Nitride Ion: Unlock The Secrets Every Chemist Should Know
The stability of lithium nitride provides crucial insights into the behavior of the nitride ion in various chemical environments. Density functional theory, a powerful computational tool, allows researchers to predict and understand the properties of compounds containing the nitride ion. The application of Karl Landsteiner's principles on chemical specificity extends to the study of the nitride ion's interactions with other elements. As such, the Max Planck Institute actively supports research into the synthesis and characterization of novel materials incorporating the nitride ion, promising advancements in fields like catalysis and materials science.
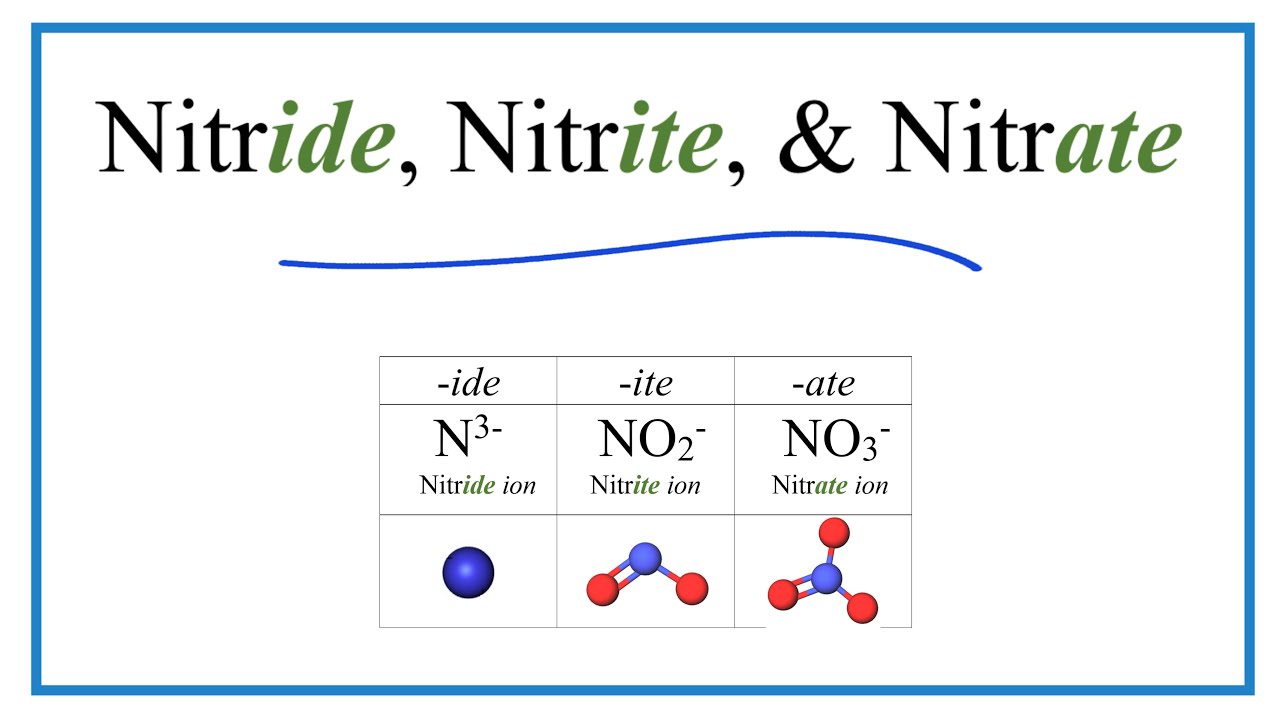
Image taken from the YouTube channel Wayne Breslyn (Dr. B.) , from the video titled Nitride, Nitrite, and Nitrate Ions (Difference and Formulas) .
Unveiling the Enigmatic Nitride Ion
The nitride ion, represented as N3-, stands as a pivotal, yet often understated, species in the vast landscape of chemistry. Its unique characteristics and versatile behavior make it a cornerstone in diverse fields, ranging from inorganic synthesis to advanced materials science.
