Phosphate Ion: Your Ultimate Guide! (You Won't Believe #3!)
The vital role of phosphate ion within biological systems significantly impacts the function of ATP, the primary energy currency of cells. The global demand for phosphorus, a key component of the phosphate ion, underscores its importance in agricultural fertilizers, affecting crop yields worldwide. Understanding the structure and behavior of the phosphate ion is crucial for researchers at institutions such as the Environmental Protection Agency (EPA), who are dedicated to maintaining water quality and mitigating pollution from phosphate-containing compounds. Moreover, insights from experts in biochemistry contribute to a deeper understanding of the phosphate ion's involvement in DNA and RNA structure.
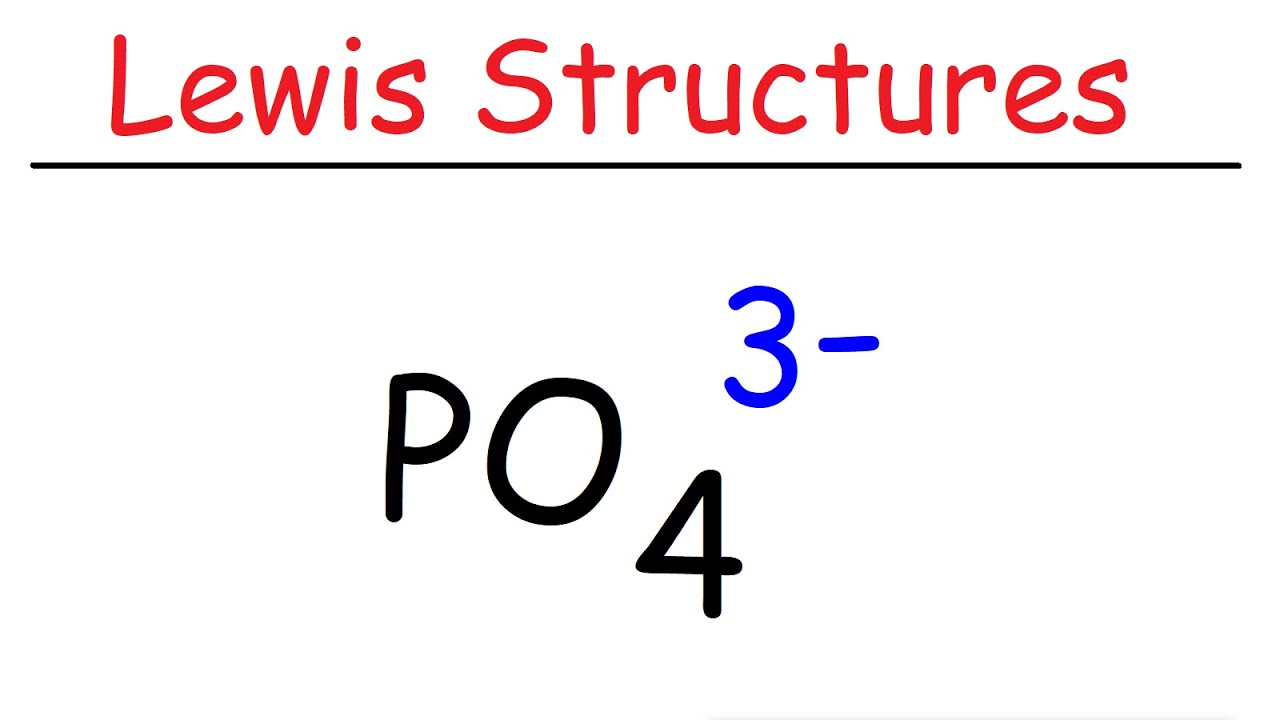
Image taken from the YouTube channel The Organic Chemistry Tutor , from the video titled PO4 3- Lewis Structure - The Phosphate Ion .
The phosphate ion (PO₄³⁻) is a fundamental building block of life and a key player in numerous chemical and industrial processes. From the energy that fuels our cells to the fertilizers that nourish our crops, phosphate is ubiquitous and indispensable.
