Ester Group IR: Decoding Spectra Like a Pro!🔥
Infrared spectroscopy, a crucial analytical technique, provides insights into molecular vibrations. Ester group IR spectral analysis relies heavily on characteristic absorption bands. These distinct peaks are identifiable using reference databases like the NIST Chemistry WebBook. Successful interpretation often involves correlating the IR data with information from mass spectrometry, a complementary technique for molecular identification. Understanding these relationships requires familiarity with established spectroscopic resources, such as those offered by institutions like the Coblentz Society. This allows for definitive determination of ester group ir peaks.
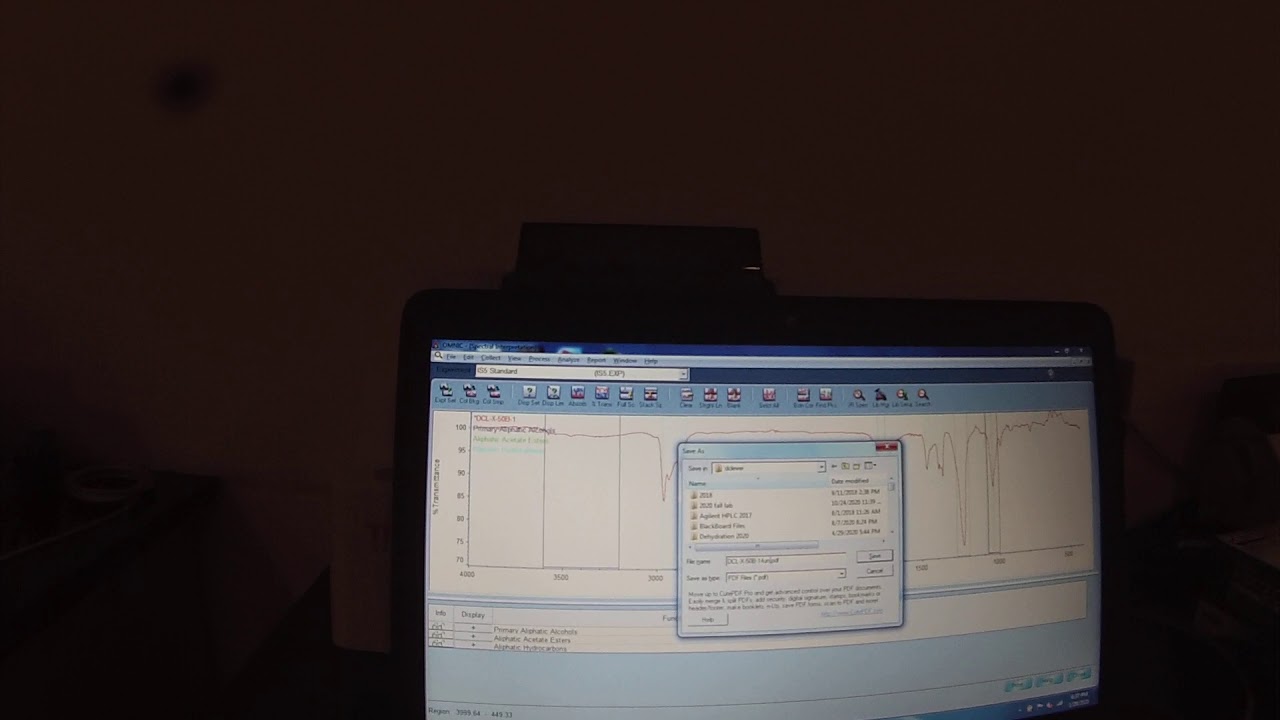
Image taken from the YouTube channel Chemistry 261 Lab , from the video titled Ester IR spectrum .
Ester groups, ubiquitous in the realm of chemistry, play a pivotal role in shaping the characteristics of numerous compounds. From the tantalizing aromas of fruits to the structural integrity of polymers, esters are fundamental building blocks. Infrared (IR) spectroscopy emerges as an indispensable analytical technique for not only identifying but also meticulously characterizing these ester functionalities.
The Pervasive Presence of Ester Groups
Ester linkages (R-COOR') are prevalent throughout various chemical domains, impacting our daily lives in countless ways. They are the cornerstone of many natural and synthetic compounds.
