Macromolecules: Unveiling Life's Tiny Building Blocks
Macromolecules, the cornerstones of life, dictate every biological process, from cellular respiration to genetic inheritance. These giant molecules, explored extensively by institutions like the National Institutes of Health (NIH), are constructed from smaller, repeating units. The central question, what are the building blocks of macromolecules, is answered by identifying four key classes: carbohydrates, lipids, proteins, and nucleic acids. Each of these classes, often studied using techniques like chromatography, is made of simpler subunits. For instance, carbohydrates utilize monosaccharides, while proteins are assembled from amino acids. Understanding Linus Pauling's pioneering work on protein structure helps to further appreciate the complexity arising from these simple components. Similarly, the cell's activities in different environments depend greatly on the macromolecule structure and the building blocks the macromolecule is made of. These building blocks determine the ultimate structure and function of these vital molecular giants.
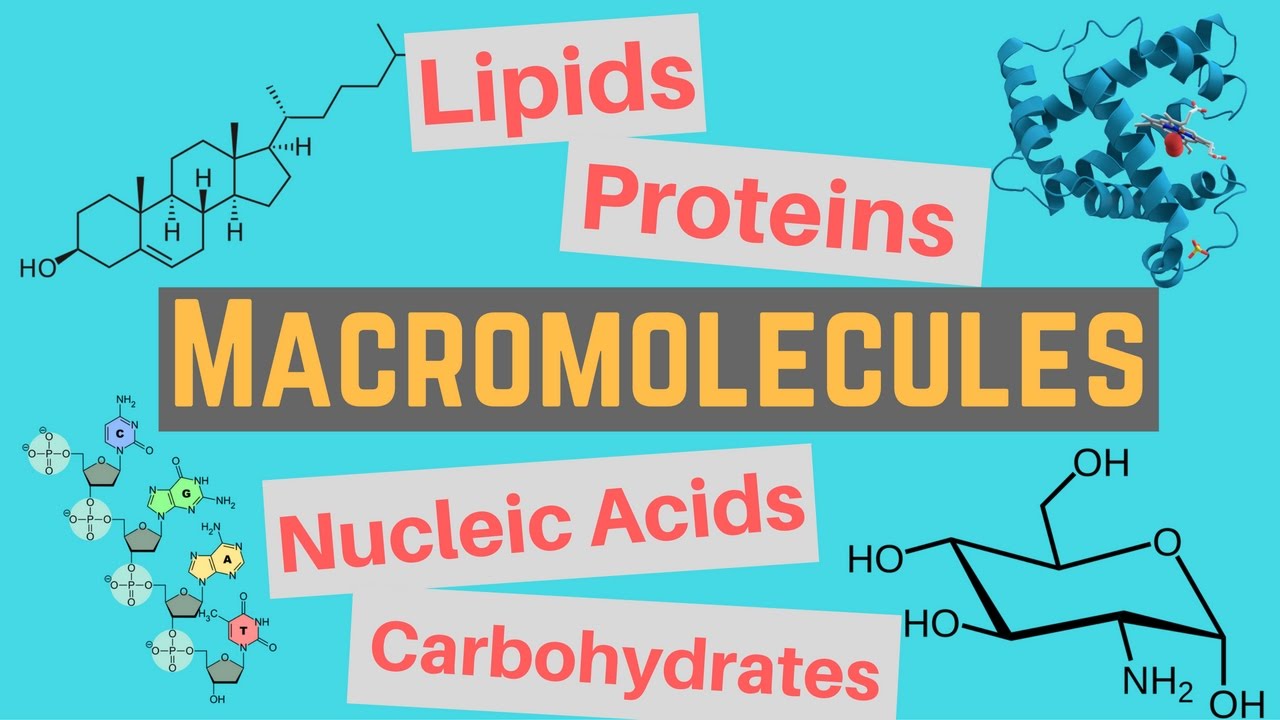
Image taken from the YouTube channel 2 Minute Classroom , from the video titled Macromolecules | Classes and Functions .
Life, in all its complexity and splendor, is fundamentally built upon a relatively small set of molecular components. Among these, macromolecules stand out as the workhorses and structural components of every living organism. Understanding these giant molecules is crucial to grasping the intricacies of biological processes, from the energy we derive from food to the genetic information that dictates our very being.
What are Macromolecules?
Macromolecules, as the name suggests, are large molecules formed by the polymerization of smaller repeating units. Think of them as long chains meticulously constructed from individual building blocks. They are essential for life because they perform a vast array of functions, from providing structural support and storing energy to catalyzing biochemical reactions and encoding genetic information.