This guide will explore the multifaceted world of phosphate ions, delving into their structure, properties, roles, and impacts.
Defining the Phosphate Ion (PO₄³⁻): Chemical Structure and Properties
The phosphate ion is a polyatomic ion composed of one central phosphorus atom surrounded by four oxygen atoms in a tetrahedral arrangement. Each oxygen atom carries a partial negative charge, giving the entire ion a -3 charge.
This negative charge is critical to phosphate's ability to form ionic bonds with other positively charged ions, such as calcium and potassium.
The chemical formula PO₄³⁻ represents the simplest form of the phosphate ion. However, under physiological conditions, phosphate exists in equilibrium with its protonated forms: HPO₄²⁻ (hydrogen phosphate) and H₂PO₄⁻ (dihydrogen phosphate).
The relative abundance of each form depends on the pH of the solution.
These different forms of phosphate are crucial for maintaining pH balance in biological systems, acting as a buffer to resist changes in acidity or alkalinity.
Why Phosphate Matters: Exploring the Widespread Importance of Phosphate Ions
Phosphate ions are not merely chemical curiosities; they are essential for life as we know it. Their importance spans across a wide range of disciplines, including:
-
Biology: Phosphate is a core component of DNA and RNA, the molecules that carry genetic information. It is also central to ATP (adenosine triphosphate), the primary energy currency of cells.
-
Chemistry: Phosphate compounds are used in a variety of chemical processes, including the production of fertilizers, detergents, and flame retardants.
-
Medicine: Phosphate plays a role in bone health, kidney function, and various drug delivery systems.
-
Agriculture: Phosphate-based fertilizers are critical for crop production, ensuring adequate yields to feed the global population.
-
Environmental Science: Phosphate levels in water systems are a key indicator of water quality and can influence the health of aquatic ecosystems.
The widespread importance of phosphate underscores its crucial role in maintaining life, driving industrial processes, and shaping our environment.
What to Expect: A Brief Overview of This Comprehensive Guide
This guide is designed to provide a thorough understanding of phosphate ions, covering a range of topics from their fundamental chemistry to their far-reaching impacts.
We will explore the structure and properties of phosphate, its role in biological systems, its environmental implications, and some of its less well-known applications.
By the end of this guide, you will have a comprehensive understanding of the power and importance of phosphate ions in the world around us.
The phosphate ions that sustain life are not spontaneously generated, and the characteristics that make them essential are deeply rooted in chemical principles.
The Chemistry Behind Phosphate
To truly understand the significance of phosphate, we must delve into its chemical underpinnings. This involves examining its structure, formation, relationship to phosphoric acid, and behavior as a buffer.
Phosphate Structure and Properties: Unveiling the Ion's Architecture
The phosphate ion (PO₄³⁻) features a central phosphorus atom bonded to four oxygen atoms. This arrangement forms a tetrahedral structure, where the phosphorus atom sits at the center.
Each oxygen atom carries a partial negative charge, which contributes to the overall -3 charge of the ion. This negative charge is the key to phosphate's interactions with other molecules.
It allows phosphate to readily form ionic bonds with positively charged ions, such as calcium (Ca²⁺) and potassium (K⁺). These ionic interactions are crucial in many biological processes.
Furthermore, the phosphate ion exhibits resonance. This means that the negative charge is distributed across all four oxygen atoms, enhancing its stability.
From Phosphorus to Phosphate: Tracing the Origins
Phosphorus, the central atom in phosphate, is a relatively abundant element on Earth. However, it rarely exists in its elemental form due to its high reactivity.
Instead, phosphorus is typically found in phosphate-containing minerals, such as apatite.
The formation of phosphate ions often begins with the weathering of these minerals. This process releases phosphate into the environment, where it can be taken up by plants and other organisms.
Industrially, phosphate is produced by reacting phosphate rock with sulfuric acid. This reaction generates phosphoric acid (H₃PO₄), which can then be neutralized to form phosphate salts.
