Global Mutation: What You Need to Know Now! #Mutation
The concept of gene flow significantly influences global mutation rates, altering allele frequencies across different populations. Population genetics, a core principle in evolutionary biology, provides frameworks for understanding these changes. Organizations like the World Health Organization (WHO) monitor mutations with potential public health implications. Furthermore, sophisticated bioinformatics tools are crucial for analyzing genomic data and tracking the spread of specific mutations, providing insights into global mutation phenomena and their impact on biodiversity and human health.
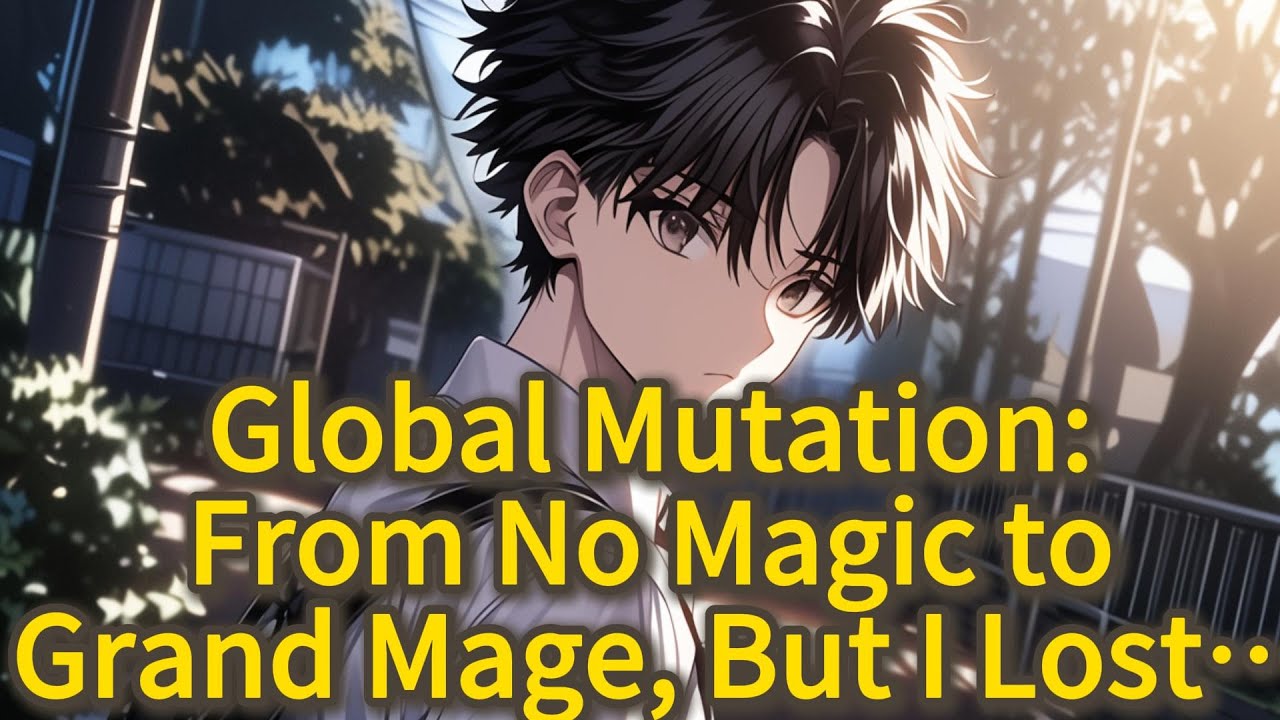
Image taken from the YouTube channel 06 Manhwa Recap , from the video titled Global Mutation: From No Magic to Grand Mage, But I Lost… .
The world of microorganisms, particularly viruses, is in a constant state of flux. This perpetual evolution, driven by mutation, has profound implications for global health, biosecurity, and our understanding of life itself.
Defining Global Mutation
Global mutation, in the context of rapidly evolving organisms like viruses, refers to the widespread and interconnected network of genetic changes occurring across the globe. It's not simply about isolated mutations in individual organisms.
It's about the broader patterns, trends, and consequences of these mutations at a planetary scale. It encompasses the ways in which viral genomes adapt and evolve in response to selective pressures across different geographical regions and populations.
This includes the emergence and spread of new variants, the development of drug resistance, and the potential for increased transmissibility or virulence.
The Urgency of Understanding
In our increasingly interconnected world, mutations in viruses and other microorganisms can rapidly traverse borders.
The COVID-19 pandemic starkly illustrated the speed and devastating impact of a globally spreading mutated virus. Understanding the dynamics of global mutation is no longer an academic exercise.
