Midsagittal Brain: Anatomy, Function & Clinical View
The intricate architecture of the human brain reveals its secrets through distinct planes, with the midsagittal ion of the brain offering a unique perspective into its bilateral symmetry and functional organization. Neuroanatomy, a cornerstone of understanding brain structure, relies heavily on the examination of this central plane to delineate key structures such as the corpus callosum, which facilitates interhemispheric communication. Advanced neuroimaging techniques, including Magnetic Resonance Imaging (MRI), provide clinicians with non-invasive methods to visualize the midsagittal brain, aiding in the diagnosis of various neurological disorders. Pathologies affecting this region, such as agenesis of the corpus callosum, highlight the critical role of the midsagittal brain in neurological function, thereby emphasizing its importance in clinical neurology and neuroscience research.
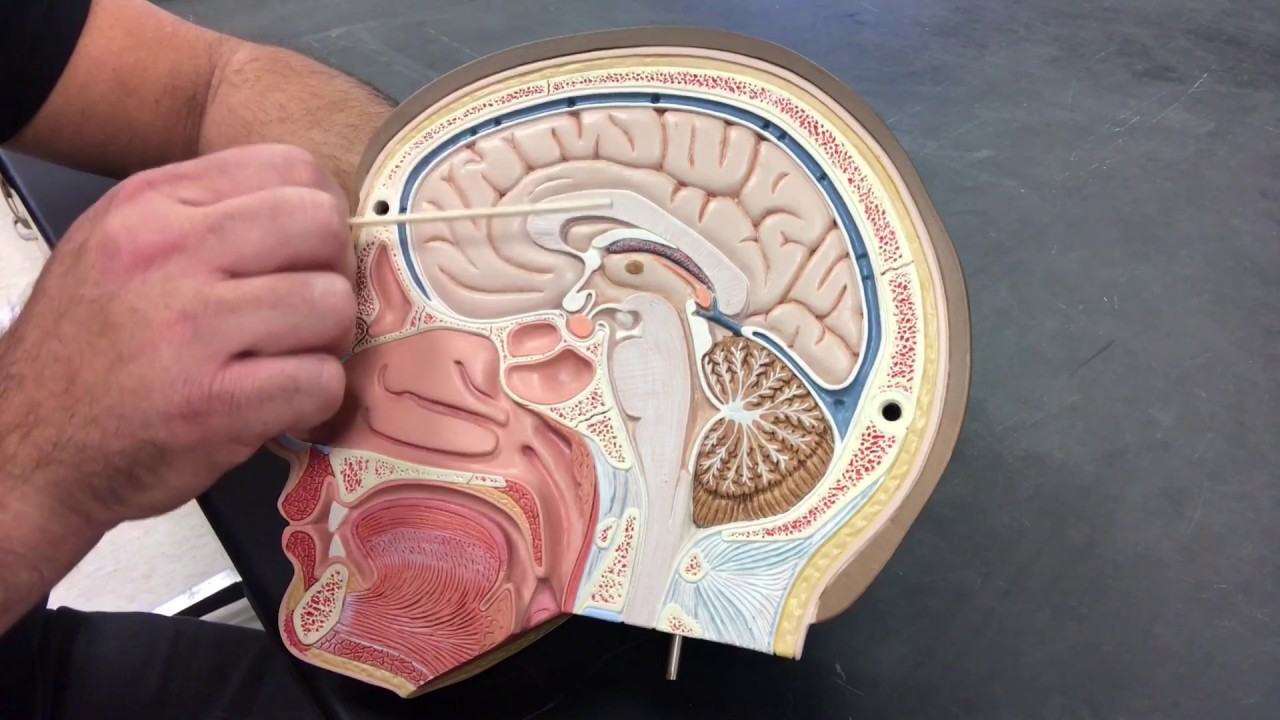
Image taken from the YouTube channel Anatomy and Physiology Prep , from the video titled Anatomy of the Brain - Midsagittal cut .
Unveiling the Midsagittal Brain View: An Anatomical Gateway
The human brain, an intricate and multifaceted organ, presents a considerable challenge to those seeking to decipher its complexities. To facilitate understanding and standardization in neuroanatomical studies, the concept of anatomical planes is paramount. Among these, the midsagittal plane stands out as a critical reference point.
Defining the Midsagittal Plane
The midsagittal plane, also known as the median plane, is an imaginary vertical plane that divides the brain, and indeed the entire body, into equal left and right halves. This division is crucial because it provides a symmetrical view of structures lying precisely at the midline.
Structures observed in this plane are, by definition, located in the very center of the brain, offering unique insights into their function and relationships with other brain regions.
