Nitrogen Charge: Oxidation States & Behavior
Nitrogen, an element extensively researched at institutions like the National Institute of Standards and Technology (NIST), exhibits a wide range of oxidation states influencing its chemical behavior. The Haber-Bosch process, a critical industrial method for synthesizing ammonia, heavily relies on understanding the charge of nitrogen and its reduction from 0 to -3. Accurate determination of nitrogen's oxidation states frequently involves techniques like X-ray photoelectron spectroscopy (XPS), which provides detailed insights into the electronic structure and chemical environment of nitrogen atoms in various compounds. The diverse charge of nitrogen dictates its role in biological systems, industrial processes, and atmospheric chemistry.
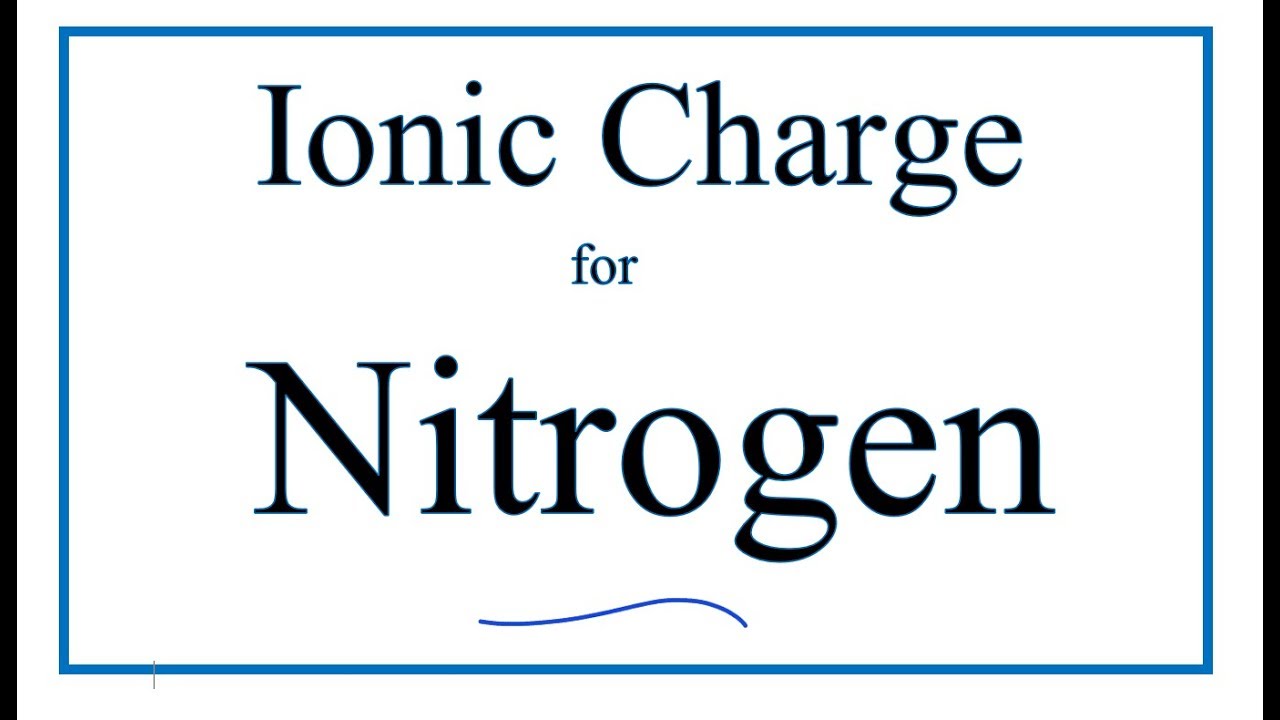
Image taken from the YouTube channel Wayne Breslyn (Dr. B.) , from the video titled Finding the Ionic charge for Nitrogen (N) .
Unveiling Nitrogen's Variable Charge: A Dynamic Perspective
The notion of "charge" associated with a nitrogen atom is not a static, immutable property. It is a dynamic characteristic that fluctuates in response to its molecular surroundings. This inherent variability stems from nitrogen's ability to form a diverse array of chemical bonds and its sensitivity to the electronic influences of neighboring atoms.