It is a critical imperative for protecting public health, developing effective countermeasures, and mitigating future pandemics. Monitoring, analyzing, and responding to these mutations in real time is essential for safeguarding global health security.
Tracking the Conversation with #Mutation
In the digital age, social media plays a significant role in disseminating information about emerging scientific trends. The hashtag #Mutation serves as a valuable tool for tracking discussions, updates, and insights related to global mutation.
It allows researchers, public health officials, and the general public to share information, collaborate on research, and raise awareness about the latest developments in the field.
By following this hashtag, one can gain access to a diverse range of perspectives and stay informed about the ongoing evolution of viruses and other microorganisms. This aids in understanding the global challenges and potential solutions associated with these mutations.
The urgency of tracking and understanding global mutation becomes clear when one considers the fundamental nature of these changes and the mechanisms driving them. To effectively address the challenges posed by evolving pathogens, a firm grasp of mutation basics is essential.
Mutation 101: Understanding the Fundamentals
At its core, a mutation represents a change in the genetic material of an organism. In the context of viruses, these changes can have significant consequences, influencing everything from transmissibility to virulence. Grasping the intricacies of viral mutation, mutation rates, and different types of mutations is crucial for anyone seeking to understand the dynamics of global mutation.
Viral Mutation Explained
Viral mutation is the process by which the genetic material of a virus undergoes alteration. Viruses, unlike organisms with DNA, often have RNA as their genetic material, which is inherently less stable.
This instability, coupled with the error-prone nature of viral replication, leads to a higher frequency of mutations.
These mutations can arise spontaneously during replication or be induced by external factors like exposure to radiation or certain chemicals. The resulting changes in the viral genome can then affect the virus's characteristics.
Decoding Mutation Rate
Mutation rate refers to the frequency at which mutations occur within an organism. Viruses are characterized by exceptionally high mutation rates compared to other life forms.
This is largely due to the lack of proofreading mechanisms during replication, which are present in more complex organisms.
The high mutation rate in viruses is a double-edged sword. On one hand, it allows them to rapidly adapt to new environments and evade immune responses.
On the other hand, many mutations are deleterious, rendering the virus non-functional. The balance between beneficial and harmful mutations ultimately determines the virus's evolutionary trajectory.
Types of Mutations and Their Impact
Mutations can be categorized into several types, each with its own potential consequences.
Point mutations involve changes to a single nucleotide base within the genetic sequence.
These can be further classified as substitutions, insertions, or deletions. Substitutions involve replacing one nucleotide with another, while insertions add a nucleotide, and deletions remove one.
Frameshift mutations, caused by insertions or deletions of nucleotides that are not multiples of three, are particularly disruptive.
They alter the reading frame of the genetic code, leading to a completely different protein sequence downstream of the mutation.
The impact of a mutation can range from negligible to catastrophic. Some mutations have no noticeable effect, particularly if they occur in non-coding regions of the genome.
However, mutations that alter the amino acid sequence of a viral protein can have profound effects on its function.
For example, a mutation in the receptor-binding domain of a virus can alter its ability to infect cells, while a mutation in an enzyme involved in replication can affect its rate of replication. Ultimately, the type and location of a mutation determine its impact on the virus and its ability to spread.
The high mutation rate in viruses is a double-edged sword. On one hand, it allows viruses to adapt quickly to changing environments, potentially leading to increased transmissibility or virulence. On the other hand, it can also lead to the emergence of non-viable mutations, effectively extinguishing viral lineages. Understanding these rates is just the beginning; we must also examine the forces that actively drive and shape these mutations on a global scale.
Driving Forces: What Fuels Global Mutation?
Several interconnected factors contribute to the increased mutation rates observed globally. These forces act as engines, accelerating the pace of viral evolution and shaping the landscape of emerging variants. Understanding these drivers is crucial for predicting future trends and developing effective mitigation strategies.
Factors Influencing Global Mutation Rates
Increased global mutation rates are not simply random occurrences. They are influenced by a complex interplay of factors, including:
-
Viral Replication Dynamics: The inherent properties of viral replication, including the error rate of viral polymerases, play a significant role. Viruses with less accurate replication machinery tend to exhibit higher mutation rates.
-
Host Immune Response: The pressure exerted by the host's immune system can drive the selection of mutations that allow the virus to evade immune recognition. This constant arms race between the virus and the host fuels ongoing evolution.
-
Antiviral Therapies: The use of antiviral drugs can also exert selective pressure on viral populations, leading to the emergence of drug-resistant mutations. Understanding the mechanisms of resistance is essential for developing next-generation therapeutics.