Understanding the nitride ion's fundamental properties is crucial for unlocking its potential and harnessing its capabilities in various technological applications. This section serves as an introduction to this fascinating anion, setting the stage for a deeper exploration of its chemistry and applications.
Defining the Nitride Ion
At its core, the nitride ion is a nitrogen atom that has gained three electrons, resulting in a formal charge of -3. This triply-charged anion is relatively small and highly electronegative.
Nitrogen, belonging to Group 15 of the periodic table, possesses five valence electrons. To achieve a stable octet configuration, it readily accepts three additional electrons, transforming into the nitride ion.
This electron gain significantly alters nitrogen's chemical behavior, transforming it from a relatively inert diatomic gas (N2) into a highly reactive anionic species. The resulting N3- ion exhibits a strong tendency to form bonds with electropositive elements.
Significance Across Scientific Disciplines
The nitride ion's significance spans several scientific disciplines, each benefiting from its unique properties and reactivity.
Inorganic Chemistry
In inorganic chemistry, nitrides play a crucial role in the synthesis of novel compounds and the stabilization of unusual oxidation states. Their ability to form strong bonds with a wide range of elements makes them essential building blocks for creating complex inorganic structures.
Solid-State Chemistry
Solid-state chemistry relies heavily on nitrides for designing and synthesizing advanced materials with tailored properties. Nitride-based ceramics, for example, exhibit exceptional hardness, high thermal stability, and resistance to chemical attack.
Materials Science
Materials science recognizes the nitride ion's contribution to creating materials with exceptional mechanical, electronic, and optical characteristics. From high-performance cutting tools to light-emitting diodes (LEDs), nitride-containing materials are ubiquitous in modern technology.
A Brief Historical Perspective
The study of nitrides has a rich history, dating back to the early days of chemical research. Early chemists recognized the ability of nitrogen to react with metals at high temperatures, forming compounds with unique properties.
However, the precise nature of the nitride ion and its bonding characteristics remained elusive for many years.
Key milestones in nitride research include:
- Early observations: Initial discoveries of metal nitrides through high-temperature reactions.
- Structural determination: Elucidation of the crystal structures of various nitride compounds using X-ray diffraction techniques.
- Applications development: The realization of the potential of nitrides in various technological applications, such as ceramics, semiconductors, and coatings.
Today, nitride research continues to be a vibrant and active area of investigation. Scientists are constantly exploring new synthetic routes, characterizing novel nitride materials, and discovering innovative applications for these versatile compounds.
The Nitride Ion: A Deep Dive into Fundamental Properties
Having established the broad significance of the nitride ion, it's crucial to now examine the fundamental properties that govern its behavior. These underlying characteristics dictate its reactivity and influence its role in forming diverse compounds. Let's unravel the atomic and chemical attributes that define this intriguing anion.
The Central Role of Nitrogen
Nitrogen, the fifth element in Group 15, holds the key to understanding the nitride ion. Its electronic configuration is [He] 2s² 2p³, revealing five valence electrons.
This configuration dictates nitrogen's eagerness to acquire three additional electrons to achieve a stable octet, mirroring the electron arrangement of the noble gas neon. This quest for stability is the primary driving force behind the formation of the N³⁻ ion.
The acquisition of these three electrons results in a significant increase in negative charge density, transforming the relatively neutral nitrogen atom into a highly reactive, triply-charged anion. This dramatic shift in electronic structure profoundly impacts its interactions with other elements.
Electronegativity: A Guiding Force
Nitrogen boasts a high electronegativity value (Pauling scale of 3.04), surpassed by only a few elements like fluorine and oxygen. Electronegativity quantifies an atom's ability to attract electrons towards itself in a chemical bond.