Nitrogen's electronegativity, its capacity to attract electrons within a chemical bond, plays a pivotal role in determining its apparent charge. However, electronegativity is only one piece of the puzzle. The type of chemical bond it forms, whether ionic, covalent, or coordinate covalent, also significantly impacts electron distribution and, consequently, its charge.
The Concept of Oxidation States
To understand the charge around nitrogen, one must first grasp the fundamental concepts that govern electron distribution in chemical compounds.
The concept of oxidation states, which represent the hypothetical charge an atom would have if all its bonds were perfectly ionic, provides a useful framework.
Furthermore, formal charge, which is calculated assuming equal sharing of electrons in covalent bonds, offers another perspective on the electronic environment of nitrogen.
Beyond the Basics
These values can be used to assess the stability of different possible structures.
However, it's crucial to recognize that these are merely models, and the true charge distribution within a molecule is often more complex.
The Impact of Environment
The charge on a nitrogen atom is not an intrinsic property but rather an emergent characteristic influenced by its immediate chemical environment.
Factors such as the identity of neighboring atoms, the presence of specific functional groups, the pH of the solution, the polarity of the solvent, and even the temperature can all exert a significant influence on the electronic environment of nitrogen and, consequently, its observed charge.
Relevance Across Disciplines
Understanding the dynamic nature of nitrogen's charge is essential for a multitude of scientific disciplines.
In chemistry, it dictates reaction mechanisms and the stability of various nitrogen-containing compounds.
In biology, it governs the function of nitrogenous bases in DNA and RNA, as well as the activity of enzymes that catalyze nitrogen metabolism.
In environmental science, it sheds light on the complex transformations of nitrogen in the atmosphere, soil, and water, impacting everything from fertilizer design to water quality monitoring.
Core Concepts: Defining Charge Around Nitrogen
The notion of "charge" associated with a nitrogen atom is not a static, immutable property.
It is a dynamic characteristic that fluctuates in response to its molecular surroundings.
This inherent variability stems from nitrogen's ability to form a diverse array of chemical bonds.
To understand this dynamic behavior, it's critical to delve into the core theoretical frameworks that chemists use to assign "charge" to nitrogen atoms within molecules.
Oxidation State/Oxidation Number
The oxidation state, or oxidation number, is a fundamental concept in chemistry.
It represents the hypothetical charge an atom would have if all its bonds were perfectly ionic.
This is a useful bookkeeping tool, but it's crucial to remember it's a simplification.
Determining Oxidation States
Assigning oxidation states follows a set of established rules.
For example, oxygen is usually -2, and hydrogen is usually +1.
Using these rules, we can determine the oxidation state of nitrogen in various compounds.
In ammonia (NH3), nitrogen has an oxidation state of -3.
In nitric acid (HNO3), nitrogen has an oxidation state of +5.
Limitations and Conventions
Oxidation states are useful, but they have limitations.
They don't always accurately reflect the actual charge distribution in a molecule, especially in covalent compounds.
Also, there are conventions to follow when assigning oxidation states in complex molecules, ensuring consistency.
Electronegativity and Bond Polarity
Electronegativity is a measure of an atom's ability to attract electrons in a chemical bond.
Nitrogen is more electronegative than hydrogen but less electronegative than oxygen.
This difference in electronegativity influences the polarity of bonds involving nitrogen.
Influence on Charge Distribution
When nitrogen bonds with hydrogen, the nitrogen atom acquires a partial negative charge (δ-) because it pulls electron density toward itself.
Conversely, when nitrogen bonds with oxygen, the oxygen atom acquires a partial negative charge (δ-), and nitrogen acquires a partial positive charge (δ+).
These partial charges, while not full ionic charges, contribute to the overall charge distribution around the nitrogen atom.
Chemical Bonding and Electron Distribution
The type of chemical bond significantly impacts the electron distribution around nitrogen.
Ionic Bonds
In ionic bonds, electrons are essentially transferred from one atom to another.
While nitrogen doesn't readily form purely ionic bonds, considering this extreme case helps illustrate the concept.
Covalent Bonds
Covalent bonds involve the sharing of electrons between atoms.