-
Population Immunity: Widespread immunity, whether through vaccination or prior infection, can similarly drive viral evolution. As the virus encounters a population with pre-existing immunity, it must adapt to overcome these defenses.
Selective Pressure: The Guiding Hand of Evolution
Selective pressure refers to environmental factors that influence the survival and reproduction of organisms with specific traits. In the context of viruses, these pressures can be exerted by a variety of sources, including the host immune system, antiviral drugs, and even environmental conditions.
Mutations that confer a selective advantage, such as increased transmissibility or resistance to antiviral drugs, are more likely to persist and spread within a population. This process of natural selection drives the evolution of viruses towards greater fitness in their specific environment.
Understanding the specific selective pressures acting on a virus is crucial for predicting its evolutionary trajectory and developing effective control measures.
Viral Evolution and the Emergence of Variants of Concern (VOCs)
Viral evolution is the process by which viruses change over time, driven by mutation and natural selection. This process can lead to the emergence of new variants with altered characteristics, such as increased transmissibility, virulence, or immune evasion. When these variants pose a significant threat to public health, they are designated as Variants of Concern (VOCs).
The emergence of VOCs is a complex process that depends on a variety of factors, including the mutation rate of the virus, the selective pressures acting on the virus, and the size and connectivity of the susceptible population.
The evolutionary pathways that lead to VOC emergence often involve:
-
Accumulation of Mutations: The gradual accumulation of mutations over time can lead to significant changes in viral phenotype.
-
Recombination: The exchange of genetic material between different viral strains can create novel combinations of mutations, potentially leading to the emergence of new VOCs.
-
Adaptation to New Hosts: Viruses that jump to new host species may undergo rapid evolution to adapt to their new environment, potentially leading to the emergence of VOCs with altered host tropism.
GISAID: A Global Resource for Tracking Viral Evolution
GISAID (Global Initiative on Sharing All Influenza Data) plays a crucial role in tracking and understanding viral evolution. This global science initiative promotes the rapid sharing of genomic data from influenza viruses and SARS-CoV-2.
By providing a centralized repository for viral sequence data, GISAID enables researchers around the world to:
-
Monitor the emergence and spread of new variants: Real-time tracking of viral sequences allows for the early detection of emerging VOCs.
-
Identify mutations of concern: Analyzing viral genomes can reveal mutations that may affect transmissibility, virulence, or immune evasion.
-
Develop and evaluate diagnostic tests and vaccines: Access to comprehensive viral sequence data is essential for developing effective diagnostic tools and vaccines.
-
Inform public health responses: Data from GISAID informs public health decision-making, allowing for targeted interventions to control the spread of emerging variants.
Genomic Surveillance: Real-Time Mutation Tracking
The relentless march of viral evolution demands constant vigilance. We’ve seen how various factors can accelerate mutation rates, leading to a dynamic landscape of viral variants. But how do we actually see these changes as they emerge and spread across the globe? The answer lies in genomic surveillance.
Genomic surveillance acts as an early warning system, providing the data needed to understand and respond to evolving threats.
The Power of Early Detection
Genomic surveillance is the systematic collection and analysis of genetic material from pathogens to identify and monitor mutations. It's a critical tool for understanding how viruses are changing, where they are spreading, and how these changes might affect public health.
Without robust surveillance, new variants could spread undetected, potentially undermining the effectiveness of vaccines, treatments, and diagnostic tests.
Next-Generation Sequencing: Unveiling the Viral Code
At the heart of genomic surveillance lies next-generation sequencing (NGS) and other advanced genome sequencing technologies. These tools allow scientists to rapidly and accurately decipher the complete genetic code of viral samples collected from infected individuals or environmental sources.
NGS has revolutionized the field by dramatically increasing the speed and decreasing the cost of sequencing. This allows for the analysis of a much larger number of samples, providing a more comprehensive view of viral diversity and evolution.
How NGS Works
NGS technologies work by fragmenting DNA or RNA into small pieces, sequencing these fragments, and then using sophisticated algorithms to assemble the complete genome. This process can be done in parallel on millions of fragments, allowing for high-throughput sequencing.
The resulting sequence data is then compared to reference genomes to identify any mutations or variations.
Informing Public Health Responses
The data generated through genomic surveillance is not just an academic exercise; it directly informs public health responses and interventions.
By tracking the emergence and spread of new variants, public health officials can make informed decisions about:
- Implementing targeted interventions: Identifying hotspots of variant transmission can allow for focused interventions, such as increased testing, vaccination efforts, or public health messaging.
- Evaluating the effectiveness of existing measures: Genomic data can help determine if current vaccines or treatments are effective against emerging variants.