In essence, macromolecules are the cornerstones of biological architecture and the engines that drive cellular processes. Without them, life as we know it would be impossible.
The Four Major Classes
There are four primary classes of macromolecules that are ubiquitous in living systems:
-
Carbohydrates: Primarily function as a source of energy and also play a structural role in some organisms.
-
Lipids: Involved in energy storage, insulation, and the formation of cell membranes.
-
Proteins: Perform a staggering variety of functions, including acting as enzymes, providing structural support, transporting molecules, and regulating cellular processes.
-
Nucleic acids: Store and transmit genetic information.
Each of these classes has a unique structure and performs specific roles within the cell, contributing to the overall complexity and functionality of living organisms.
Monomers: The Key to Macromolecular Diversity
A common thread runs through all these macromolecules: they are all polymers, meaning they are constructed from smaller, repeating units called monomers. These monomers are like individual Lego bricks that, when linked together in specific sequences, create structures with distinct properties.
The diversity of macromolecules arises from the variety of monomers available and the different ways in which they can be linked together. Just as a limited number of letters can form countless words, a relatively small set of monomers can give rise to the immense diversity of macromolecules found in nature.
Our Objective
The objective here is to embark on a journey to explore the specific monomers that constitute each type of macromolecule. We will delve into the unique characteristics of each monomer, understand how they are linked together to form larger structures, and appreciate the relationship between monomer composition and macromolecular function. By understanding these fundamental building blocks, we can unlock a deeper understanding of the complexities of life itself.
Life, in all its complexity and splendor, is fundamentally built upon a relatively small set of molecular components. Among these, macromolecules stand out as the workhorses and structural components of every living organism. Understanding these giant molecules is crucial to grasping the intricacies of biological processes, from the energy we derive from food to the genetic information that dictates our very being.
The intricate tapestry of life is woven from large, complex molecules called macromolecules, but their construction relies on a simpler principle: the assembly of smaller, fundamental units. Just as a bricklayer uses individual bricks to build a wall, biological systems utilize monomers to construct the diverse array of macromolecules essential for life.
Monomers: The Foundation of Macromolecular Structures
Monomers are the foundational building blocks of macromolecules, the individual units that, when linked together, create the polymers responsible for the structure and function of living organisms. Understanding the nature of monomers is key to unraveling the secrets of macromolecular architecture.
Defining the Basic Building Blocks
At their core, monomers are small organic molecules that have the remarkable ability to bind to one another. This linking process is the key to creating larger, more complex structures. Think of them as individual beads on a string, each unique but capable of joining to form a longer chain.
Each class of macromolecule—carbohydrates, lipids, proteins, and nucleic acids—has its own characteristic set of monomers. These monomers possess specific chemical properties that dictate how they interact and assemble, leading to the diversity of macromolecular structures found in nature.
The Polymerization Process
Monomers don't exist in isolation. They actively participate in a process called polymerization, where they covalently bond to one another to form longer chains. These chains, known as polymers, are the macromolecules that carry out essential biological functions.
Polymerization is not a random event. It is a carefully orchestrated process, often facilitated by enzymes, that ensures monomers are joined in a specific order and orientation. This precise arrangement is crucial for determining the overall structure and function of the resulting macromolecule.
Dehydration Synthesis: The Bonding Mechanism
The most common mechanism for linking monomers is dehydration synthesis. During this process, a water molecule (H₂O) is removed as two monomers form a covalent bond. One monomer donates a hydroxyl group (OH), and the other donates a hydrogen atom (H), resulting in the formation of water and a new bond between the monomers.
This process requires energy and is typically catalyzed by enzymes, which lower the activation energy needed for the reaction to occur.
Diversity from Simple Units
Despite the relative simplicity of individual monomers, their diversity gives rise to an astounding range of macromolecular structures and functions. Just as the 26 letters of the alphabet can be combined to create countless words and sentences, the variety of monomers allows for the construction of a vast library of macromolecules.
The specific arrangement, sequence, and chemical properties of monomers within a macromolecule determine its unique characteristics. It is this diversity at the monomer level that ultimately drives the complexity and functionality of living systems. Without this diversity, life as we know it simply could not exist.
Monomers serve as life’s fundamental bricks, and understanding their individual properties and the ways they combine is crucial to understanding the nature of the vast and diverse world of macromolecules. We can now delve deeper into the specific types of monomers that construct each class of these biological giants, beginning with the essential sugars and starches that fuel our bodies and provide structural support to plants.