The Significance of Standardization in Neuroanatomy
The adoption of the midsagittal plane as a standardized view is not arbitrary. Its importance stems from several key factors:
-
Consistency: It ensures that neuroanatomical descriptions and analyses are consistent across different researchers and studies. Without such standardization, comparing findings would be significantly more difficult.
-
Localization: It allows for precise localization of midline structures, facilitating the identification of abnormalities or pathological changes that may occur at these specific locations.
-
Reference: It serves as a reference point for understanding the spatial relationships between different brain regions. By visualizing the brain in this standardized orientation, neuroscientists can better comprehend how various structures interact and communicate with one another.
Midsagittal Structures: A Foundation for Understanding
This specific view offers a window into fundamental brain structures, offering a unique opportunity to study their contributions to overall brain function. The midline structures play pivotal roles in coordinating communication between hemispheres, controlling vital autonomic functions, and integrating sensory information.
The corpus callosum, the brainstem, the cerebellum, the thalamus, and the hypothalamus are all prominently visible in the midsagittal view. Understanding their individual contributions, and how they work together, is essential for a comprehensive grasp of neuroscience.
These structures will be examined in detail, including their anatomical features, their functional roles, and their clinical relevance. This analysis will serve to highlight the profound importance of the midsagittal plane in understanding the intricacies of the human brain.
Navigating the Midsagittal Landscape: Key Anatomical Structures
The human brain, an intricate and multifaceted organ, presents a considerable challenge to those seeking to decipher its complexities. To facilitate understanding and standardization in neuroanatomical studies, the concept of anatomical planes is paramount. Among these, the midsagittal plane offers a critical perspective, revealing a wealth of neural architecture. This section serves as a topographical introduction to the major brain structures visible from this vantage point, laying the groundwork for a more detailed exploration in subsequent sections.
A Bird's-Eye View of Midline Structures
The midsagittal view provides an invaluable, almost panoramic, perspective of the brain's centrally located structures. Imagine a precise cut dividing the brain into two symmetrical hemispheres.
What remains visible on the medial surface offers critical insights into brain function. This plane showcases structures that are crucial for interhemispheric communication, autonomic regulation, and even cognitive processing.
At the forefront of this view is the corpus callosum, a massive bundle of nerve fibers that acts as the primary conduit between the left and right cerebral hemispheres. It's the brain's superhighway for information exchange.
Ventrally, we observe the brainstem, a stalk-like structure that connects the cerebrum to the spinal cord. This region controls fundamental life functions.
Posteriorly, the cerebellum is evident, playing a vital role in motor coordination and balance.
Delineating Key Regions
Moving further into the telencephalon, diencephalon, and mesencephalon, more intricate structures appear.
The thalamus, often described as a sensory relay station, sits atop the brainstem, directing sensory information to the appropriate cortical areas.
Below the thalamus lies the hypothalamus, a crucial control center for autonomic functions and endocrine regulation.
The Ventricular System and Other Midline Landmarks
The ventricular system, a network of interconnected cavities filled with cerebrospinal fluid (CSF), is also readily apparent in the midsagittal view. The third ventricle and cerebral aqueduct are key components.
Other notable structures include the pineal gland, involved in circadian rhythm regulation.
The cingulate gyrus, part of the limbic system, is involved in emotion and behavior.
Additionally, the septum pellucidum, and fornix contribute to this intricate medial landscape.
Visualizing the Brain's Blueprint
Understanding the location and relationships of these structures within the midsagittal plane is fundamental to grasping overall brain function. Think of it as learning the street names and major intersections of a city before trying to understand its traffic patterns.
The midsagittal view, in essence, provides a blueprint for navigating the complex neural terrain. It gives a spatial context for understanding the roles of each individual structure and their interactions with one another.
The Corpus Callosum: Bridging the Hemispheres
Navigating the intricate landscape of the midsagittal brain view brings us to a critical structure: the corpus callosum. This expansive white matter tract serves as the primary bridge between the cerebral hemispheres, facilitating a constant exchange of information vital for integrated brain function. Understanding its structure and function is paramount, as disruptions to the corpus callosum can manifest in a range of neurological disorders.
Anatomy and Interhemispheric Communication
The corpus callosum is the largest white matter structure in the brain, composed of approximately 200 million axons. These axons connect corresponding cortical regions in the left and right hemispheres, enabling the seamless integration of sensory, motor, and cognitive processes.
- Rostrum: Connects the orbital frontal cortices.
- Genu: Connects the prefrontal cortices.
- Body: Connects the motor, premotor, and sensory cortices.
- Splenium: Connects the parietal, temporal, and occipital cortices.