- Developing new countermeasures: Understanding the genetic makeup of new variants can guide the development of new vaccines, antiviral drugs, and diagnostic tests.
- Informing travel advisories: Tracking the global distribution of variants can help inform travel advisories and border control measures.
Effective public health responses require accurate and timely data, and genomic surveillance provides just that.
Challenges and Future Directions
While genomic surveillance has proven to be an invaluable tool, there are challenges to overcome. These include:
- Data sharing: Ensuring that genomic data is shared rapidly and openly across international borders is crucial for effective global surveillance.
- Capacity building: Many countries lack the resources and infrastructure to conduct comprehensive genomic surveillance.
- Data analysis: The massive amount of data generated by NGS requires sophisticated analytical tools and expertise.
Overcoming these challenges will require sustained investment, international collaboration, and continued innovation in sequencing and data analysis technologies. The future of global health security depends on our ability to stay one step ahead of evolving pathogens.
Global Health Impact: Consequences of Mutation
The data gleaned from genomic surveillance, while invaluable for understanding the evolutionary trajectory of pathogens, ultimately serves a more critical purpose: mitigating the impact of mutations on global public health. The emergence and spread of mutations, particularly in viruses and bacteria, pose substantial challenges to treatment strategies, vaccine efficacy, and overall disease control efforts.
The Rise of Drug-Resistant Pathogens
One of the most concerning consequences of mutation is the development of drug resistance. Pathogens, through the accumulation of genetic changes, can evolve mechanisms to evade the effects of antimicrobial drugs.
This phenomenon is observed across a wide range of infectious agents, including bacteria, viruses, fungi, and parasites. For example, the rise of antibiotic-resistant bacteria, such as methicillin-resistant Staphylococcus aureus (MRSA) and carbapenem-resistant Enterobacteriaceae (CRE), represents a significant threat to modern medicine.
Similarly, mutations in viruses like HIV and influenza can lead to resistance to antiviral medications, necessitating the development of new drugs and treatment strategies. The process of drug resistance underscores the dynamic interplay between pathogen evolution and human interventions.
Implications for Vaccine Development and Efficacy
Mutations also have profound implications for vaccine development and efficacy. Vaccines work by stimulating the immune system to recognize and mount a defense against specific antigens, typically proteins on the surface of a pathogen.
However, if these antigens undergo significant changes due to mutation, the effectiveness of the vaccine may be compromised. This is particularly relevant for viruses with high mutation rates, such as influenza and SARS-CoV-2.
The emergence of new variants of concern (VOCs) for SARS-CoV-2, for example, has raised concerns about the ability of existing vaccines to provide adequate protection. While current vaccines still offer some level of protection against severe disease, hospitalization, and death, their efficacy against infection may be reduced for certain variants.
This necessitates ongoing monitoring of viral evolution and, potentially, the development of updated vaccines that target emerging variants.
The Role of Global Health Organizations
Combating the global health threats posed by mutations requires a coordinated and collaborative effort involving international organizations, governments, and research institutions. The World Health Organization (WHO) and the Centers for Disease Control and Prevention (CDC) play critical roles in this effort.
The WHO is responsible for coordinating international health within the United Nations system. It provides guidance and technical support to countries on a wide range of health issues, including infectious disease surveillance, prevention, and control.
The CDC, as the leading national public health institute of the United States, conducts research, monitors disease trends, and provides recommendations for preventing and controlling infectious diseases.
Both organizations work closely with other international partners to track the emergence and spread of mutations, assess their potential impact on public health, and develop strategies to mitigate their effects. They also play a key role in communicating risks to the public and promoting evidence-based interventions.
Hotspots of Mutation: A Global Perspective
The emergence of variants of concern is not a geographically uniform phenomenon. Certain regions have emerged as hotspots for viral evolution and the generation of new variants.
South Africa, for example, was the origin of the Beta variant and played a significant role in the early detection of Omicron. The United Kingdom identified and characterized the Alpha variant. India saw the rise of the Delta variant. Brazil was an area of concern with the Gamma variant.
Factors such as high infection rates, limited access to vaccines, and the presence of immunocompromised individuals may contribute to the accelerated evolution of viruses in these regions. Understanding the factors that drive viral evolution in specific geographic locations is crucial for implementing targeted interventions and preventing the global spread of new variants. These locations underscore the importance of global cooperation in monitoring and responding to emerging health threats.
The implications of mutations are clear: They challenge existing treatments, undermine vaccine efficacy, and necessitate constant vigilance. But how do scientists actually find these mutations in the first place? The answer lies, in large part, with a technique that has become a cornerstone of molecular biology: Polymerase Chain Reaction, or PCR.