Carbohydrates: Sugars and Their Monosaccharide Building Blocks
Carbohydrates are arguably the most readily accessible source of energy for most living organisms, and they also play critical roles in structural support, particularly in the plant kingdom. These versatile molecules range from simple, sweet-tasting sugars to complex, long-chain polymers, all built from a common foundation: monosaccharides.
Defining Monosaccharides: The Simple Sugar Foundation
Monosaccharides, or simple sugars, are the fundamental building blocks of all carbohydrates. They are the monomers that, when linked together through glycosidic bonds, form more complex carbohydrates like disaccharides and polysaccharides.
These single-unit sugars typically contain three to seven carbon atoms and exist in either linear or ring structures. The arrangement of these carbons, along with their associated hydroxyl (-OH) and carbonyl (C=O) groups, dictates the unique properties of each monosaccharide.
Common Examples of Monosaccharides
While a vast array of monosaccharides exist in nature, a few stand out due to their prevalence and importance in biological systems.
-
Glucose: Often referred to as "blood sugar," glucose is a primary source of energy for cells. It is readily broken down through cellular respiration to produce ATP, the cell's energy currency.
-
Fructose: Known as "fruit sugar," fructose is naturally found in fruits and honey. It is significantly sweeter than glucose and is often used in processed foods and beverages.
-
Galactose: This monosaccharide is a component of lactose, or "milk sugar." It is also found in some fruits and vegetables.
These three monosaccharides—glucose, fructose, and galactose—are isomers, meaning they share the same chemical formula (C6H12O6) but differ in their structural arrangement.
This subtle difference in structure leads to distinct properties, such as varying sweetness levels and metabolic pathways.
From Monosaccharides to Complex Carbohydrates
The real magic of carbohydrates lies in the ability of monosaccharides to link together, forming more complex structures with diverse functions.
Disaccharides: Two Sugars Linked Together
When two monosaccharides join via a glycosidic bond, formed through dehydration synthesis, a disaccharide is created.
Common examples include:
- Sucrose (table sugar): Glucose + Fructose
- Lactose (milk sugar): Glucose + Galactose
- Maltose (malt sugar): Glucose + Glucose
Polysaccharides: Long Chains of Sugar Units
Polysaccharides are large, complex carbohydrates composed of many monosaccharides linked together. These polymers can be linear or branched, and they serve various roles, including energy storage and structural support.
-
Starch: The primary energy storage molecule in plants, starch is a polymer of glucose. Plants store starch in the form of granules, which can be broken down into glucose when energy is needed.
-
Glycogen: Animals store glucose in the form of glycogen, primarily in the liver and muscles. Glycogen is a highly branched polymer of glucose, allowing for rapid mobilization of glucose when energy demands increase.
-
Cellulose: A major structural component of plant cell walls, cellulose is a linear polymer of glucose. Its unique arrangement of glycosidic bonds makes it indigestible by most animals, although it serves as an important source of dietary fiber.
-
Chitin: Found in the exoskeletons of insects and crustaceans, as well as in the cell walls of fungi, chitin is a structural polysaccharide composed of modified glucose units.
The diversity of carbohydrates, from simple monosaccharides to complex polysaccharides, reflects their versatility and essential role in sustaining life. Their structures dictate their function and are crucial in providing energy and building biological structures.
Carbohydrates, with their monosaccharide foundations, provide the energy and structural support essential for life. But the true workhorses of the cell, the molecules responsible for catalyzing reactions, transporting substances, and providing structural integrity, are proteins. These incredibly versatile macromolecules are built from a different set of monomers, each contributing unique properties to the final protein product.
Proteins: Amino Acids as the Monomers of Life's Workhorses
Proteins are the most diverse and functionally sophisticated macromolecules in living systems. They orchestrate nearly every aspect of cellular life, acting as enzymes that speed up biochemical reactions, structural components that provide support and shape to cells and tissues, transport molecules that carry vital substances throughout the body, hormones that coordinate physiological processes, antibodies that defend against foreign invaders, and motor proteins that enable movement.
The sheer breadth of protein function is a testament to the remarkable diversity encoded within their structures.
Defining Amino Acids: The Building Blocks of Proteins
Just as monosaccharides are the monomers of carbohydrates, amino acids are the monomers that constitute proteins. Each amino acid possesses a central carbon atom bonded to four groups: an amino group (-NH2), a carboxyl group (-COOH), a hydrogen atom (-H), and a unique side chain, also known as an R-group.