This anatomical organization allows for a highly specific exchange of information between different brain regions. The corpus callosum's integrity is fundamental for cognitive functions that require coordination between both hemispheres.
Clinical Significance: Multiple Sclerosis
Multiple Sclerosis (MS) is a demyelinating disease that affects the central nervous system, often impacting the corpus callosum. The inflammation and axonal damage characteristic of MS can disrupt the white matter tracts of the corpus callosum, impairing interhemispheric communication.
MRI imaging often reveals lesions within the corpus callosum in individuals with MS. These lesions can disrupt the transmission of signals between the hemispheres, leading to a variety of neurological symptoms. These symptoms may include cognitive deficits, motor coordination problems, and sensory disturbances.
Agenesis of the Corpus Callosum
Agenesis of the corpus callosum (ACC) is a rare congenital disorder in which the corpus callosum is partially or completely absent. ACC can occur as an isolated anomaly or in association with other neurological conditions.
The clinical presentation of ACC is highly variable. Some individuals with ACC may be asymptomatic, while others may experience significant cognitive, motor, and behavioral impairments.
- Complete Agenesis: The entire structure is missing.
- Partial Agenesis: Only parts of the corpus callosum are formed.
The absence of the corpus callosum forces the brain to develop alternative pathways for interhemispheric communication. These alternative pathways, while functional, may not fully compensate for the loss of the corpus callosum.
Compensatory Mechanisms in Agenesis
In cases of ACC, other white matter tracts, such as the anterior commissure and the posterior commissure, may enlarge to partially compensate for the missing corpus callosum. Neuroplasticity plays a crucial role in the brain's adaptation to ACC, influencing the development of alternative neural networks. The extent of functional impairment depends on the degree of agenesis and the brain's ability to reorganize itself.
The Brainstem: Life's Foundation
Following our exploration of the corpus callosum, we now delve into the foundational structure that sustains life itself: the brainstem. This critical region serves as the essential relay station between the cerebrum, cerebellum, and the spinal cord. Its intricate network of nuclei and fiber tracts orchestrates a symphony of involuntary functions, ensuring our survival from moment to moment.
The Brainstem's Vital Role
The brainstem is, without exaggeration, the life support center of the brain. It regulates essential functions like heart rate, respiration, blood pressure, sleep-wake cycles, and consciousness. Damage to this area can have devastating consequences, often resulting in coma or even death.
Its position as the primary conduit for motor and sensory information necessitates an understanding of its intricate anatomy and diverse functionality. This section will dissect the key components of the brainstem, highlighting their specific roles in maintaining life and facilitating awareness.
Components of the Brainstem: A Detailed Look
The brainstem is comprised of three primary divisions: the midbrain (mesencephalon), the pons, and the medulla oblongata. Each region possesses a unique set of structures and functions, yet they all work in concert to maintain the body's homeostasis.
The Midbrain (Mesencephalon)
The midbrain, the most rostral portion of the brainstem, plays a critical role in motor control, visual and auditory processing, and arousal. Key structures within the midbrain include:
- Superior and Inferior Colliculi: These structures are involved in visual and auditory reflexes, respectively. The superior colliculus helps coordinate eye movements, while the inferior colliculus processes auditory information.
- Substantia Nigra: This dopamine-producing nucleus is crucial for motor control, and its degeneration is a hallmark of Parkinson's disease.
- Red Nucleus: Involved in motor coordination, particularly in the upper extremities.
The Pons
The pons, situated between the midbrain and the medulla, serves as a bridge connecting the cerebral cortex and the cerebellum. It also contains nuclei involved in:
- Sleep and Arousal: The pons plays a crucial role in regulating sleep-wake cycles and alertness.
- Motor Control: Nuclei within the pons contribute to motor coordination and balance.
- Cranial Nerve Function: Several cranial nerve nuclei are located within the pons, controlling functions such as facial sensation, eye movement, and chewing.
The Medulla Oblongata
The medulla oblongata, the most caudal portion of the brainstem, is essential for autonomic functions. Key functions regulated by the medulla include:
- Cardiac Function: Regulates heart rate and blood pressure through the cardiac and vasomotor centers.
- Respiratory Function: Controls breathing through the respiratory center, which responds to changes in blood pH and carbon dioxide levels.
- Reflexes: Mediates reflexes such as swallowing, coughing, and vomiting.
Consciousness and the Brainstem
The brainstem is home to the reticular activating system (RAS), a diffuse network of neurons that plays a critical role in maintaining wakefulness and alertness. Damage to the RAS can lead to a loss of consciousness or coma. The RAS receives input from various sensory pathways and projects to the cortex, activating and maintaining a state of arousal. Without this constant activation, the cortex is unable to process information effectively, resulting in a diminished state of awareness.