Phosphoric Acid's Influence: The Protonation Game
Phosphoric acid (H₃PO₄) plays a pivotal role in the chemistry of phosphate ions. As a triprotic acid, it can donate up to three protons (H⁺) in a stepwise manner.
This deprotonation process leads to the formation of various phosphate species.
These species include: dihydrogen phosphate (H₂PO₄⁻), hydrogen phosphate (HPO₄²⁻), and the phosphate ion (PO₄³⁻).
The relative abundance of each species is determined by the pH of the solution. At low pH, H₃PO₄ predominates, while at high pH, PO₄³⁻ is the most abundant form.
In physiological conditions (pH around 7.4), H₂PO₄⁻ and HPO₄²⁻ are the primary forms. This equilibrium between different phosphate species is essential for maintaining pH balance in biological systems.
Phosphate as a Buffer: Maintaining Equilibrium
Phosphate ions and their protonated forms act as a buffer system in biological fluids. A buffer system resists changes in pH by neutralizing added acids or bases.
When acid (H⁺) is added to a solution containing phosphate, it reacts with PO₄³⁻ to form HPO₄²⁻. HPO₄²⁻ can further react to form H₂PO₄⁻, and so on.
Conversely, when base (OH⁻) is added, it reacts with H₂PO₄⁻ to form HPO₄²⁻, releasing water. This ability to both accept and donate protons allows phosphate to maintain a relatively stable pH, which is crucial for enzymatic activity and other biological processes.
The buffering capacity of phosphate is particularly important in intracellular and extracellular fluids, where pH fluctuations can have detrimental effects on cellular function.
Therefore, the intricate chemistry of phosphate ions, from their structure to their buffering capabilities, is fundamental to understanding their widespread importance in biological and environmental systems.
Phosphate Ions: The Spark of Life
Phosphate, beyond its role in basic chemistry, is an indispensable element in the machinery of life. Its presence is not merely incidental; it is fundamental to the very processes that define biological existence. From powering cellular activities to encoding genetic information and building cellular structures, phosphate ions orchestrate a symphony of functions that are essential for life as we know it.
ATP: The Cellular Energy Currency
Adenosine triphosphate, or ATP, is often referred to as the "energy currency" of the cell. This molecule is the primary carrier of energy in cells. Phosphate ions are integral to ATP's structure and function.
ATP consists of an adenosine molecule attached to a chain of three phosphate groups. The bonds between these phosphate groups are high-energy bonds.
Energy Release from ATP
When a cell requires energy to perform work, the terminal phosphate group of ATP is cleaved off through a process called hydrolysis.
This releases a significant amount of energy that the cell can use to power various activities, such as muscle contraction, nerve impulse transmission, and protein synthesis. The product of this reaction is adenosine diphosphate (ADP) and an inorganic phosphate ion (Pi).
The continuous cycle of ATP hydrolysis and regeneration is central to cellular energy management. Without the readily available phosphate groups in ATP, life would grind to a halt.
Phosphorylation: A Key Regulatory Mechanism
Phosphorylation is the process of adding a phosphate group to a molecule, typically a protein. This process is a crucial regulatory mechanism in cells.
Enzymes called kinases catalyze the transfer of phosphate groups from ATP to specific target proteins. This can alter the protein's activity, conformation, or interaction with other molecules.
Phosphorylation can activate or inactivate enzymes, modulate protein-protein interactions, and influence signal transduction pathways. This is essential for controlling a wide range of cellular processes, including metabolism, growth, and differentiation.
Dephosphorylation, the removal of a phosphate group, is catalyzed by enzymes called phosphatases, and serves to reverse the effects of phosphorylation.
The balance between kinase and phosphatase activity determines the phosphorylation state of a protein and, consequently, its function. This dynamic interplay allows cells to respond rapidly and precisely to changing environmental conditions.