Nitrogen's high electronegativity plays a crucial role in determining the nature of the bonds it forms. When nitrogen bonds with less electronegative elements, such as alkali and alkaline earth metals, it readily pulls electron density towards itself, resulting in the formation of highly polar or even ionic bonds.
This electron-attracting capability makes nitrogen an exceptional electron acceptor, fostering the formation of strong, stable bonds with electropositive elements. The resulting compounds often exhibit unique structural and electronic properties, making them highly valuable in various applications.
Anion Formation and Stability
The formation of the nitride ion is an endothermic process, requiring energy input to overcome the electron repulsion and promote the addition of three electrons. However, the subsequent formation of stable compounds containing the nitride ion releases a significant amount of energy, compensating for the initial energy investment.
Several factors contribute to the stability of the nitride ion once formed.
Primarily, the attainment of a noble gas configuration confers inherent stability. The completely filled valence shell provides a low-energy, stable electronic state.
Furthermore, the strong electrostatic interactions between the N³⁻ ion and the surrounding cations in a compound contribute significantly to the overall lattice energy, enhancing the stability of the resulting nitride material.
The smaller size and higher charge density of the nitride ion compared to other common anions like oxide (O²⁻) or fluoride (F⁻) also contribute to its ability to form strong and stable ionic lattices. This high charge density leads to stronger electrostatic attractions, further stabilizing the nitride compounds.
Chemical Bonding with Nitrides: From Theory to Practice
Having established the nitride ion's fundamental characteristics, specifically its high electronegativity, it's time to explore how these attributes translate into the realm of chemical bonding. The strong negative charge and inherent reactivity of N³⁻ dictate the types of interactions it engages in with other elements, leading to a rich tapestry of compounds with diverse properties.
Formation of Ionic Compounds Containing Nitride Ions
The nitride ion, with its -3 charge, is a prime candidate for forming ionic compounds, particularly with electropositive elements like alkali and alkaline earth metals. These interactions are governed by the principles of electrostatic attraction, where the negatively charged nitride ion is drawn towards positively charged cations.
The process begins with the transfer of electrons from the metal atom to the nitrogen atom, resulting in the formation of respective ions. For example, in the formation of lithium nitride (Li₃N), each lithium atom donates one electron to the nitrogen atom, achieving a stable electronic configuration for both.
These ionic compounds exhibit characteristic properties, including high melting and boiling points, due to the strong electrostatic forces holding the ions together in the crystal lattice. They are also generally hard and brittle and often good electrical insulators in the solid state.
Types of Chemical Bonding in Nitride Compounds
While ionic bonding is prevalent, particularly with highly electropositive elements, the reality of chemical bonding in nitride compounds is often more nuanced. Covalent character can also manifest depending on the electronegativity difference between nitrogen and the other element involved.
For example, in nitrides formed with transition metals or elements with intermediate electronegativity, the bonding can exhibit significant covalent character. In these cases, the electron density is not entirely transferred from one atom to another but rather shared between them.
This leads to a bonding continuum, ranging from purely ionic to partially covalent, affecting the physical and chemical properties of the resulting compounds. For instance, nitrides with significant covalent character tend to have lower melting points and can exhibit semiconducting properties.
Lattice Energy and Its Influence on Nitride Compound Stability
Lattice energy is a crucial factor determining the stability and properties of ionic compounds. It represents the energy released when gaseous ions combine to form a solid crystalline lattice.
A high lattice energy indicates a strong attraction between the ions, leading to a more stable compound. Several factors influence lattice energy, including the charge of the ions, their size, and the crystal structure.
Since the nitride ion carries a high charge (-3), nitride compounds tend to have relatively high lattice energies, contributing to their stability and high melting points. However, the relatively large size of the nitride ion, compared to other common anions like oxide or fluoride, can somewhat reduce the lattice energy.
The interplay of these factors dictates the overall stability and properties of nitride compounds, making lattice energy a critical consideration in understanding their behavior. A nuanced understanding of the interplay between ionic character, covalent contributions, and lattice energy provides valuable insights into the diverse and fascinating world of nitride compounds.