The Cerebellum: The Conductor of Movement
Following our exploration of the brainstem, the life-sustaining engine of the central nervous system, we now turn our attention to the cerebellum. This structure, nestled behind the cerebrum, plays a pivotal role in the orchestration of movement, balance, and coordination. Its intricate architecture and strategic location make it a crucial component of the motor system, and its visibility in the midsagittal view offers valuable insights into its function and integrity.
Cerebellar Function: Beyond Motor Skills
The cerebellum, often referred to as the "little brain," is far more than a mere motor controller. While its role in coordinating voluntary movements, maintaining posture, and ensuring balance is well-established, its influence extends to cognitive functions as well.
The cerebellum contributes to motor learning, adapting and refining movements through practice and experience.
It also plays a role in cognitive processes such as language, attention, and emotional regulation.
Damage to the cerebellum can manifest as a range of motor and cognitive deficits, highlighting its diverse and essential functions.
The Midsagittal View: A Glimpse into Cerebellar Anatomy
The midsagittal view provides a clear depiction of the cerebellum's overall structure and its relationship to other brain regions. In this view, the cerebellum appears as a distinct, rounded structure located inferior to the occipital lobe and posterior to the brainstem.
This perspective allows for the visualization of key cerebellar features.
The Arbor Vitae: The Tree of Life
One of the most striking features visible in the midsagittal view is the Arbor Vitae, the "tree of life." This distinctive branching pattern represents the cerebellar white matter, composed of myelinated axons that carry signals to and from the cerebellar cortex.
The Arbor Vitae is a testament to the intricate connectivity within the cerebellum, facilitating the rapid and efficient processing of information required for coordinated movement.
The branching pattern of the Arbor Vitae also increases the surface area for connections within the cerebellum, improving functionality.
Its appearance can serve as an indicator of cerebellar health, with alterations in its structure potentially signifying underlying pathology.
Cerebellar Lobes and the Vermis
The midsagittal view also allows for the identification of the cerebellar lobes.
The vermis, the midline structure that separates the two cerebellar hemispheres, is particularly prominent in this view.
The anterior, posterior, and flocculonodular lobes can be distinguished, each with specific functions related to motor control and coordination.
Clinical Significance: Cerebellar Disorders
The cerebellum is vulnerable to a variety of disorders, including stroke, tumors, and genetic conditions. Damage to the cerebellum can lead to a range of motor deficits, including ataxia (lack of coordination), dysmetria (inaccurate movements), and tremor.
The midsagittal view can be instrumental in diagnosing and monitoring these conditions.
MRI and CT scans can reveal structural abnormalities within the cerebellum.
The ability to visualize the cerebellum and its internal structures in the midsagittal plane is essential for understanding its function and diagnosing cerebellar disorders.
The Thalamus: Gateway to Conscious Perception
Following the cerebellum, the conductor of movement, we arrive at the thalamus. This structure, often likened to a central switchboard, acts as the primary relay station for sensory information ascending to the cerebral cortex. Its strategic location and diverse connectivity underscore its critical role in shaping our conscious experience. The thalamus filters, prioritizes, and modulates sensory input, ensuring that only the most relevant information reaches the higher cortical areas for further processing. Without this vital intermediary, our perception of the world would be a chaotic and undifferentiated barrage of stimuli.
The Sensory Relay Hub: Routing Information
The thalamus is not a monolithic structure, but rather a complex assembly of distinct nuclei, each serving a specific function. These nuclei receive inputs from various sensory pathways, including visual, auditory, somatosensory (touch, temperature, pain), and gustatory (taste). Each sensory modality has its own dedicated thalamic nucleus, ensuring that information is processed and relayed efficiently to the appropriate cortical area.
For example, the lateral geniculate nucleus (LGN) receives visual information from the retina and projects it to the visual cortex in the occipital lobe. Similarly, the medial geniculate nucleus (MGN) relays auditory information from the inner ear to the auditory cortex in the temporal lobe. The ventro-posterior lateral (VPL) and ventro-posterior medial (VPM) nuclei process somatosensory information from the body and face, respectively, and relay it to the somatosensory cortex in the parietal lobe.
This intricate organization allows the thalamus to act as a gatekeeper, selectively allowing or inhibiting the flow of sensory information. This gating mechanism is crucial for focusing attention and filtering out irrelevant stimuli, preventing sensory overload.
Beyond Sensory Relay: Modulatory Functions
While its role as a sensory relay is paramount, the thalamus also participates in a variety of other cognitive functions. It receives inputs from the basal ganglia, cerebellum, and prefrontal cortex, allowing it to modulate motor control, attention, and executive functions.