DNA and RNA: The Blueprint of Life
The very blueprint of life, DNA and RNA, owes its structure and stability to phosphate ions. These nucleic acids, which carry genetic information, are composed of chains of nucleotides.
Phosphate's Structural Role in Nucleic Acids
Each nucleotide consists of a sugar molecule (deoxyribose in DNA, ribose in RNA), a nitrogenous base, and one or more phosphate groups.
The phosphate groups link the sugar molecules of adjacent nucleotides together, forming the phosphodiester backbone of the DNA or RNA strand.
This backbone provides the structural framework for the nucleic acid molecule, ensuring its integrity and stability. The negative charge of the phosphate groups also contributes to the overall charge of DNA and RNA, influencing their interactions with other molecules.
The arrangement of the nitrogenous bases along this backbone encodes the genetic information that directs all cellular processes. Without the phosphate groups, the structural integrity of DNA and RNA would be compromised, and the transmission of genetic information would be impossible.
Cell Membrane Integrity
The cell membrane, a critical barrier that separates the interior of a cell from its external environment, relies on phospholipids, which contain phosphate groups, for its structure and function.
Phospholipids are a major component of cell membranes. These molecules have a polar, hydrophilic head containing a phosphate group and two nonpolar, hydrophobic fatty acid tails.
In an aqueous environment, phospholipids spontaneously arrange themselves into a bilayer, with the hydrophobic tails facing inward and the hydrophilic heads facing outward, interacting with the surrounding water.
This bilayer structure forms the foundation of the cell membrane, providing a barrier that is impermeable to most water-soluble molecules. The selective permeability of the cell membrane is crucial for maintaining cellular homeostasis and regulating the transport of molecules into and out of the cell.
Enzymes and Phosphate
Many enzymes rely on phosphate ions for their function. Some enzymes require phosphate as a cofactor, while others are regulated by phosphorylation.
Phosphate ions can bind to the active site of an enzyme, participating directly in the catalytic reaction. In other cases, the binding of phosphate can induce conformational changes in the enzyme, altering its activity.
The interplay between enzymes and phosphate is essential for regulating a wide range of metabolic pathways and cellular processes.
Calcium Phosphate and Hydroxyapatite: Building Strong Bones
Calcium phosphate, particularly in the form of hydroxyapatite [Ca10(PO4)6(OH)2], is a major mineral component of bones and teeth. It provides rigidity and strength to the skeletal system.
Hydroxyapatite crystals are deposited within a matrix of collagen fibers in bone tissue, forming a composite material that is both strong and flexible.
The formation and maintenance of healthy bones depend on the availability of calcium and phosphate ions in the body. Disruptions in calcium and phosphate homeostasis can lead to bone disorders such as osteoporosis and rickets.
Kidney's Role: Regulation of Phosphate Ion Levels
The kidneys play a critical role in regulating phosphate ion levels in the body. They filter phosphate from the blood and reabsorb it back into the bloodstream.
The kidneys regulate phosphate excretion in response to hormonal signals, such as parathyroid hormone (PTH) and fibroblast growth factor 23 (FGF23).
Maintaining proper phosphate balance is essential for bone health, energy metabolism, and various other physiological processes. Kidney dysfunction can lead to phosphate imbalances, which can have significant health consequences.
Phosphate's Environmental Impact
Having explored the intricate roles phosphate plays within living organisms, it’s vital to consider the broader environmental consequences of its presence and distribution. While essential for life, an excess of phosphate can disrupt ecological balances, leading to significant environmental challenges.
This section examines the multifaceted environmental impact of phosphate ions, analyzing both the benefits and detrimental effects, with a particular focus on its use in agriculture, its influence on water quality, and its contribution to eutrophication.
Fertilizers and Agriculture: A Double-Edged Sword
Phosphate-based fertilizers are crucial for modern agriculture, enabling high crop yields necessary to feed a growing global population. These fertilizers provide plants with the essential phosphorus they need for growth, root development, and overall health.
However, the extensive use of these fertilizers has led to a significant increase in phosphate levels in the environment, with far-reaching consequences.