Having explored the fundamental principles governing chemical bonding with nitrides, the stage is now set to examine the concrete manifestations of these interactions. The diverse array of nitride compounds, each with its unique structure and properties, showcases the versatility of the nitride ion in materials science.
Exploring the Diverse Structures and Properties of Nitride Compounds
The world of nitride compounds extends far beyond simple ionic interactions. The structural arrangements and resulting properties are dictated by a delicate interplay of factors, including ionic radii, electronegativity differences, and the electronic configurations of the constituent elements. Let's delve into the fascinating realm of nitride structures and their implications.
Unveiling Common Crystal Structures
The crystal structures of nitride compounds are critical in understanding their macroscopic properties. Several common structure types recur throughout the nitride family, each imparting specific characteristics to the material.
-
Rock Salt Structure: This structure, adopted by many alkali metal nitrides (e.g., Li₃N), features a simple cubic arrangement with alternating cations and anions. Its relative simplicity often leads to predictable properties.
-
Wurtzite and Zinc Blende Structures: These structures, prevalent in semiconducting nitrides like GaN and AlN, are characterized by tetrahedral coordination of the constituent atoms. This arrangement contributes to their unique electronic and optical properties.
-
Layered Structures: Some nitrides, particularly those with larger cations, adopt layered structures that facilitate intercalation and exfoliation. This opens avenues for creating two-dimensional materials with novel applications.
These structural motifs directly influence the mechanical, electrical, and optical properties of the resulting nitride compounds.
Nitrides of Alkali and Alkaline Earth Metals
The electropositive nature of alkali and alkaline earth metals leads to the formation of predominantly ionic nitrides.
Alkali Metal Nitrides: These compounds (e.g., Li₃N, Na₃N) are generally synthesized by direct reaction of the metal with nitrogen gas at elevated temperatures.
Lithium nitride stands out for its relatively high stability. It exhibits a layered structure and serves as a fast ion conductor. Sodium and potassium nitrides are less stable and more challenging to synthesize.
Alkaline Earth Metal Nitrides: Alkaline earth metals form nitrides with the general formula M₃N₂ (e.g., Mg₃N₂, Ca₃N₂, Sr₃N₂, Ba₃N₂). Magnesium nitride (Mg₃N₂) is a common source of ammonia upon reaction with water. Calcium nitride (Ca₃N₂) finds use in refractory materials. These nitrides tend to be more refractory than their alkali metal counterparts, owing to the higher charge and smaller ionic radii of the alkaline earth cations.
Transition Metal Nitrides: A Realm of Diversity
Transition metal nitrides (TMNs) represent a diverse class of compounds with a wide range of compositions and properties. Unlike alkali and alkaline earth metal nitrides, TMNs often exhibit significant covalent character in their bonding. This leads to a diverse array of structures and properties.
-
Hardness and Refractoriness: Many TMNs, such as titanium nitride (TiN) and chromium nitride (CrN), are exceptionally hard and possess high melting points. This makes them suitable for wear-resistant coatings and high-temperature applications.
-
Electrical Conductivity: Some TMNs exhibit metallic conductivity, while others are semiconductors or insulators. This wide range of electronic properties makes them attractive for various electronic and optoelectronic applications.
-
Catalytic Activity: Certain TMNs demonstrate catalytic activity in various chemical reactions. This positions them as potential catalysts in industrial processes.
The specific properties of TMNs depend on the electronic configuration of the transition metal, the nitrogen content, and the crystal structure.
Interstitial Nitrides: Filling the Gaps
Interstitial nitrides are formed when nitrogen atoms occupy interstitial sites within a metal lattice.
-
Formation and Stability: These compounds are typically formed at high temperatures and pressures. The inserted nitrogen atoms strengthen the metal lattice, leading to increased hardness and strength.
-
Property Modification: The presence of interstitial nitrogen atoms can significantly alter the electrical, magnetic, and mechanical properties of the host metal. This allows for tailoring materials for specific applications.