The thalamus plays a role in regulating sleep-wake cycles and levels of arousal. Certain thalamic nuclei, such as the reticular nucleus, contain inhibitory neurons that can suppress cortical activity, promoting sleep. Damage to these nuclei can lead to disturbances in sleep and wakefulness.
Clinical Significance: Thalamic Lesions and Sensory Deficits
The importance of the thalamus is underscored by the profound sensory and cognitive deficits that can result from thalamic lesions. Stroke or other damage to the thalamus can disrupt the flow of sensory information to the cortex, leading to sensory loss or abnormal sensory experiences. Thalamic pain syndrome, characterized by chronic and debilitating pain, can also result from thalamic lesions.
Furthermore, thalamic lesions can affect motor control, attention, and executive functions. Depending on the location and extent of the damage, individuals with thalamic lesions may experience difficulties with movement, coordination, attention, memory, and decision-making. The specific symptoms will vary depending on which thalamic nuclei are affected.
The thalamus remains a subject of ongoing research. It is a crucial structure in the brain, and its diverse functions extend far beyond simple sensory relay.
Hypothalamus: Maintaining Homeostasis
Following the thalamus, the sensory gateway, we turn our attention to the hypothalamus. This small but mighty structure plays a crucial role in maintaining the body's internal equilibrium. Its influence extends to autonomic functions, endocrine activity, and even behavior, making it a central regulator of life-sustaining processes.
The Hypothalamus: A Central Regulator
The hypothalamus, despite its diminutive size, exerts a profound influence on the body's internal environment.
It serves as a critical control center for a variety of essential functions. These functions are vital to survival.
Autonomic control, endocrine regulation, and behavioral modulation are all orchestrated by this central hub.
Autonomic Nervous System Control
One of the hypothalamus's primary responsibilities is to regulate the autonomic nervous system. This system controls involuntary functions such as heart rate, blood pressure, respiration, and digestion.
The hypothalamus integrates sensory information from the body and then adjusts autonomic output to maintain homeostasis. For example, in response to rising body temperature, the hypothalamus triggers sweating and vasodilation to promote heat loss.
Endocrine Activity and the Hypothalamic-Pituitary Axis
The hypothalamus is intimately connected to the endocrine system through its control of the pituitary gland. It secretes hormones that either stimulate or inhibit the release of hormones from the anterior pituitary.
This hypothalamic-pituitary axis is essential for regulating growth, metabolism, reproduction, and stress response.
Disruptions to this axis can have far-reaching consequences on overall health.
The Pituitary Gland: A Master Conductor
The pituitary gland, nestled below the hypothalamus, acts as the chief executive of the endocrine system. The hypothalamus dictates the activity of the pituitary gland.
It is often called the "master gland" because it regulates many of the hormone-secreting glands of the human body.
The interplay between the hypothalamus and pituitary gland is critical to the control of various physiological functions.
Behavioral Regulation
Beyond its autonomic and endocrine roles, the hypothalamus also influences behavior. It plays a role in regulating hunger, thirst, sleep-wake cycles, and sexual behavior.
Specific hypothalamic nuclei are involved in processing sensory information related to these drives. The hypothalamus then initiates appropriate behavioral responses.
Dysfunction in these areas can lead to disorders of eating, sleeping, and sexual function.
Mammillary Bodies: Memory and Olfaction
The mammillary bodies, two small, rounded structures located on the inferior surface of the hypothalamus, are closely associated with memory and olfaction.
They receive input from the hippocampus, a brain region crucial for memory formation, and project to the thalamus. This connection suggests a role in consolidating memories and integrating them with sensory information.
Damage to the mammillary bodies, as seen in Korsakoff's syndrome, can result in severe memory deficits.
The Ventricular System: CSF Circulation and Brain Health
Following the hypothalamus, the regulator of homeostasis, we delve into the intricate ventricular system, a network of interconnected cavities within the brain. This system, comprising the Third Ventricle, Cerebral Aqueduct, and Fourth Ventricle, is not merely an anatomical curiosity.
It is the lifeline for cerebrospinal fluid (CSF) circulation, a process critical for maintaining brain health and functionality. Understanding its anatomy and physiology is essential for grasping the pathogenesis of conditions like hydrocephalus and other neurological disorders.
Anatomy of the Ventricular System
The ventricular system consists of four interconnected ventricles within the brain, all filled with CSF. While the lateral ventricles are not clearly visible from a midsagittal view, understanding their contribution is key to the entire system. The central components readily observed in this view are:
-
Third Ventricle: This ventricle is a narrow cavity located in the midline of the brain, between the two halves of the thalamus. It receives CSF from the lateral ventricles via the foramina of Monro.