The Phosphate Mining Problem
One of the main issues with phosphate fertilizers is the mining process. Phosphate rock, the raw material for these fertilizers, is a finite resource, and its extraction can have negative environmental consequences.
These consequences include habitat destruction, soil erosion, and water pollution. Furthermore, the geographical concentration of phosphate rock deposits raises concerns about resource depletion and geopolitical dependencies.
Phosphate Runoff: An Unintended Consequence
A significant portion of phosphate applied as fertilizer does not get absorbed by plants. Instead, it runs off into nearby water bodies, such as rivers, lakes, and coastal areas.
This runoff is exacerbated by heavy rainfall, poor soil management practices, and over-application of fertilizers. The accumulation of phosphate in these aquatic ecosystems triggers a cascade of ecological imbalances.
Eutrophication: The Algal Bloom Catastrophe
Eutrophication is the process by which a body of water becomes enriched with nutrients, primarily phosphate and nitrogen, leading to excessive plant and algal growth. This process is often driven by human activities, particularly agricultural runoff and wastewater discharge.
The consequences of eutrophication can be devastating for aquatic ecosystems.
The Algal Bloom Phenomenon
The most visible manifestation of eutrophication is the proliferation of algal blooms. These blooms can consist of various types of algae, including cyanobacteria (blue-green algae), some of which produce potent toxins.
These toxins can contaminate drinking water sources, harm aquatic life, and pose a risk to human health.
Oxygen Depletion and Dead Zones
As algal blooms die and decompose, the process consumes large amounts of oxygen in the water. This oxygen depletion, known as hypoxia, creates "dead zones" where aquatic organisms cannot survive.
These dead zones can decimate fish populations, disrupt food webs, and severely degrade the overall health of aquatic ecosystems.
Impact on Biodiversity
Eutrophication can alter the composition of aquatic communities, favoring species that thrive in nutrient-rich conditions, while suppressing others that are more sensitive to pollution. This loss of biodiversity can have long-term consequences for ecosystem stability and resilience.
Water Quality: Monitoring and Management are Key
Maintaining good water quality requires careful monitoring and management of phosphate levels. Excessive phosphate concentrations can render water unsuitable for drinking, recreation, and irrigation.
Monitoring Phosphate Levels
Regular monitoring of phosphate concentrations in water bodies is essential to assess the extent of pollution and track the effectiveness of management strategies. This monitoring can involve collecting water samples and analyzing them in the laboratory, as well as using remote sensing techniques to detect algal blooms.
Management Strategies
Effective management of phosphate pollution requires a multi-pronged approach that addresses both point and non-point sources of pollution.
This approach includes:
- Implementing best management practices in agriculture to reduce fertilizer runoff.
- Upgrading wastewater treatment plants to remove phosphate from effluent.
- Restoring riparian buffers to filter pollutants before they reach water bodies.
- Promoting the use of alternative fertilizers and sustainable farming practices.
By implementing these strategies, it is possible to mitigate the harmful effects of phosphate pollution and protect the health of aquatic ecosystems.
In conclusion, phosphate, while essential for life and agriculture, poses significant environmental challenges when present in excessive amounts. Understanding the sources, pathways, and impacts of phosphate pollution is crucial for developing effective management strategies to protect water quality and preserve the health of our ecosystems.
Unbelievable Phosphate Fact: The Surprising Side of Phosphate
Beyond its well-established roles in agriculture and biological systems, phosphate plays a surprisingly diverse array of roles in less publicized, yet equally vital, applications. This section aims to illuminate the versatility of phosphate ions, moving beyond the conventional understanding to reveal its fascinating applications in pharmaceuticals and as fire retardants.
Phosphate in Pharmaceuticals: Enhancing Drug Delivery and Bone Regeneration
Phosphate compounds are emerging as valuable assets in pharmaceutical research and development, with applications ranging from targeted drug delivery to promoting bone regeneration. Their biocompatibility and unique chemical properties make them ideal candidates for addressing various challenges in the medical field.