-
Flavors and Fragrances: Esters are largely responsible for the pleasant scents and tastes of many fruits and flowers. For example, ethyl acetate contributes to the aroma of some wines, and butyl acetate gives apples their characteristic scent.
-
Polymers: Many polymers, such as polyesters (e.g., PET used in plastic bottles and clothing) and polyacrylates, are built upon ester linkages. These impart specific properties like flexibility, durability, and chemical resistance.
-
Pharmaceuticals: Ester functionalities are also found in several pharmaceuticals, where they can play roles in the drug's mechanism, stability, or bioavailability.
IR Spectroscopy: A Window into Molecular Vibrations
IR spectroscopy provides a unique "fingerprint" of a molecule based on its vibrational modes.
When a molecule absorbs infrared radiation, specific bonds within the molecule vibrate at characteristic frequencies. These vibrations are dependent on the bond strength and the masses of the atoms involved. The resulting absorption spectrum, a plot of absorbance or transmittance versus wavenumber, reveals the presence of specific functional groups.
For esters, the carbonyl (C=O) and carbon-oxygen (C-O) stretches are particularly informative. By analyzing the positions, intensities, and shapes of these absorption bands, a trained spectroscopist can confidently identify and characterize ester groups within a sample.
Empowering Your Spectral Interpretation Skills
This article aims to equip readers with the knowledge and skills necessary to confidently interpret IR spectra containing ester group signatures. We will delve into the key absorption bands, factors influencing their positions, and strategies for differentiating esters from other functional groups. By mastering these concepts, you will be empowered to "decode spectra like a pro!" and leverage the power of IR spectroscopy for your research, analysis, or quality control endeavors.
Ester linkages (R-COOR') are prevalent throughout various chemical domains, impacting our daily lives in countless ways. They are the cornerstone of many natural and synthetic compounds.
Therefore, gaining a deeper understanding of the interaction of IR radiation with molecular bonds is imperative to effectively analyze ester groups. This section elucidates the core principles of IR spectroscopy, providing a foundation for interpreting the spectral data.
Understanding the Fundamentals of Infrared (IR) Spectroscopy
Infrared (IR) spectroscopy is a powerful analytical technique used to identify and characterize molecules based on their vibrational modes.
It works by measuring the absorption of infrared radiation by a sample. The resulting spectrum provides a unique "fingerprint" of the molecule, revealing valuable information about its structure and composition.
Molecular Vibrations and Infrared Absorption
At the heart of IR spectroscopy lies the interaction between infrared radiation and the vibrational modes of molecules. Molecules are not static entities. Their atoms are constantly vibrating around their equilibrium positions. These vibrations occur at specific frequencies that are determined by the bond strength and the masses of the atoms involved.
When a molecule is exposed to infrared radiation, it can absorb energy if the frequency of the radiation matches the frequency of a particular vibrational mode.
This absorption causes the amplitude of the vibration to increase. Only vibrations that cause a change in the dipole moment of the molecule are IR active and will result in absorption. Symmetric molecules or vibrations may not always be observed.
Key Concepts in IR Spectroscopy
Several key concepts are essential for understanding and interpreting IR spectra:
Wavenumber (cm-1)
Wavenumber is the reciprocal of the wavelength, typically expressed in units of inverse centimeters (cm-1).
It is directly proportional to the frequency of the vibration and, therefore, to the energy of the absorbed radiation. Wavenumber is the most common unit used to represent the position of absorption bands in an IR spectrum. Higher wavenumbers correspond to higher energy vibrations.
Absorption Bands
Absorption bands are regions in the IR spectrum where the molecule absorbs infrared radiation. Each band corresponds to a specific vibrational mode within the molecule.
The position, intensity, and shape of an absorption band provide valuable information about the functional groups present and the molecular environment. The intensity of the band is proportional to the change in dipole moment during the vibration.
Transmittance vs. Absorbance
IR spectra are typically presented as either transmittance or absorbance versus wavenumber.
-
Transmittance is the fraction of incident radiation that passes through the sample. It is expressed as a percentage (%). Low transmittance indicates strong absorption.
-
Absorbance is the measure of the amount of light absorbed by the sample. It is logarithmically related to transmittance. High absorbance indicates strong absorption. Absorbance is often preferred for quantitative analysis due to its linear relationship with concentration (Beer-Lambert Law).