Its strategic position makes it vulnerable to compression or obstruction, potentially disrupting CSF flow.
-
Cerebral Aqueduct: Also known as the Aqueduct of Sylvius, this narrow channel connects the third and fourth ventricles. Its small diameter makes it particularly susceptible to blockage, a common cause of hydrocephalus.
-
Fourth Ventricle: Located between the pons and cerebellum, the fourth ventricle is the final ventricle in the system. It drains CSF into the subarachnoid space via the foramina of Luschka (laterally) and the foramen of Magendie (medially).
This release is crucial for the distribution of CSF around the brain and spinal cord.
Cerebrospinal Fluid (CSF): Production, Circulation, and Function
CSF, the clear, colorless fluid that bathes the brain and spinal cord, is produced primarily by the choroid plexus, a network of specialized cells within the ventricles. This fluid is not static; it continuously circulates throughout the ventricular system and the subarachnoid space, performing several vital functions:
-
Protection: CSF acts as a cushion, protecting the brain from physical trauma.
-
Waste Removal: It helps remove metabolic waste products from the brain.
-
Nutrient Transport: CSF transports nutrients and hormones to the brain.
-
Regulation of Intracranial Pressure: It helps maintain a stable intracranial pressure.
The circulation of CSF follows a precise pathway: from the lateral ventricles to the third ventricle, through the cerebral aqueduct to the fourth ventricle, and finally into the subarachnoid space. From there, it is reabsorbed into the bloodstream via the arachnoid granulations.
Any disruption to this intricate circulation can have severe consequences.
Clinical Significance: Hydrocephalus
Hydrocephalus, characterized by an abnormal accumulation of CSF within the brain, is a prime example of the clinical relevance of the ventricular system. This condition can arise from various causes:
-
Obstruction: Blockage within the ventricular system, such as at the cerebral aqueduct, can prevent CSF from flowing properly.
-
Impaired Absorption: Problems with the arachnoid granulations can hinder CSF reabsorption.
-
Overproduction: Rarely, the choroid plexus may produce too much CSF.
Hydrocephalus can lead to increased intracranial pressure, causing a range of symptoms, including headache, nausea, vision problems, and cognitive impairment. In infants, it can cause an abnormal enlargement of the head.
Diagnosis often involves neuroimaging techniques, such as MRI or CT scans, which can reveal the enlarged ventricles. Treatment typically involves the surgical placement of a shunt to drain excess CSF.
The Ventricular System: An Essential Component of Brain Health
In conclusion, the ventricular system is far more than a series of fluid-filled cavities. It is a dynamic system essential for CSF circulation, waste removal, and overall brain health. Understanding its anatomy and physiology is crucial for diagnosing and managing neurological conditions like hydrocephalus, highlighting its importance in clinical neuroscience.
Other Structures of Note: Pineal Gland, Cingulate Gyrus, and More
Following the ventricular system, the regulator of homeostasis, our exploration of the midsagittal brain view extends to other noteworthy structures, each playing a unique role in the orchestra of neurological functions. While the corpus callosum, brainstem, and cerebellum often dominate the discussion, these additional components contribute significantly to the overall understanding of the brain's intricate architecture.
The Pineal Gland: Melatonin and Circadian Rhythms
The pineal gland, a small endocrine gland located near the center of the brain, is a crucial component of the neuroendocrine system. Its primary function is the production and secretion of melatonin, a hormone that regulates sleep-wake cycles and circadian rhythms.
Melatonin secretion is influenced by light exposure, with production increasing in darkness and decreasing in light. This sensitivity to light allows the pineal gland to synchronize the body's internal clock with the external environment.
Beyond sleep regulation, the pineal gland has been implicated in various other physiological processes, including immune function, reproduction, and antioxidant defense.
Cerebral Hemispheres (Medial Surface): Higher Cognitive Functions
While the lateral surfaces of the cerebral hemispheres are readily apparent, the medial surface, as seen in the midsagittal view, offers a unique perspective on higher cognitive functions. This region includes portions of the frontal, parietal, and temporal lobes, each contributing to complex processes such as decision-making, spatial awareness, and memory.
The medial prefrontal cortex, for instance, plays a critical role in executive functions, including planning, working memory, and attention. Damage to this area can result in significant cognitive impairments.
The medial temporal lobe, including the hippocampus and amygdala, is essential for memory formation and emotional processing. These structures are particularly vulnerable in neurodegenerative diseases like Alzheimer's disease.
Cingulate Gyrus: Emotion, Learning, and Memory
The cingulate gyrus, a prominent structure located above the corpus callosum, is a key component of the limbic system, a network of brain regions involved in emotion, learning, and memory.