Targeted Drug Delivery Systems
One of the most promising applications of phosphate in pharmaceuticals is in the development of targeted drug delivery systems. By incorporating phosphate groups into drug carriers, researchers can achieve more precise and controlled release of therapeutic agents within the body.
For example, liposomes modified with phosphate moieties can selectively target cells expressing specific phosphate receptors, enhancing drug efficacy while minimizing side effects on healthy tissues. This targeted approach is particularly relevant in cancer therapy, where selective delivery of chemotherapeutic drugs to tumor cells is crucial.
Furthermore, calcium phosphate nanoparticles have gained traction as drug carriers due to their excellent biocompatibility and biodegradability. These nanoparticles can encapsulate drugs and release them in a controlled manner, offering sustained therapeutic effects.
Promoting Bone Regeneration
Phosphate is a key component of bone mineral (hydroxyapatite), making it essential for bone formation and regeneration. Consequently, phosphate-based materials are extensively used in bone grafts, dental implants, and other orthopedic applications.
Calcium phosphate ceramics, such as hydroxyapatite and tricalcium phosphate, are commonly used as bone substitutes due to their osteoconductive properties. These materials provide a scaffold for new bone tissue to grow, accelerating the healing process and restoring bone function.
In addition, phosphate-containing bioactive glasses have shown promise in stimulating bone regeneration. These glasses release ions that promote osteoblast proliferation and differentiation, enhancing bone formation and integration with the surrounding tissues.
Phosphate and Fire Retardants: A Key to Enhanced Safety
Phosphate compounds are widely used as flame retardants in various materials, including plastics, textiles, and coatings. Their effectiveness in inhibiting combustion stems from their ability to interfere with the chemical reactions involved in the burning process.
The Chemistry Behind Phosphate Flame Retardants
Phosphate-based flame retardants primarily function through two mechanisms: the formation of a protective char layer and the release of water molecules during heating.
When exposed to heat, phosphate compounds decompose to form a char layer on the surface of the material. This char layer acts as a barrier, preventing oxygen from reaching the underlying material and slowing down the combustion process.
Some phosphate flame retardants also release water molecules upon heating, which further cools the material and dilutes the concentration of flammable gases. This dual action makes phosphate flame retardants highly effective in preventing or slowing down the spread of fire.
Applications in Various Materials
Phosphate flame retardants are used in a wide range of products to improve fire safety. They are commonly incorporated into plastics used in electronics, furniture, and building materials. In textiles, phosphate-based treatments can make fabrics more resistant to ignition and flame spread.
Furthermore, phosphate coatings are applied to wood and other combustible materials to reduce their flammability. The use of phosphate flame retardants has significantly contributed to reducing fire-related injuries and property damage.
By understanding the surprising applications of phosphate ions in pharmaceuticals and as fire retardants, we gain a deeper appreciation for the versatility and importance of this ubiquitous chemical species. These examples highlight the potential for further innovation and discovery in phosphate chemistry, with implications for human health, safety, and beyond.
The Broader Significance of Phosphate
Having explored the specific roles of phosphate across various domains, it is crucial to appreciate its overarching significance. Phosphate ions are not isolated actors but rather integral components of interconnected systems that sustain life and shape our environment. Their impact resonates deeply within agriculture, environmental science, and human health, revealing a complex web of dependencies that demands careful consideration.
Phosphate in Agriculture: A Cornerstone of Food Production
At the heart of modern agriculture lies the essential role of phosphate. As a primary macronutrient for plants, it fuels vital processes like photosynthesis, energy transfer, and root development. Without sufficient phosphate, crops cannot thrive, leading to stunted growth, reduced yields, and ultimately, compromised food security.
Phosphate fertilizers have become indispensable in boosting agricultural productivity, enabling farmers to cultivate larger areas and achieve higher yields. However, this reliance on phosphate also presents a challenge: ensuring its sustainable use.