-
Examples and Applications: Examples include iron nitrides (Fe₄N, Fe₂N) which enhance the surface hardness of steel. They also offer improved corrosion resistance.
The study of interstitial nitrides provides a pathway for creating advanced materials with enhanced performance characteristics.
Having explored the fundamental principles governing chemical bonding with nitrides, the stage is now set to examine the concrete manifestations of these interactions. The diverse array of nitride compounds, each with its unique structure and properties, showcases the versatility of the nitride ion in materials science.
Spotlight on Key Nitride Materials: GaN, BN, and Si₃N₄
The world of nitride materials is rich and varied, but some compounds have risen to prominence due to their exceptional properties and wide-ranging applications. Gallium Nitride (GaN), Boron Nitride (BN), and Silicon Nitride (Si₃N₄) stand out as prime examples of how the unique characteristics of the nitride ion can be harnessed to create materials with groundbreaking capabilities.
Let's delve into the specifics of each of these key nitride materials, exploring their structures, properties, and the applications that have made them indispensable in modern technology.
Gallium Nitride (GaN): A Semiconductor Revolution
GaN has revolutionized the fields of electronics and optoelectronics, particularly in recent years. Its exceptional properties have enabled the development of more efficient and powerful devices.
Properties and Advantages of GaN
GaN is a wide bandgap semiconductor, which means that it requires a significant amount of energy to excite electrons from the valence band to the conduction band. This characteristic gives GaN several advantages over traditional semiconductors like silicon:
- Higher Breakdown Voltage: GaN can withstand much higher voltages than silicon before breaking down, making it suitable for high-power applications.
- Higher Switching Speed: Electrons can move through GaN faster than silicon, enabling faster switching speeds in electronic devices.
- Higher Thermal Conductivity: GaN dissipates heat more efficiently, allowing devices to operate at higher temperatures without overheating.
GaN in Semiconductor Applications
These properties make GaN ideal for use in power amplifiers, high-frequency transistors, and other demanding electronic applications. GaN-based transistors are now commonly used in:
- Radio Frequency (RF) Electronics: GaN power amplifiers are used in cellular base stations, radar systems, and satellite communications.
- Power Electronics: GaN transistors are replacing silicon transistors in power supplies, motor drives, and electric vehicle chargers, improving efficiency and reducing size.
GaN in Optoelectronics: Lighting the Future
GaN is also a key material in the development of light-emitting diodes (LEDs) and laser diodes. By varying the composition of GaN with other elements like indium and aluminum, the wavelength of emitted light can be tuned across the visible spectrum and beyond.
- LED Lighting: GaN-based LEDs are the dominant technology in modern lighting, offering superior energy efficiency and long lifetimes compared to traditional incandescent and fluorescent bulbs.
- Laser Diodes: GaN laser diodes are used in Blu-ray players, laser pointers, and medical devices.
Advancements in GaN Technology
Ongoing research is focused on improving the quality and reducing the cost of GaN materials, as well as developing new GaN-based devices with enhanced performance. Some key areas of advancement include:
- GaN-on-Silicon: Growing GaN on silicon substrates can significantly reduce the cost of GaN devices, making them more accessible for widespread use.
- Vertical GaN Devices: Vertical GaN transistor structures offer improved power handling capabilities and are being developed for high-voltage applications.
Boron Nitride (BN): A Versatile Ceramic Material
Boron Nitride (BN) presents itself in diverse structural forms, exhibiting unique properties that make it invaluable in high-temperature and advanced material applications.
Structural Forms of Boron Nitride
Boron Nitride exists in several different structural forms, each with its own distinct properties:
- Hexagonal Boron Nitride (h-BN): The most common form of BN, h-BN has a layered structure similar to graphite. It is an excellent electrical insulator and thermal conductor.