It plays a role in a wide range of functions, including emotional regulation, pain perception, and decision-making.
Different regions of the cingulate gyrus are associated with specific functions. The anterior cingulate cortex (ACC), for instance, is involved in error detection, conflict monitoring, and motivation. The posterior cingulate cortex (PCC) is involved in autobiographical memory and spatial orientation.
Septum Pellucidum: A Thin Membrane
The septum pellucidum is a thin, triangular membrane located in the midline of the brain, separating the two lateral ventricles. While its exact function is not fully understood, it is believed to play a role in cognitive and emotional processes.
Cavum septum pellucidum (CSP) is a common anatomical variant where a space exists between the two layers of the septum pellucidum. It is usually asymptomatic and found incidentally on brain imaging.
Fornix: Memory and Cognition
The fornix, a C-shaped bundle of nerve fibers, connects the hippocampus to other brain regions, including the hypothalamus and mammillary bodies. It plays a crucial role in memory formation and retrieval.
The fornix is considered a major output tract of the hippocampus, transmitting information related to spatial memory and declarative memory. Damage to the fornix can result in memory impairments, particularly difficulty with recalling recent events.
Together, these structures, while sometimes overlooked, contribute significantly to the complexity and functionality of the brain as visualized in the midsagittal plane. Understanding their individual roles and interconnections is essential for a comprehensive appreciation of neuroanatomy and its clinical relevance.
Following the ventricular system, the regulator of homeostasis, our exploration of the midsagittal brain view extends to other noteworthy structures, each playing a unique role in the orchestra of neurological functions. While the corpus callosum, brainstem, and cerebellum often dominate discussions of brain anatomy, it is essential to appreciate how these structures collaborate with other, sometimes less conspicuous, components. This understanding requires delving into the foundational sciences that underpin our knowledge of the brain: neuroanatomy and neurophysiology.
Structure Meets Function: Understanding Neuroanatomy and Neurophysiology
Neuroanatomy and neurophysiology are the bedrock upon which our understanding of brain function is built. Neuroanatomy provides the map, detailing the intricate architecture of the nervous system, its components, and their spatial relationships. Neurophysiology, on the other hand, explores the operational mechanisms, investigating how these structures function, communicate, and generate behavior. These are not disparate fields, but rather complementary disciplines that converge to provide a holistic view of the brain.
The Intertwined Nature of Neuroanatomy and Neurophysiology
The relationship between structure and function in the brain is not merely correlative; it is fundamentally causative. The specific morphology of a neuron, the precise layering of the cerebral cortex, or the intricate circuitry within the cerebellum—all dictate the functions that these structures can perform. Disruption to the anatomical integrity of a brain region inevitably leads to altered physiological function.
Consider, for example, the laminar organization of the cerebral cortex. The distinct layers, each characterized by a unique cellular composition and connectivity pattern, are responsible for specific aspects of cortical processing. Damage to a particular layer, through stroke or traumatic injury, will selectively impair the corresponding functions, highlighting the inextricable link between the physical architecture and the physiological operation.
Why Structure-Function Relationships Matter
The importance of understanding structure-function relationships extends beyond theoretical neuroscience. In clinical practice, this knowledge is crucial for diagnosing and treating neurological disorders. The ability to localize a lesion based on observed functional deficits, or to predict the functional consequences of a structural abnormality detected on neuroimaging, is a hallmark of effective neurological care.
Furthermore, the development of new therapeutic strategies, such as targeted drug delivery or neural prosthetics, relies heavily on a thorough understanding of how specific brain structures contribute to particular functions. Manipulating or modulating neural activity requires precise knowledge of the underlying anatomy and physiology.
Navigating the Complexity: A Multi-Scale Approach
The brain operates across multiple scales, from the molecular interactions within individual synapses to the large-scale networks that coordinate cognitive processes. Understanding structure-function relationships requires a multi-scale approach, integrating information from various levels of analysis.
Microscopic Level
At the microscopic level, techniques such as electron microscopy and immunohistochemistry allow us to examine the fine details of neuronal morphology and synaptic organization.
Mesoscopic Level
At the mesoscopic level, methods like electrophysiology and optogenetics enable us to probe the activity of individual neurons and circuits.
Macroscopic Level
At the macroscopic level, neuroimaging techniques, such as fMRI and EEG, provide insights into the activity of large-scale brain networks.
By combining these different perspectives, we can build a more complete picture of how the brain works, bridging the gap between its structural organization and its functional capabilities.
The Future of Structure-Function Research
The study of structure-function relationships in the brain is an ongoing endeavor, driven by technological advancements and theoretical innovations. Emerging techniques, such as connectomics and computational modeling, hold promise for unraveling the complexities of brain organization and function.