Fourier Transform Infrared (FTIR) Spectroscopy
Fourier Transform Infrared (FTIR) spectroscopy is a modern and widely used technique that offers significant advantages over traditional dispersive IR spectroscopy.
Instead of using a monochromator to select specific wavelengths of infrared radiation, FTIR uses an interferometer. The interferometer simultaneously measures all frequencies of infrared radiation. A mathematical process called Fourier transformation is then used to convert the data into a conventional IR spectrum.
FTIR offers several advantages, including:
- Higher sensitivity: FTIR instruments can collect data much faster than traditional IR instruments, allowing for the analysis of smaller samples.
- Improved accuracy: FTIR instruments are less susceptible to stray light and other sources of error, resulting in more accurate spectra.
- Better resolution: FTIR instruments can achieve higher spectral resolution, allowing for the separation of closely spaced absorption bands.
Understanding these fundamental principles of IR spectroscopy is crucial for effectively interpreting IR spectra and extracting valuable information about molecular structure and composition. The next section will delve into the specific IR signatures of ester groups, building upon this foundational knowledge.
Ester linkages (R-COOR') are prevalent throughout various chemical domains, impacting our daily lives in countless ways. They are the cornerstone of many natural and synthetic compounds.
Therefore, gaining a deeper understanding of the interaction of IR radiation with molecular bonds is imperative to effectively analyze ester groups. This section elucidates the core principles of IR spectroscopy, providing a foundation for interpreting the spectral data.
The Ester Group: Structure and Characteristic IR Signatures
Having established the fundamental principles of IR spectroscopy, the next logical step is to delve into the specifics of the ester group. We will explore its unique chemical structure and correlate it with the characteristic absorption bands observed in an IR spectrum. Furthermore, we will examine factors that can influence the position and intensity of these bands, adding another layer of nuance to spectral interpretation.
Understanding the Chemical Structure of an Ester Group (R-COOR')
At its core, an ester group is characterized by a carbon atom double-bonded to an oxygen atom (C=O), and singly bonded to another oxygen atom, which is in turn bonded to an alkyl or aryl group. This arrangement can be represented as R-COOR', where R and R' represent alkyl or aryl groups.
The presence of two oxygen atoms directly bonded to the carbonyl carbon imparts unique electronic properties to the ester functional group. This arrangement also influences the vibrational modes and, consequently, its IR spectrum.
Characteristic Absorption Bands of Ester Groups
The IR spectrum of an ester exhibits several characteristic absorption bands that are invaluable for identification. These bands arise from the vibrational modes of specific bonds within the ester group. The most prominent bands are associated with the C=O and C-O stretches.
The Carbonyl (C=O) Stretch
The carbonyl stretch is arguably the most important and easily identifiable band in the ester IR spectrum.
-
Location: This strong absorption typically appears in the region of 1750-1735 cm-1 for aliphatic esters.
-
Intensity: The C=O stretch is usually very intense, making it a reliable indicator of the presence of an ester group.
-
Factors Affecting Position: Several factors can influence the precise location of the C=O stretch.
- Conjugation: Conjugation with an adjacent double bond or aromatic ring lowers the frequency by about 20-40 cm-1.
- Ring Strain: In cyclic esters (lactones), the carbonyl frequency increases as ring size decreases due to increased ring strain.
- Inductive Effects: Electron-withdrawing groups near the carbonyl carbon can increase the frequency.
The C-O Stretch
Ester groups exhibit two distinct C-O stretching bands due to the presence of two different C-O bonds.
-
These bands typically appear in the region of 1300-1000 cm-1.
-
The band at higher wavenumber is usually attributed to the C-O stretch adjacent to the carbonyl group (C-O-C=O). The other band is from the other C-O bond (O-CH2-R).
-
The intensities of these bands can vary, and their precise positions are sensitive to the structure of the ester.
-
The presence of two strong absorptions in this region is further compelling evidence for the presence of an ester.
Other Relevant Bands
While the C=O and C-O stretches are the most characteristic, other bands can provide additional information.
-
C-H stretches: These bands appear below 3000 cm-1. Their positions and intensities depend on the nature of the alkyl or aryl groups attached to the ester.
-
O-H stretches: The absence of a broad O-H stretch around 3300 cm-1 helps differentiate esters from carboxylic acids.