Nitrogen commonly forms covalent bonds, and the sharing can be equal (nonpolar) or unequal (polar), depending on the electronegativity difference.
Coordinate Covalent Bonds
Coordinate covalent bonds occur when one atom provides both electrons for the bond.
Ammonium ion (NH4+) is an example.
Nitrogen donates its lone pair to a proton (H+).
Formal Charge
Formal charge is another method of assigning charge, assuming electrons are shared equally in a covalent bond.
Calculating Formal Charge
The formula for calculating formal charge is:
Formal Charge = (Valence Electrons) - (Non-bonding Electrons + 1/2 Bonding Electrons).
Assessing Molecular Stability
Formal charge helps assess the stability of different Lewis structures for the same molecule.
Structures with minimal formal charges on atoms are generally more stable.
Lewis Structures: Visualizing Bonding
Lewis structures are diagrams that show the bonding and lone pairs of electrons in a molecule.
They're essential for understanding the electronic environment around nitrogen.
Drawing Lewis Structures
Drawing Lewis structures for nitrogen-containing compounds involves:
- Determining the total number of valence electrons.
- Arranging the atoms and drawing single bonds.
- Distributing the remaining electrons as lone pairs.
- Satisfying the octet rule (or duet rule for hydrogen).
Applying the Octet Rule
Nitrogen typically follows the octet rule, meaning it tends to form bonds to achieve eight electrons in its valence shell.
Resonance Structures: Delocalized Charge
Resonance occurs when multiple valid Lewis structures can be drawn for the same molecule.
Understanding Resonance
Resonance structures represent different possible arrangements of electrons.
The actual molecule is a hybrid of these structures.
Effect on Charge Distribution
Resonance leads to delocalization of charge, meaning the charge is spread out over multiple atoms, rather than being localized on a single atom.
Nitrous oxide (N2O) is a prime example.
Simplifications vs. Reality: Limitations of Models
It's crucial to remember that oxidation states, formal charges, and Lewis structures are models, not actual representations of charge.
Limitations
These models have limitations and don't always accurately depict the electron distribution in a molecule.
More sophisticated methods, such as computational chemistry, are needed for a more precise understanding.
Context Matters: Environmental Influences
The "charge" around nitrogen is highly dependent on its environment.
Neighboring Atoms and Functional Groups
The atoms and functional groups surrounding nitrogen significantly influence its electronic environment.
Electron-withdrawing groups increase nitrogen's partial positive charge.
Electron-donating groups increase nitrogen's partial negative charge.
pH, Solvent, and Temperature
External factors like pH, solvent, and temperature can also affect nitrogen's charge.
Trends and Patterns: Common Oxidation States
Nitrogen exhibits a range of oxidation states, from -3 to +5.
Prevalence and Stability
The most common oxidation states include -3 (in ammonia), 0 (in N2), and +5 (in nitrates).
The stability of each oxidation state depends on the chemical environment.
Nitrogen Compounds: A Spectrum of Charges
The notion of "charge" associated with a nitrogen atom is not a static, immutable property. It is a dynamic characteristic that fluctuates in response to its molecular surroundings. This inherent variability stems from nitrogen's ability to form a diverse array of chemical bonds. To understand this variability more concretely, let's examine a range of nitrogen-containing compounds and analyze the charge distribution within each.
Simple Inorganic Compounds
We begin with simple inorganic compounds to illustrate fundamental principles.
Ammonia (NH3) and the Ammonium Ion (NH4+)
In ammonia (NH3), nitrogen is covalently bonded to three hydrogen atoms. Due to nitrogen's higher electronegativity, it pulls electron density towards itself, resulting in a partial negative charge and an oxidation state of -3.
The nitrogen atom also possesses a lone pair of electrons. This lone pair is crucial for ammonia's ability to act as a Lewis base.
Upon protonation, ammonia forms the ammonium ion (NH4+). While the overall ion has a +1 charge, the oxidation state of nitrogen remains -3.
The positive charge is distributed across the entire ion, with each hydrogen atom bearing a partial positive charge.
Dinitrogen (N2)
Nitrogen gas (N2) is remarkably stable due to the strong triple bond between the two nitrogen atoms.
Because the two atoms are identical, the electron density is shared equally. The oxidation state of each nitrogen atom is 0.