It is this R-group that distinguishes each amino acid from the others and dictates its specific chemical properties.
The Peptide Bond: Linking Amino Acids Together
Amino acids are joined together through peptide bonds, which are formed via a dehydration reaction between the carboxyl group of one amino acid and the amino group of another. This process releases a water molecule and creates a covalent bond between the two amino acids.
Multiple amino acids linked together through peptide bonds form a polypeptide chain.
A protein can consist of one or more polypeptide chains, precisely folded and arranged in three-dimensional space.
The 20 Common Amino Acids: A Symphony of Diversity
While hundreds of amino acids exist in nature, only 20 are commonly found in proteins. These 20 amino acids are categorized based on the properties of their R-groups:
-
Nonpolar, hydrophobic amino acids: These amino acids have R-groups that are primarily composed of hydrocarbons, making them water-repelling. They tend to cluster together within the interior of a protein, away from the aqueous environment.
-
Polar, hydrophilic amino acids: These amino acids have R-groups that contain electronegative atoms, such as oxygen or nitrogen, making them water-attracting. They tend to be located on the surface of a protein, interacting with the surrounding water.
-
Charged amino acids (acidic or basic): These amino acids have R-groups that are either positively charged (basic) or negatively charged (acidic) at physiological pH. These charged amino acids play crucial roles in protein folding, stability, and interactions with other molecules.
The Role of Side Chains in Protein Structure and Function
The unique chemical properties of each amino acid’s side chain profoundly influence the overall structure and function of the protein. The sequence of amino acids in a polypeptide chain, dictated by the genetic code, determines how the protein will fold and interact with other molecules.
For instance, the arrangement of hydrophobic amino acids can create pockets for binding nonpolar molecules, while charged amino acids can form salt bridges that stabilize the protein structure.
Therefore, understanding the properties of the 20 common amino acids is essential for comprehending the intricate relationship between protein structure and function. These seemingly simple building blocks, when arranged in specific sequences and folded into precise three-dimensional shapes, give rise to the incredible diversity and functional power of proteins, the workhorses of the cell.
Carbohydrates, with their monosaccharide foundations, provide the energy and structural support essential for life. But the true workhorses of the cell, the molecules responsible for catalyzing reactions, transporting substances, and providing structural integrity, are proteins. These incredibly versatile macromolecules are built from a different set of monomers, each contributing unique properties to the final protein product. Now, let’s shift our focus to another class of essential biological molecules: lipids. Though they don’t always follow the strict polymer-from-monomer structure seen in carbohydrates and proteins, they are crucial for energy storage, insulation, and the very structure of our cells.
Lipids: Fatty Acids and Glycerol as Key Components
Lipids, often referred to as fats, oils, and waxes, constitute a diverse group of hydrophobic molecules vital to life. While they might not always adhere to the classic polymer structure formed from repeating monomer units, they are assembled from smaller building blocks. These components contribute to lipids' diverse functions within living organisms. Lipids play critical roles in long-term energy storage, providing insulation against the cold, and forming the structural basis of cell membranes. Their unique chemical properties enable them to perform functions distinct from carbohydrates, proteins, and nucleic acids.
The Multifaceted Roles of Lipids
Lipids are indispensable for a multitude of biological processes. Their primary role is energy storage. Fats, specifically triglycerides, serve as a highly efficient way to store energy reserves for later use.
Lipids also act as insulators, protecting organisms from extreme temperature fluctuations. This is particularly important for animals living in cold environments.
Furthermore, lipids are essential components of cell membranes, forming a barrier that separates the cell's interior from its external environment.
Lipids Are Assembled, Not Always Polymerized
Unlike carbohydrates, proteins, and nucleic acids, lipids are not strictly polymers in the conventional sense. They are not formed by the repetitive linkage of identical monomer units. Instead, lipids are assembled from a variety of components. The primary building blocks are fatty acids and glycerol. These components combine to form diverse lipid structures such as triglycerides, phospholipids, and steroids.
Fatty Acids: The Energy-Rich Chains
Fatty acids are long hydrocarbon chains with a carboxyl group (-COOH) at one end. These chains are the primary source of energy stored within many lipids. They can be saturated, containing only single bonds between carbon atoms, or unsaturated, containing one or more double bonds. Saturated fatty acids tend to be solid at room temperature (like butter), while unsaturated fatty acids are typically liquid (like olive oil). The saturation level of fatty acids influences the physical properties and biological functions of the lipids they compose.