Factors Affecting Peak Positions and Intensities
The precise locations and intensities of the characteristic ester absorption bands are not static; they are influenced by several factors:
-
Inductive Effects: Electron-withdrawing groups can shift the C=O stretch to higher wavenumbers, while electron-donating groups have the opposite effect.
-
Resonance/Mesomeric Effects: For example, the carbonyl C=O stretching frequency decreases when the ester group is conjugated with an alkene or aromatic ring, due to resonance and the single bond character increases.
-
Conjugation: As mentioned earlier, conjugation lowers the frequency of the C=O stretch.
-
Hydrogen Bonding: While esters are not strong hydrogen bond donors, intermolecular hydrogen bonding can still affect the band positions and shapes, particularly in concentrated samples.
Understanding these factors allows for a more nuanced interpretation of ester IR spectra, enabling the differentiation of various ester subclasses and providing insights into their molecular environment.
Step-by-Step Guide to Interpreting Ester Group IR Spectra
Having established the fundamental principles of IR spectroscopy, the next logical step is to delve into the specifics of the ester group. We will explore its unique chemical structure and correlate it with the characteristic absorption bands observed in an IR spectrum. Furthermore, we will examine factors that can influence the position and intensity of these bands, adding another layer of nuance to spectral interpretation.
The ability to interpret IR spectra effectively is crucial for identifying and characterizing chemical compounds. This section provides a practical, step-by-step guide to help you confidently identify ester groups in IR spectra and distinguish them from other functional groups.
A Systematic Approach to Spectra Interpretation
Interpreting an IR spectrum can seem daunting at first, but a systematic approach simplifies the process significantly.
-
Examine the Overall Spectrum: Begin by taking a broad view of the entire spectrum. Look for the most prominent peaks and note their positions (wavenumbers) and intensities.
-
Identify Key Functional Group Regions: Divide the spectrum into regions associated with specific functional groups. The region between 4000 cm-1 and 1500 cm-1 is typically associated with stretching vibrations, while the region below 1500 cm-1, known as the fingerprint region, is more complex and unique to each molecule.
-
Focus on the Carbonyl Region: The carbonyl (C=O) region, around 1800-1650 cm-1, is a critical area for identifying esters and other carbonyl-containing compounds.
-
Analyze Specific Absorption Bands: Once you've identified a potential functional group, examine the specific absorption bands associated with it. Consider their positions, intensities, and shapes.
-
Consult Reference Spectra and Databases: Compare your spectrum with reference spectra and databases to confirm your assignments.
Identifying the Ester Group: Key Absorption Bands
The presence of an ester group can be confirmed by identifying its characteristic absorption bands:
-
C=O Stretch: This is the most prominent band for esters, typically appearing in the range of 1750-1735 cm-1 for aliphatic esters. Aromatic esters and esters with conjugation may exhibit slightly lower wavenumbers. The intensity of this band is usually strong.
-
C-O Stretch: Esters exhibit two distinct C-O stretching bands. One typically appears between 1300-1100 cm-1, and another weaker band appears around 1000 cm-1. These bands arise from the C-O bond adjacent to the carbonyl group and the C-O bond attached to the alkyl/aryl group (R').
-
Other Relevant Bands: While the C=O and C-O stretches are the most diagnostic, other bands, such as C-H stretches (around 3000-2850 cm-1), can provide additional information about the ester's structure.
Distinguishing Esters from Other Functional Groups
Differentiating esters from other carbonyl-containing compounds is a crucial step in spectral interpretation. Consider the following:
Esters vs. Carboxylic Acids
- Carboxylic acids exhibit a broad O-H stretch in the range of 3300-2500 cm-1 due to hydrogen bonding, which is absent in esters. Also, carboxylic acids typically display a C=O stretch at slightly lower wavenumbers (around 1725-1700 cm-1) compared to esters.
Esters vs. Ketones
- Ketones generally have a single strong C=O absorption. While the location can be similar to esters (around 1715 cm-1), ketones lack the two characteristic C-O stretching bands found in esters.
Esters vs. Aldehydes
- Aldehydes exhibit a C=O stretch similar to ketones and esters. However, they also display a distinctive pair of C-H stretches around 2850 and 2750 cm-1, which are not present in esters.
ATR (Attenuated Total Reflectance) for Sample Analysis
Attenuated Total Reflectance (ATR) is a sampling technique used in IR spectroscopy that simplifies the analysis of solid and liquid samples. Instead of transmitting the IR beam through the sample, ATR uses an internal reflection element (crystal) to create an evanescent wave that interacts with the sample surface.
