuel.
When a door or window is opened, introducing a rush of oxygen, the accumulated flammable gases ignite rapidly, resulting in a violent explosion. Backdrafts highlight the critical importance of understanding how pyrolysis can create hazardous conditions, especially in enclosed spaces with limited ventilation.
Testing the Flames: Analytical Methods for Pyrolysis Study
Having established pyrolysis as the essential first step in the combustion of solid fuels, it's now crucial to dissect the analytical methods used to study and quantify this complex process. These methods provide invaluable data for understanding material behavior under fire conditions, ultimately informing fire safety regulations and product design.
The Role of Analytical Methods in Pyrolysis Research
Analytical methods play a crucial role in deciphering the intricate details of pyrolysis. They allow scientists and engineers to:
- Characterize the pyrolysis products.
- Determine the kinetics of the decomposition process.
- Assess the fire hazard associated with various materials.
This information is vital for developing fire-resistant materials, improving fire suppression techniques, and enhancing our overall understanding of fire dynamics.
The Cone Calorimeter: A Cornerstone of Fire Testing
The Cone Calorimeter stands as a cornerstone in fire testing, providing a standardized and reproducible method for assessing the fire behavior of materials. This instrument simulates real-world fire conditions by exposing a sample to a controlled radiant heat flux, mimicking the thermal radiation from a fire.
The key principle behind the Cone Calorimeter is the measurement of oxygen consumption. It's directly proportional to the heat released during combustion, based on Thornton's Rule.
Key Measurements and Parameters
The Cone Calorimeter allows for the determination of several critical parameters that characterize the fire behavior of a material. These include:
- Heat Release Rate (HRR): The most important parameter, indicating the rate at which heat is released during combustion.
- Time to Ignition (TTI): The time it takes for the sample to ignite under a given heat flux.
- Total Heat Released (THR): The total amount of heat released during the entire combustion process.
- Effective Heat of Combustion (EHC): The amount of heat released per unit mass of volatiles burned.
- Smoke Production Rate (SPR): The rate at which smoke is produced during combustion.
- Mass Loss Rate (MLR): The rate at which the sample loses mass during combustion.
These parameters provide a comprehensive picture of a material's fire performance, enabling comparisons between different materials and assessment of their suitability for various applications.
How the Cone Calorimeter Works
The Cone Calorimeter operates by exposing a horizontally oriented sample to a well-defined radiant heat flux using a conical heater.
The sample is placed on a load cell to measure mass loss. Exhaust gases are collected and analyzed to determine oxygen consumption, which is then used to calculate the heat release rate.
Smoke density is measured using a laser beam obscuration technique. Data acquisition systems record all measurements in real-time, providing a detailed profile of the material's fire behavior.
Applications of Cone Calorimeter Data
Data obtained from Cone Calorimeter testing have wide-ranging applications:
- Material Selection: Allows engineers to choose materials with superior fire resistance for construction, transportation, and other industries.
- Product Development: Helps manufacturers optimize product design to minimize fire hazards.
- Fire Safety Regulations: Provides the scientific basis for developing and enforcing fire safety codes and standards.
- Fire Investigation: Assists in reconstructing fire events and determining the cause and origin of fires.
Video: What is Pyrolysis in Fire? A Comprehensive Guide
FAQs: Pyrolysis in Fire
What's the most important thing to know about pyrolysis in fire?
Pyrolysis in fire is the thermal decomposition of a material due to heat, without oxygen being directly involved. It’s the process that releases flammable gases from solid fuels, which then ignite and cause a flame. Without pyrolysis, most solid fuels wouldn't burn.
How does pyrolysis differ from combustion?
Combustion is the rapid reaction between a substance with an oxidant, usually oxygen, producing heat and light. Pyrolysis precedes combustion in the burning of solid fuels. It's the heat that causes what is pyrolysis in fire, not the presence of oxygen directly. Combustion happens after pyrolysis releases the fuel gases.
What are some examples of materials undergoing pyrolysis in a fire?
Wood is a prime example, breaking down into flammable gases, charcoal, and other byproducts. Plastics also undergo pyrolysis when heated, releasing volatile organic compounds that fuel the fire. Even fabrics and many synthetic materials break down because of what is pyrolysis in fire.
Does pyrolysis always result in flames?
No. While pyrolysis produces flammable gases that can lead to flames, it can also occur in oxygen-poor environments where combustion isn't possible. In these scenarios, the material will char or decompose slowly. Even without flames, what is pyrolysis in fire is still happening due to the heat.
So, next time you see a fire crackling away, remember it's not just flames; it's a complex chemical dance where heat is breaking down materials, starting with pyrolysis in fire. Hopefully, this guide has shed some light on this process and given you a better understanding of what's really going on when things go up in smoke.