This lack of polarity contributes to N2's inertness under normal conditions.
Oxides of Nitrogen: From Nitrous Oxide to Nitrogen Dioxide
Nitrogen forms a variety of oxides, each exhibiting distinct properties and oxidation states.
-
Nitrous oxide (N2O), also known as laughing gas, features nitrogen in a mixed oxidation state. One nitrogen atom is typically assigned an oxidation state of +1 and the other, -1. Resonance structures contribute to the overall charge distribution, which helps to stabilize the molecule.
-
Nitric oxide (NO) is a radical molecule with an unpaired electron. Nitrogen carries an oxidation state of +2. NO plays an important role in biological signaling and atmospheric chemistry.
-
Nitrogen dioxide (NO2) is a reddish-brown gas and a significant component of smog. In NO2, the oxidation state of nitrogen is +4. It is a potent oxidizing agent and contributes to acid rain.
Nitric Acid (HNO3) and Nitrate/Nitrite Ions
Nitrogen reaches its highest common oxidation state (+5) in nitric acid (HNO3).
Nitric acid is a strong acid and a powerful oxidizing agent.
Nitric acid readily loses a proton to form the nitrate ion (NO3-).
Nitrates are widely used as fertilizers. The nitrate ion has a planar structure with the negative charge delocalized over the three oxygen atoms.
Nitrites (NO2-), on the other hand, have a bent structure. They are used as food preservatives, particularly in cured meats.
The charge distribution and reactivity of nitrites differ significantly from nitrates due to their structural difference.
Organic Nitrogen Compounds
Nitrogen is a fundamental element in organic molecules, playing a key role in the structure and function of biomolecules.
Amines and Amides
Amines are organic derivatives of ammonia, with one or more hydrogen atoms replaced by alkyl or aryl groups. Amines are classified as primary, secondary, or tertiary, depending on the number of carbon atoms bonded to the nitrogen.
Amides are formed from the reaction of a carboxylic acid with an amine. The nitrogen atom in an amide is less basic than in an amine due to the resonance stabilization of the amide group. This resonance also influences the charge distribution around the nitrogen.
Special Cases: Azides, Cyanides, and Diazonium Salts
Azides (N3-) are linear anions with three nitrogen atoms. They are known for their explosive properties. The charge is delocalized across the three nitrogen atoms, contributing to their instability.
Cyanide (CN-) features a triple bond between carbon and nitrogen. Nitrogen in cyanide has an oxidation state of -3. Cyanide is highly toxic because it inhibits cellular respiration.
Diazonium salts (RN2+) are important intermediates in organic synthesis, particularly in the production of azo dyes. The diazonium group (N2+) is attached to an organic group (R). Diazonium salts are unstable and readily decompose, releasing nitrogen gas. This decomposition is exploited in various industrial processes.
By examining these diverse nitrogen compounds, we see that the "charge" on a nitrogen atom is not a fixed value. It is a dynamic property influenced by bonding, electronegativity, and the overall molecular structure. The ability of nitrogen to adopt various oxidation states and participate in different bonding arrangements underpins its essential role in chemistry, biology, and environmental science.
Nitrogen in Action: Natural and Applied Systems
[Nitrogen Compounds: A Spectrum of Charges The notion of "charge" associated with a nitrogen atom is not a static, immutable property. It is a dynamic characteristic that fluctuates in response to its molecular surroundings. This inherent variability stems from nitrogen's ability to form a diverse array of chemical bonds. To understand this behavior fully, it's crucial to explore nitrogen's roles within various systems, both natural and human-engineered.]
Nitrogen, in its myriad oxidation states, is not a mere spectator in the grand theater of chemical reactions; it's a key player. From fueling the intricate dance of life to driving industrial processes, nitrogen's chemical versatility is on full display.
The Nitrogen Cycle: A Global Redox Symphony
The nitrogen cycle stands as a testament to the dynamic interplay of oxidation and reduction involving nitrogen compounds. This biogeochemical cycle is not just a series of reactions, but a carefully orchestrated system that sustains life on Earth.