PCR's Role: Identifying and Tracking Mutations
Polymerase Chain Reaction (PCR) is a revolutionary molecular biology technique that allows scientists to amplify specific DNA sequences, creating millions or even billions of copies from a tiny starting sample. This amplification process is essential for identifying and tracking mutations, as it enables researchers to analyze genetic material even when it's present in very low concentrations.
The Power of Amplification
PCR's primary function is to amplify specific regions of DNA. This is achieved through a cyclical process involving denaturation, annealing, and extension, using a DNA polymerase enzyme.
By selectively amplifying target sequences, PCR makes it possible to detect even rare mutations within a complex genetic background. This is crucial in situations where the mutated sequence represents only a small fraction of the total genetic material.
PCR-Based Mutation Detection Methods
Several PCR-based methods are employed to detect and characterize mutations. These include:
-
Real-time PCR (qPCR): qPCR allows for the quantification of DNA amplification in real-time. This can be used to detect the presence of specific mutations by designing primers that selectively bind to either the wild-type or mutant sequence. The presence and abundance of the mutant sequence can then be determined by monitoring the fluorescence signal during PCR amplification.
-
Reverse Transcription PCR (RT-PCR): For RNA viruses, such as SARS-CoV-2 and influenza, RT-PCR is used to convert the viral RNA into DNA, which can then be amplified and analyzed using PCR. This is essential for detecting mutations in RNA viruses and tracking their evolution.
-
Allele-Specific PCR: This technique uses primers that are specifically designed to bind to either the wild-type or mutant allele. By using different primer sets, researchers can determine whether a sample contains the wild-type allele, the mutant allele, or both.
-
High-Resolution Melting (HRM) Analysis: HRM is a post-PCR technique that analyzes the melting behavior of DNA fragments. Even subtle sequence differences, such as single nucleotide polymorphisms (SNPs), can alter the melting profile, allowing for the identification of mutations.
PCR in Surveillance and Research
PCR plays a central role in genomic surveillance efforts, enabling the rapid detection and characterization of emerging mutations in pathogens.
Public health agencies and research institutions use PCR to:
-
Monitor the spread of variants of concern (VOCs): By designing PCR assays that target specific mutations associated with VOCs, public health agencies can track the prevalence and geographic distribution of these variants.
-
Assess the impact of mutations on diagnostic tests: Mutations can affect the accuracy of diagnostic tests, particularly PCR-based assays. PCR is used to identify mutations that may interfere with primer binding or probe hybridization, leading to false-negative results.
-
Investigate the mechanisms of drug resistance: PCR is used to identify mutations that confer drug resistance in pathogens. This information is crucial for developing new drugs and treatment strategies that can overcome resistance.
-
Develop and validate new diagnostic assays: PCR is used to develop and validate new diagnostic assays that can detect emerging mutations in pathogens. This is essential for ensuring that diagnostic tests remain accurate and effective as pathogens evolve.
PCR, therefore, is far more than just a lab technique. It's a critical tool that empowers scientists and public health officials to stay one step ahead in the ever-evolving landscape of global mutations. Without it, our ability to detect, track, and respond to emerging threats would be significantly diminished.
Video: Global Mutation: What You Need to Know Now! #Mutation
Global Mutation: Frequently Asked Questions
Here are some common questions about global mutation and its implications. We hope this clarifies any confusion and provides a better understanding of this important topic.
What exactly is global mutation?
Global mutation refers to changes in DNA that occur across a wide range of organisms or even the entire biosphere. It signifies that the rate or pattern of genetic alterations is shifting on a large scale, potentially due to environmental factors or selection pressures. This is more than just isolated mutations in a single species.
What could cause a surge in global mutation rates?
Increased exposure to mutagens (radiation, chemicals), changes in the effectiveness of DNA repair mechanisms, or new evolutionary pressures can drive higher rates of global mutation. Human activities such as pollution and habitat destruction can also indirectly contribute.
Are all global mutations harmful?
No, not all global mutations are inherently harmful. Some mutations can be neutral, meaning they don't have a noticeable effect. Others might even be beneficial in certain environments, allowing organisms to adapt to changing conditions.
What are the potential long-term consequences of global mutation?
The long-term effects are hard to predict precisely. However, increased global mutation could accelerate evolution, leading to new species or significant changes in existing ones. It could also increase the risk of diseases or disrupt ecosystems if harmful mutations become widespread.
Alright, that's the lowdown on global mutation! Hopefully, you've got a better grasp of what's happening. Keep an eye on the science, and who knows, maybe you'll even contribute to understanding it someday! Cheers!