Glycerol: The Backbone Builder
Glycerol is a three-carbon alcohol that serves as the backbone for many lipids, particularly triglycerides. In triglycerides, three fatty acids are attached to glycerol through ester linkages, forming a molecule that is highly efficient for energy storage. The process of attaching fatty acids to glycerol involves dehydration synthesis, where a water molecule is removed for each bond formed. The resulting triglyceride molecule is hydrophobic and readily stored in adipose tissue.
Triglycerides: Efficient Energy Reservoirs
Triglycerides are the most abundant lipids in living organisms. They are primarily used for long-term energy storage. These molecules are highly concentrated sources of energy, providing more than twice the energy per gram compared to carbohydrates or proteins. Stored in specialized cells called adipocytes, triglycerides serve as an essential reserve that can be mobilized when energy demands increase.
Phospholipids: The Architects of Cell Membranes
Phospholipids are a class of lipids that are crucial components of cell membranes. They consist of a glycerol molecule attached to two fatty acids and a phosphate group. The phosphate group is polar and hydrophilic ("water-loving"), while the fatty acid tails are nonpolar and hydrophobic ("water-fearing"). This amphipathic nature allows phospholipids to form bilayers in aqueous environments, creating the structural basis of cell membranes.
Nucleic Acids: Nucleotides, the Genetic Code Builders
Having examined the roles of lipids in energy storage and cellular structure, we now turn to the information-bearing molecules of life: nucleic acids. These complex macromolecules, DNA and RNA, are the blueprints and instruction manuals for all living organisms, dictating everything from eye color to enzyme production. Understanding their structure, particularly the nature of their constituent monomers, is fundamental to grasping the very essence of heredity and genetic expression.
The Role of DNA and RNA
Nucleic acids, deoxyribonucleic acid (DNA) and ribonucleic acid (RNA), are the cornerstones of genetic information storage, replication, and expression in all known forms of life. DNA serves as the long-term repository of genetic instructions, a carefully guarded archive containing the blueprints for building and maintaining an organism. RNA, on the other hand, acts as a versatile messenger, intermediary, and even a functional enzyme, participating directly in the processes that translate the genetic code into tangible biological products.
Both DNA and RNA are polymers, meaning they are constructed from repeating monomeric units. These monomers are called nucleotides, and their precise sequence determines the genetic information encoded within the nucleic acid molecule. It’s the specific order of these nucleotides that dictates the traits and characteristics of an organism, making them the essential alphabet of life.
Nucleotides: The Monomers of Nucleic Acids
Each nucleotide is itself a composite molecule, built from three essential components: a sugar, a phosphate group, and a nitrogenous base.
Sugar Component
The sugar component differs between DNA and RNA. In DNA, the sugar is deoxyribose, while in RNA, it is ribose. The subtle difference—deoxyribose lacks one oxygen atom compared to ribose—has profound implications for the stability and function of the two nucleic acids. DNA’s deoxyribose sugar contributes to its greater stability, making it ideal for long-term storage of genetic information.
Phosphate Group
The phosphate group is identical in both DNA and RNA nucleotides. It is a negatively charged group that links nucleotides together, forming the sugar-phosphate backbone of the nucleic acid strand. This backbone provides the structural framework for the molecule and is crucial for its overall stability.
Nitrogenous Base
The nitrogenous base is the component that distinguishes one nucleotide from another and encodes the actual genetic information. There are five primary nitrogenous bases found in nucleic acids: adenine (A), guanine (G), cytosine (C), thymine (T), and uracil (U). DNA utilizes adenine, guanine, cytosine, and thymine, while RNA uses adenine, guanine, cytosine, and uracil.
The specific pairing of these bases—adenine with thymine (or uracil in RNA) and guanine with cytosine—is fundamental to DNA's double helix structure and its ability to replicate accurately.
Polymerization: Linking Nucleotides Together
Nucleotides are linked together through a phosphodiester bond, formed between the phosphate group of one nucleotide and the sugar of the next. This process creates a long chain of nucleotides, forming a single strand of DNA or RNA.
In DNA, two such strands wind around each other in a double helix, held together by hydrogen bonds between the complementary base pairs. This double-stranded structure provides stability and allows for accurate replication of the genetic code. RNA, on the other hand, is typically single-stranded, allowing it to fold into complex three-dimensional structures that enable its diverse functions within the cell.
The sequence of nucleotides within a DNA or RNA strand dictates the genetic information it carries. This sequence is read and interpreted by the cellular machinery to synthesize proteins, regulate gene expression, and carry out the myriad processes that sustain life.