- Cubic Boron Nitride (c-BN): This form of BN has a diamond-like structure and is one of the hardest materials known. It is used as an abrasive and cutting tool material.
- Wurtzite Boron Nitride (w-BN): Similar to the wurtzite structure found in GaN, w-BN is another hard, high-pressure form of Boron Nitride.
Applications in High-Temperature Materials
The exceptional thermal stability and chemical inertness of BN make it an ideal material for high-temperature applications:
- Refractory Materials: BN is used in crucibles, furnace linings, and other refractory components that must withstand extreme temperatures.
- High-Temperature Lubricants: h-BN is used as a dry lubricant in high-temperature environments where traditional lubricants would break down.
Other Applications of Boron Nitride
Beyond high-temperature applications, BN is also used in a variety of other fields:
- Cosmetics: h-BN is used in cosmetics and personal care products due to its lubricating and absorbent properties.
- Electronics: h-BN is used as an insulating layer in electronic devices and as a substrate for graphene electronics.
Silicon Nitride (Si₃N₄): A High-Strength Ceramic
Silicon Nitride (Si₃N₄) stands out as a high-performance ceramic material renowned for its remarkable strength, wear resistance, and thermal shock resistance.
Properties of Silicon Nitride
Si₃N₄ possesses a unique combination of properties that make it suitable for demanding applications:
- High Strength and Hardness: Si₃N₄ is a very strong and hard material, capable of withstanding high stresses and abrasive wear.
- High-Temperature Stability: Si₃N₄ retains its strength and hardness at high temperatures, making it suitable for use in high-temperature environments.
- Thermal Shock Resistance: Si₃N₄ can withstand rapid changes in temperature without cracking or fracturing.
- Chemical Inertness: Si₃N₄ is resistant to attack by most chemicals.
Applications in Ceramics and High-Strength Materials
These properties make Si₃N₄ an ideal material for a wide range of ceramic and high-strength applications:
- Engine Components: Si₃N₄ is used in engine components such as turbocharger rotors, valves, and bearings, which can withstand high temperatures and stresses.
- Cutting Tools: Si₃N₄ cutting tools are used for machining hard materials such as cast iron and hardened steel.
- Bearings: Si₃N₄ bearings are used in high-speed and high-precision applications such as machine tools and aerospace equipment.
- Medical Implants: Si₃N₄ is biocompatible and can be used in medical implants such as hip and knee replacements.
The enduring relevance of GaN, BN, and Si₃N₄ underscores the critical function of nitride compounds in materials science. Their distinct attributes have facilitated technological breakthroughs, and continued research guarantees even more novel uses in the future.
Having explored the fundamental principles governing chemical bonding with nitrides, the stage is now set to examine the concrete manifestations of these interactions. The diverse array of nitride compounds, each with its unique structure and properties, showcases the versatility of the nitride ion in materials science.
Nitride Ions: A Cornerstone of Materials Science and Technology
The nitride ion (N3-) stands as a pivotal building block in the realm of modern materials science. Its ability to form robust and diverse compounds has enabled breakthroughs across a spectrum of technological domains. From revolutionizing electronics to enhancing structural integrity in advanced materials, the influence of nitrides is undeniable.
This section will explore the profound impact of nitride ions on materials development. It will also examine the specific applications of key nitride compounds – Gallium Nitride (GaN), Boron Nitride (BN), and Silicon Nitride (Si₃N₄) – highlighting their transformative contributions to various industries.
The Indispensable Role of Nitrides in Advanced Materials
The incorporation of nitride ions into materials often leads to dramatic enhancements in their physical and chemical properties. This stems from several factors, including the strong, directional nature of the bonds formed by nitrogen, as well as its ability to stabilize unique crystal structures.
Hardness, thermal stability, and chemical inertness are frequently amplified in nitride-containing materials, making them ideal candidates for demanding applications.
The presence of the nitride ion can also influence electronic band structure. It can tailor optical properties, unlocking possibilities for novel electronic and optoelectronic devices.