This technique is particularly useful for samples that are difficult to prepare for transmission IR, such as:
-
Opaque Solids: ATR allows for the direct analysis of solids without requiring them to be dissolved or finely ground.
-
Liquids: A small drop of liquid can be placed directly onto the ATR crystal for analysis.
-
Films and Coatings: ATR is ideal for analyzing thin films and coatings on various substrates.
By simplifying sample preparation and analysis, ATR enhances the accessibility and versatility of IR spectroscopy, making it a valuable tool for identifying and characterizing ester groups and other functional groups in a wide range of materials.
Having mastered the art of identifying ester groups through their primary IR signatures, it's time to consider more advanced techniques and subtle influences that can affect spectral interpretation. These nuances are critical for accurate analysis, especially when dealing with complex mixtures or subtle structural variations.
Advanced Techniques and Considerations in IR Spectroscopy
While characteristic absorption bands provide a solid foundation for identifying functional groups, relying solely on these can sometimes lead to misinterpretations. Several factors, including the chemical environment and the physical state of the sample, can significantly influence the appearance of an IR spectrum. Advanced techniques, coupled with careful consideration of these influencing factors, are essential for a comprehensive and reliable analysis.
The Indispensable Role of Reference Spectra and Databases
Spectral interpretation is rarely performed in isolation. Comparing an obtained spectrum against known standards is a crucial step in confirming peak assignments and identifying unknown compounds. Thankfully, readily accessible reference spectra and databases offer a wealth of information.
Exploring Key Spectral Databases
-
NIST WebBook: This invaluable resource, maintained by the National Institute of Standards and Technology (NIST), offers a vast collection of reference spectra for a wide range of compounds.
It's an excellent starting point for verifying peak positions and intensities.
-
SDBS (Spectral Database for Organic Compounds): Maintained by the National Institute of Advanced Industrial Science and Technology (AIST) in Japan, SDBS provides access to IR, NMR, and mass spectra.
This database is particularly useful for identifying organic compounds.
By comparing your sample's spectrum with these reference spectra, you can confidently confirm the presence of specific functional groups and identify potential contaminants.
Unraveling the Effects of Intermolecular Interactions
Molecules don't exist in a vacuum; they interact with their neighbors. These intermolecular interactions, particularly hydrogen bonding, can significantly alter the vibrational frequencies of certain bonds, resulting in shifts in absorption bands.
Hydrogen Bonding and Spectral Shifts
-
Hydrogen bonding is a strong intermolecular force that occurs between a hydrogen atom bonded to a highly electronegative atom (like oxygen) and another electronegative atom.
In esters, hydrogen bonding can occur between the carbonyl oxygen and protic solvents or impurities.
-
This interaction weakens the C=O bond, leading to a decrease in the wavenumber of the carbonyl stretching band.
The band may also broaden due to the varying strengths of hydrogen bonds.
Understanding the potential for hydrogen bonding and other intermolecular interactions is crucial for accurately interpreting the IR spectra of esters, especially in solution or in the presence of protic impurities.
Beyond qualitative identification, IR spectroscopy can also be used for quantitative analysis, determining the concentration of a specific compound in a sample. This relies on the principle that the absorbance of a compound is directly proportional to its concentration, as described by the Beer-Lambert Law.
The Beer-Lambert Law in Action
-
The Beer-Lambert Law states that absorbance (A) is equal to the product of the molar absorptivity (ε), the path length (b), and the concentration (c): A = εbc.
-
By measuring the absorbance of a specific absorption band and knowing the molar absorptivity and path length, the concentration of the analyte can be determined.
Considerations for Accurate Quantitative Analysis
-
Careful calibration is essential to establish a reliable relationship between absorbance and concentration.
-
The choice of the absorption band is also crucial; it should be well-resolved and free from interference from other compounds in the sample.
While quantitative IR analysis may not be as precise as other spectroscopic techniques like GC-MS, it offers a rapid and convenient method for estimating concentrations in certain applications.
By comparing your sample's spectrum with these reference spectra, you gain a much deeper understanding of its composition and structure. Now, let's shift our focus to how this knowledge translates into tangible, real-world applications.