Dehydration Synthesis and Hydrolysis: Constructing and Deconstructing Life's Building Blocks
Now that we’ve explored the diverse monomers that serve as the foundational units of macromolecules – from the simple sugars that build carbohydrates to the nucleotides that encode our genetic information – it’s time to examine the processes that govern their assembly and disassembly. These two opposing forces, dehydration synthesis and hydrolysis, are essential for life, allowing organisms to build complex structures when needed and break them down for energy or to recycle their components.
Dehydration Synthesis: Building Polymers
Dehydration synthesis, also known as condensation, is the fundamental process by which monomers are covalently linked together to form polymers. The term "dehydration" aptly describes the reaction, as it involves the removal of a water molecule (H₂O) for each bond formed between two monomers.
Essentially, a hydroxyl group (-OH) is removed from one monomer, and a hydrogen atom (-H) is removed from the other. These combine to form water, while the remaining atoms on the monomers form a new covalent bond.
This process requires energy input, usually facilitated by enzymes, which act as catalysts to speed up the reaction.
How Dehydration Synthesis Works
Imagine linking two glucose molecules to form maltose, a disaccharide. In this process, one glucose molecule donates a hydroxyl group, and the other donates a hydrogen atom. The released water molecule is a byproduct, and a glycosidic bond is formed between the two glucose monomers, creating maltose. This same principle applies to the formation of all other macromolecules.
Amino acids are linked by peptide bonds to form polypeptides (proteins). Nucleotides are linked by phosphodiester bonds to form nucleic acids (DNA and RNA). Fatty acids are linked to glycerol by ester bonds to form triglycerides (fats).
Hydrolysis: Breaking Down Polymers
Hydrolysis is the reverse of dehydration synthesis. It’s the process by which polymers are broken down into their constituent monomers through the addition of a water molecule. The term "hydrolysis" literally means "to break with water."
In this reaction, a water molecule is split into a hydroxyl group (-OH) and a hydrogen atom (-H). The hydroxyl group attaches to one monomer, and the hydrogen atom attaches to the other, breaking the covalent bond that held them together.
The Role of Hydrolysis in Digestion
Hydrolysis is a crucial process in digestion. When we eat food, our bodies use enzymes to catalyze hydrolysis reactions, breaking down complex carbohydrates, proteins, and fats into smaller, absorbable monomers.
For example, the enzyme amylase breaks down starch (a polysaccharide) into glucose monomers. Proteases break down proteins into amino acids. Lipases break down triglycerides into fatty acids and glycerol.
These monomers are then absorbed into the bloodstream and used by the body for energy, building new molecules, or other essential functions.
Dehydration Synthesis and Hydrolysis: A Dynamic Equilibrium
Dehydration synthesis and hydrolysis are not merely opposing reactions, they are intertwined processes that maintain a dynamic equilibrium within living organisms. The balance between these two processes allows cells to constantly build, remodel, and break down macromolecules as needed.
This dynamic interplay is essential for growth, repair, and adaptation to changing environmental conditions. Understanding these fundamental processes is key to understanding the dynamic nature of life itself.
Video: Macromolecules: Unveiling Life's Tiny Building Blocks
FAQs: Macromolecules - Unveiling Life's Tiny Building Blocks
Here are some frequently asked questions about macromolecules and their crucial roles in living organisms. We'll clarify their basic components and functions.
What exactly are macromolecules?
Macromolecules are large, complex molecules essential for life. They're polymers, meaning they're built from repeating smaller units. Examples include proteins, carbohydrates, lipids (fats), and nucleic acids. Understanding what are the building blocks of macromolecules is key to understanding their function.
What are the primary functions of each type of macromolecule?
Proteins have diverse roles, from enzymes to structural support. Carbohydrates are primarily for energy. Lipids store energy and form cell membranes. Nucleic acids (DNA and RNA) store and transmit genetic information. These differing functions arise from what are the building blocks of macromolecules.
What are the building blocks of macromolecules, and what are they called?
The building blocks are called monomers. For proteins, they are amino acids. Carbohydrates are built from monosaccharides (simple sugars). Lipids are made of fatty acids and glycerol. Nucleic acids are composed of nucleotides. It’s crucial to know what are the building blocks of macromolecules.
How are macromolecules assembled from their building blocks?
Monomers join together through a process called dehydration synthesis (or condensation). This process removes a water molecule, forming a bond between the monomers and creating the larger polymer. Understanding how these polymers are made from what are the building blocks of macromolecules is fundamental to biology.