GaN: Powering the Future of Electronics and Optoelectronics
Gallium Nitride (GaN) has emerged as a disruptive force in the electronics and optoelectronics industries, largely due to its wide bandgap and high electron mobility. These characteristics enable the creation of devices that operate at higher frequencies, higher power levels, and higher temperatures compared to those based on traditional semiconductors like silicon.
Applications of GaN:
-
Power Electronics: GaN-based transistors are replacing silicon in power supplies, electric vehicle inverters, and other applications demanding high efficiency and compact size.
-
Radio Frequency (RF) Electronics: GaN amplifiers are crucial components in cellular base stations, radar systems, and satellite communications, offering superior performance at microwave frequencies.
-
Light-Emitting Diodes (LEDs): GaN is the foundation for blue and green LEDs, which are used in energy-efficient lighting, displays, and medical devices. The development of GaN LEDs has revolutionized the lighting industry.
-
Laser Diodes: GaN laser diodes are employed in Blu-ray players, laser pointers, and industrial applications, providing high-power and high-resolution beams.
BN: A Versatile Material for High-Temperature Applications
Boron Nitride (BN) exhibits exceptional thermal stability and chemical inertness. This makes it an invaluable material in applications where resistance to extreme conditions is paramount.
Applications of BN:
-
High-Temperature Lubricants: BN's layered structure allows it to function as a solid lubricant at high temperatures where conventional lubricants would degrade.
-
Crucibles and Furnace Linings: BN's resistance to chemical attack and high melting point make it ideal for containing molten metals and protecting furnace components.
-
Cosmetics: BN powder is used in cosmetics to improve texture and provide a smooth feel.
-
Cutting Tools: Cubic boron nitride (c-BN) is the second-hardest material known, after diamond, and is used as an abrasive in cutting tools for machining hardened steels and other difficult-to-cut materials.
Si₃N₄: Enhancing Strength and Durability in Structural Materials
Silicon Nitride (Si₃N₄) is renowned for its high strength, wear resistance, and thermal shock resistance. This combination of properties makes it a vital component in various structural applications.
Applications of Si₃N₄:
-
Engine Components: Si₃N₄ is used in engine components, such as bearings and turbocharger rotors, to reduce friction, improve fuel efficiency, and withstand high temperatures.
-
Cutting Tools: Si₃N₄ cutting tools exhibit excellent wear resistance. This makes them suitable for machining cast iron, steel, and other materials.
-
Ball Bearings: Silicon nitride's hardness and smoothness make it an excellent material for ball bearings used in high-speed and high-precision applications.
-
High-Temperature Bearings: Si₃N₄ bearings are used in high-temperature environments where steel bearings would fail.
The examples of GaN, BN, and Si₃N₄ demonstrate the transformative potential of nitride materials across diverse technological sectors. Their unique properties, stemming from the incorporation of the nitride ion, have enabled groundbreaking innovations in electronics, optoelectronics, structural materials, and beyond. As research continues to unlock new possibilities, the nitride ion is poised to remain a cornerstone of materials science and technology for years to come.
Having explored the indispensable role of nitrides in advanced materials, from enhancing structural integrity to revolutionizing electronics, it's crucial to acknowledge the hurdles and future prospects that lie ahead in this dynamic field. While the potential of nitride-based materials is vast, realizing their full capabilities requires addressing significant challenges and embracing emerging research trends.
Challenges and Future Horizons in Nitride Research
The field of nitride research stands at a fascinating crossroads. While the existing applications of nitride compounds have already had a transformative impact, significant obstacles remain in the path of further progress. Overcoming these challenges and capitalizing on new research directions will be key to unlocking the full potential of these remarkable materials.
The Synthesis Gauntlet: Purity and Perfection
One of the primary hurdles in nitride research lies in the synthesis of high-purity nitride compounds. The formation of nitrides often requires extreme conditions, such as high temperatures and pressures. These conditions can lead to unintended impurities and defects in the resulting materials.