Nitrogen fixation, the linchpin of the cycle, converts inert atmospheric nitrogen (N2) into bioavailable forms like ammonia (NH3). This process, primarily carried out by certain bacteria and archaea, often associated symbiotically with plants, is essential for introducing nitrogen into the food chain. Industrially, the Haber-Bosch process mimics this, albeit at high energy costs, to produce ammonia-based fertilizers.
Nitrification follows, oxidizing ammonia to nitrite (NO2-) and then to nitrate (NO3-), processes mediated by specific groups of bacteria. Nitrate is the primary form of nitrogen absorbed by plants.
However, the cycle also features a reductive counterpoint, denitrification. Here, bacteria convert nitrate back into atmospheric nitrogen, completing the cycle and preventing excessive nitrogen buildup in ecosystems.
Ammonification is the process where organic nitrogen (from dead plants or animals) is converted back into ammonia by decomposers.
Human activities have significantly disrupted the delicate balance of the nitrogen cycle. The widespread use of synthetic fertilizers, while boosting agricultural yields, has led to nitrogen runoff into waterways. This triggers eutrophication, causing algal blooms and oxygen depletion, harming aquatic life.
Redox Versatility: Nitrogen as Oxidizer and Reducer
Nitrogen exhibits a remarkable capacity to act as both an oxidizing and a reducing agent, depending on its chemical environment. In compounds where nitrogen possesses a low oxidation state (e.g., NH3), it can readily donate electrons, acting as a reductant. Conversely, in compounds with high oxidation states (e.g., HNO3), nitrogen readily accepts electrons, acting as an oxidant.
Nitrous oxide (N2O), a potent greenhouse gas, provides a compelling example. It can be both a product and a reactant in redox reactions, contributing to atmospheric chemistry and climate change. The controlled manipulation of nitrogen redox chemistry is also fundamental to many industrial processes, including the production of polymers and pharmaceuticals.
Nitrogen in Biological Systems: The Building Blocks of Life
Nitrogen is an indispensable element in biological systems, forming the backbone of crucial biomolecules.
Amino acids, the building blocks of proteins, all contain at least one nitrogen atom. The arrangement and sequence of these amino acids, dictated by genetic code, determine the unique properties of each protein.
Proteins perform a vast array of functions, from catalyzing biochemical reactions (enzymes) to providing structural support (collagen).
Nucleic acids (DNA and RNA), the carriers of genetic information, also heavily rely on nitrogen. The nitrogenous bases (adenine, guanine, cytosine, thymine/uracil) are responsible for encoding and transmitting hereditary information.
Nitrogen metabolism, encompassing the processes of nitrogen assimilation, biosynthesis, and excretion, is crucial for maintaining cellular homeostasis. Disruptions in nitrogen metabolism can lead to various metabolic disorders.
Video: Nitrogen Charge: Oxidation States & Behavior
FAQs: Nitrogen Charge, Oxidation States & Behavior
How does nitrogen's electronegativity affect its charge in compounds?
Nitrogen is highly electronegative. This means it strongly attracts electrons in chemical bonds. When bonded to less electronegative elements, nitrogen usually gains electron density, resulting in a partial or formal negative charge.
What is the range of oxidation states nitrogen can exhibit?
Nitrogen exhibits a wide range of oxidation states, from -3 to +5. This versatility allows it to form diverse compounds. The specific charge of nitrogen in a given compound depends on the other elements it's bonded to and the overall molecular structure.
Why is nitrogen gas (N₂) so unreactive?
Nitrogen gas features a triple bond between the two nitrogen atoms, requiring significant energy to break. This strong bond makes N₂ relatively inert under normal conditions. Therefore, the charge of nitrogen in N₂ is zero.
How does the charge of nitrogen influence the acidity or basicity of nitrogen-containing compounds?
The charge of nitrogen affects the compound's ability to donate or accept protons. For example, in ammonia (NH₃), the partial negative charge of nitrogen makes it a base because it readily accepts a proton. Conversely, in nitric acid (HNO₃), the positive charge of nitrogen makes it acidic.
So, next time you're thinking about molecules, remember our friend nitrogen. Its versatility in bonding and reactivity is all thanks to its ability to sport so many different faces, charge of nitrogen wise, ranging from happily accepting electrons to willingly donating them. Pretty neat, huh?