Real-World Applications of Ester Group IR Spectroscopy
The ability to identify and characterize ester groups via IR spectroscopy extends far beyond the laboratory. This technique finds widespread use across diverse fields, from cutting-edge research to rigorous industrial quality control. Its versatility stems from the ubiquitous nature of esters, which play crucial roles in various chemical processes and product formulations.
Ester Group IR in Research Environments
In research settings, IR spectroscopy serves as a powerful tool for reaction monitoring. By tracking the appearance or disappearance of characteristic ester absorption bands, scientists can gain real-time insights into reaction kinetics and mechanisms.
For example, consider the synthesis of a polyester. Monitoring the decrease in the carboxylic acid peak and the simultaneous increase in the ester carbonyl peak allows researchers to optimize reaction conditions, such as temperature and catalyst concentration, to maximize yield and minimize unwanted byproducts.
Furthermore, IR spectroscopy is invaluable for characterizing novel ester-containing compounds. When synthesizing new molecules with ester functionalities, IR provides immediate confirmation of the presence of the ester group. This structural confirmation is critical for verifying the success of the synthesis and for further investigating the compound's properties.
Industrial Applications of Ester Group IR Spectroscopy
The industrial applications of ester group IR spectroscopy are extensive, particularly in industries dealing with polymers, flavors, and fragrances.
Polymer Characterization
Polymers containing ester linkages, such as polyesters and polyacrylates, are widely used in textiles, packaging, and coatings. IR spectroscopy is a critical technique for characterizing these polymers.
By analyzing the ester carbonyl and C-O stretching bands, manufacturers can determine the polymer's composition, molecular weight, and degree of cross-linking. This information is essential for ensuring that the polymer meets the required performance specifications.
Changes in the ester bands can also indicate polymer degradation due to heat, light, or chemical exposure, enabling early detection of potential material failures.
Quality Control of Flavors and Fragrances
Esters are key components of many flavors and fragrances, contributing to their characteristic aromas and tastes. IR spectroscopy plays a vital role in quality control within these industries.
By comparing the IR spectrum of a raw material or finished product to a reference standard, manufacturers can verify its identity and purity. Any deviations from the expected spectrum can indicate the presence of contaminants or degradation products, leading to rejection of the batch.
Quantitative analysis using IR spectroscopy can also be employed to determine the concentration of specific ester compounds in a formulation, ensuring batch-to-batch consistency and adherence to regulatory standards.
In summary, the diverse applications of ester group IR spectroscopy highlight its importance as an analytical technique. From fundamental research to industrial quality control, IR spectroscopy provides valuable insights into the structure, composition, and behavior of ester-containing materials.
Video: Ester Group IR: Decoding Spectra Like a Pro!🔥
FAQ: Decoding Ester Group IR Spectra
Here are some frequently asked questions about interpreting IR spectra to identify ester groups.
What key peaks should I look for to identify an ester group in an IR spectrum?
The most important peaks for identifying an ester group in IR spectroscopy are a strong C=O stretch around 1750-1735 cm⁻¹, a C-O stretch between 1300-1000 cm⁻¹, and often, a weaker C-O stretch in a different region within 1300-1000 cm⁻¹. The exact positions will vary slightly depending on the specific ester.
How can I differentiate between an ester and a carboxylic acid using IR spectroscopy?
Both esters and carboxylic acids show a strong C=O stretch. However, carboxylic acids exhibit a broad O-H stretch around 2500-3300 cm⁻¹ due to hydrogen bonding. Esters lack this broad O-H stretch, making it a key distinguishing factor. The absence of that broad peak indicates the presence of the ester group ir spectrum, not a carboxylic acid.
Why does the C=O stretch for an ester group sometimes shift to lower or higher wavenumbers?
The position of the C=O stretch in an ester group ir spectrum is sensitive to neighboring substituents. Electron-withdrawing groups increase the wavenumber, while conjugation with a double bond decreases the wavenumber. Ring strain in cyclic esters also increases the wavenumber of the C=O stretch.
Can IR spectroscopy alone definitively identify an ester?
While IR spectroscopy provides strong evidence for the presence of an ester group, it's often best used in conjunction with other spectroscopic techniques like NMR and mass spectrometry for definitive identification. IR is great for functional group identification, but NMR helps confirm the overall structure, and Mass Spec gives molecular weight information.