These imperfections can significantly impact the desired properties of the nitride compound. For example, the presence of oxygen impurities in GaN can reduce its efficiency in optoelectronic devices. Similarly, defects in Si₃N₄ can weaken its mechanical strength.
Several strategies are being explored to overcome these synthetic challenges. These include:
- Advanced Precursor Chemistry: Developing novel precursors that decompose cleanly and efficiently into the desired nitride phase.
- High-Pressure Synthesis Techniques: Refining high-pressure methods to minimize defect formation and control stoichiometry.
- Thin-Film Deposition Methods: Employing techniques like molecular beam epitaxy (MBE) and atomic layer deposition (ALD) to create highly controlled, thin films of nitrides.
Charting the Course: Current Research Trends
The world of nitride research is not static. It evolves continuously, with new trends and discoveries emerging regularly. Several key areas are currently at the forefront of nitride research.
One prominent trend is the exploration of novel nitride compositions and structures. Researchers are investigating new combinations of elements to create nitrides with tailored properties. For example, alloying GaN with other semiconductors like indium nitride (InN) and aluminum nitride (AlN) allows for precise control over the bandgap, enabling the creation of light-emitting diodes (LEDs) that emit across the entire visible spectrum.
Another exciting area is the development of nitride-based nanostructures. Nanowires, nanotubes, and quantum dots made from nitride materials exhibit unique properties due to their size and shape. These nanostructures hold promise for applications in high-performance transistors, sensors, and energy storage devices.
Furthermore, significant effort is being directed towards improving the performance and reliability of existing nitride devices. This includes developing new doping strategies, optimizing device architectures, and mitigating the effects of defects and impurities.
Peering into the Crystal Ball: Future Applications
The future of nitride-based materials is bright, with potential applications spanning a wide range of industries.
- Power Electronics: GaN and AlN are poised to revolutionize power electronics. Their high breakdown voltages and switching speeds make them ideal for use in high-efficiency power converters, electric vehicles, and renewable energy systems.
- Optoelectronics: Nitride-based LEDs are already ubiquitous in lighting and displays, and future advancements will lead to even brighter, more efficient, and more versatile light sources.
- High-Temperature Materials: Nitrides like Si₃N₄ and AlN are well-suited for high-temperature applications due to their exceptional thermal stability and mechanical strength. They can be used in aerospace components, cutting tools, and wear-resistant coatings.
- Quantum Computing: Certain nitride materials are being explored as potential building blocks for quantum computers. Their unique electronic and optical properties could enable the creation of qubits, the fundamental units of quantum information.
In conclusion, while challenges remain in the synthesis and processing of nitride compounds, the ongoing research efforts and the vast potential applications paint an optimistic picture for the future of this field. Continued innovation and collaboration will be essential to unlocking the full potential of nitride materials and ushering in a new era of technological advancement.
Video: Nitride Ion: Unlock The Secrets Every Chemist Should Know
FAQs: Nitride Ion Secrets Revealed
Hopefully this article has clarified the importance of the nitride ion. Here are some frequently asked questions to further expand your understanding:
What exactly is a nitride ion?
A nitride ion is a nitrogen atom that has gained three electrons, giving it a -3 charge (N3-). It's a relatively small, highly charged anion.
How reactive is the nitride ion?
The nitride ion is extremely reactive due to its high negative charge density. It readily forms strong bonds with various cations, especially metals. This high reactivity makes it key to creating very stable nitrides.
Where are nitride ions commonly found?
Nitride ions are not found in isolation in nature. They are primarily found in solid-state compounds called nitrides. Examples include transition metal nitrides used in coatings and semiconductor materials like gallium nitride (GaN).
Why are nitride compounds so stable?
The high stability of nitride compounds comes from the strong electrostatic attraction between the highly charged nitride ion (N3-) and positively charged cations. The strong bond energy makes them resistant to decomposition and high temperatures.