Sustainable Farming Practices
The finite nature of phosphate rock, the primary source of phosphate fertilizers, necessitates a shift towards more sustainable farming practices. Efficient fertilizer application methods, such as precision agriculture, can minimize phosphate losses and maximize its uptake by crops.
Cover cropping, crop rotation, and no-till farming are other valuable strategies that enhance soil health, improve nutrient cycling, and reduce the need for synthetic phosphate inputs. These practices not only conserve phosphate resources but also promote long-term soil fertility and environmental stewardship.
Phosphate in Environmental Science: A Delicate Balance
While essential for plant life, excessive phosphate in aquatic ecosystems can trigger a cascade of detrimental effects. Phosphate pollution, primarily from agricultural runoff and wastewater discharge, is a major driver of eutrophication, a process that disrupts the delicate balance of aquatic environments.
Eutrophication leads to algal blooms, which deplete oxygen levels, block sunlight, and harm aquatic organisms. These blooms can also produce toxins that contaminate drinking water and pose risks to human health.
Responsible Phosphate Management
Effective phosphate management is crucial for protecting water quality and preserving aquatic ecosystems. This involves implementing strategies to reduce phosphate inputs from agricultural and urban sources, such as:
- Improving wastewater treatment processes to remove phosphate.
- Implementing buffer strips along waterways to filter runoff.
- Promoting responsible fertilizer use in agriculture.
By adopting a holistic approach that considers the entire phosphate cycle, we can minimize its environmental impacts and ensure the long-term health of our water resources.
Phosphate in Human Health: An Indispensable Nutrient
Phosphate is not only vital for plants and ecosystems but also for human health. It plays a crucial role in numerous physiological processes, including:
- Bone formation and maintenance.
- Energy production (ATP).
- DNA and RNA synthesis.
- Cell signaling.
Bone Health
Approximately 85% of the body's phosphate is stored in bones and teeth, where it combines with calcium to form hydroxyapatite, the primary mineral component of bone. Adequate phosphate intake is essential for maintaining bone strength and preventing conditions like osteoporosis.
Cellular Function and Overall Well-being
Beyond bone health, phosphate is indispensable for cellular function. It is a key component of ATP, the energy currency of cells, and is involved in numerous enzymatic reactions that regulate metabolism, growth, and repair.
Maintaining optimal phosphate levels is crucial for overall well-being. Phosphate deficiency can lead to fatigue, muscle weakness, and impaired cognitive function, while excessive phosphate intake may contribute to kidney problems and cardiovascular disease. A balanced diet that includes phosphate-rich foods, such as dairy products, meat, and legumes, is essential for ensuring adequate phosphate nutrition.
Video: Phosphate Ion: Your Ultimate Guide! (You Won't Believe #3!)
Phosphate Ion FAQ: Understanding the Essentials
Here are some frequently asked questions to further clarify the details surrounding the phosphate ion.
What exactly is a phosphate ion?
A phosphate ion (PO₄³⁻) is an inorganic, polyatomic anion. It's formed when phosphoric acid loses three hydrogen atoms, resulting in a negatively charged ion. It plays a crucial role in various biological processes.
Why is the phosphate ion so important in biology?
Phosphate ions are essential for life. They are a key component of DNA and RNA, the molecules that carry genetic information. Additionally, phosphate ions are involved in energy transfer within cells in the form of ATP (adenosine triphosphate).
What did point #3 in the main article, the "unbelievable" fact, refer to regarding phosphate ions?
The "unbelievable" point likely highlights the surprisingly large amount of phosphate ions that can accumulate in certain environments. This often leads to problems like algal blooms in aquatic ecosystems due to excessive nutrient availability.
How does the concentration of phosphate ion affect water quality?
High concentrations of phosphate ions can negatively impact water quality. Excessive phosphates can lead to eutrophication, an over-enrichment of water bodies with nutrients, causing algal blooms, oxygen depletion, and harm to aquatic life. Monitoring phosphate levels is critical for maintaining healthy aquatic ecosystems.