Connectomics, the comprehensive mapping of neural connections, aims to create detailed wiring diagrams of the brain, providing a structural foundation for understanding how information flows through neural circuits.
Computational modeling uses mathematical and computer simulations to recreate brain processes, allowing researchers to test hypotheses about how different structures interact to produce behavior.
These cutting-edge approaches, combined with traditional methods in neuroanatomy and neurophysiology, will continue to deepen our understanding of the most complex organ in the human body.
In essence, a comprehensive understanding of the midsagittal brain structures depends on the grasp of structure-function relationships that are essential for comprehending brain function as a whole.
Functional Significance: Interhemispheric Communication, CSF Flow, and Hormonal Control
Following the ventricular system, the regulator of homeostasis, our exploration of the midsagittal brain view extends to other noteworthy structures, each playing a unique role in the orchestra of neurological functions. While the corpus callosum, brainstem, and cerebellum often dominate discussions of brain anatomy, it is essential to appreciate how their integrated functionality shapes our understanding of neurological processes.
This section synthesizes the functional implications of the midsagittal structures, focusing on interhemispheric communication, the dynamics of cerebrospinal fluid (CSF), and the intricate hormonal controls mediated by the hypothalamus and pituitary gland.
Interhemispheric Communication and the Corpus Callosum
The corpus callosum stands as the principal commissure of the brain, facilitating a seamless exchange of information between the cerebral hemispheres. This massive bundle of nerve fibers allows for the integration of cognitive, sensory, and motor functions, ensuring a unified and coherent perception of the world.
Disruptions to the integrity of the corpus callosum, whether through trauma, disease, or congenital abnormalities, can lead to a range of neurological deficits. These deficits may manifest as difficulties in coordinating movements, impaired sensory processing, or disruptions in higher-level cognitive functions.
CSF Flow and the Ventricular System
The ventricular system, prominently visualized in the midsagittal view, represents a network of interconnected cavities filled with cerebrospinal fluid (CSF). Beyond its role as a mechanical cushion, CSF serves as a critical medium for nutrient delivery, waste removal, and the maintenance of a stable chemical environment within the brain.
The flow of CSF through the ventricles, propelled by the choroid plexus, is essential for the proper functioning of the central nervous system.
Obstructions within the ventricular system, or imbalances in CSF production and absorption, can lead to hydrocephalus. This condition results in the abnormal accumulation of fluid within the brain, placing pressure on neural tissue and potentially causing severe neurological damage.
Hypothalamic-Pituitary Axis: Hormonal Orchestration
The hypothalamus and pituitary gland, intimately connected both structurally and functionally, form the centerpiece of the endocrine system's control over the body. This axis orchestrates a complex interplay of hormonal signals that regulate a wide array of physiological processes.
These processes include growth, metabolism, reproduction, and the body's response to stress.
The hypothalamus, acting as the command center, releases hormones that either stimulate or inhibit the release of hormones from the pituitary gland. The pituitary, in turn, secretes hormones that target various organs and tissues throughout the body, exerting their influence on cellular function.
Disruptions to this delicate hormonal balance can have far-reaching consequences, leading to a spectrum of endocrine disorders. These disorders can affect growth and development, reproductive health, and the body's ability to maintain homeostasis. The midline location of these structure highlights its vital contribution.
Clinical Applications: Visualizing the Brain with Imaging Techniques
Following the ventricular system, the regulator of homeostasis, our exploration of the midsagittal brain view extends to other noteworthy structures, each playing a unique role in the orchestra of neurological functions. While the corpus callosum, brainstem, and other key regions reveal valuable anatomical insights, it is the application of modern neuroimaging that truly unlocks the potential of the midsagittal perspective in clinical settings.
The ability to visualize these structures in vivo is paramount for diagnosing and managing a wide range of neurological conditions. Various imaging modalities offer unique perspectives, each with its strengths and limitations in revealing the intricacies of the brain.
Magnetic Resonance Imaging (MRI): High-Resolution Anatomical Detail
MRI has become the gold standard for detailed neuroimaging. It leverages powerful magnetic fields and radio waves to generate high-resolution images of the brain's soft tissues.
Strengths of MRI
MRI's primary advantage is its superior soft tissue contrast.
This allows for exquisite visualization of structures like the corpus callosum, brainstem, and cerebellum, as well as subtle abnormalities that might be missed on other imaging techniques.
Furthermore, MRI does not involve ionizing radiation, making it a safer option for repeated imaging studies, particularly in vulnerable populations such as children and pregnant women.
Limitations of MRI
Despite its strengths, MRI also has limitations.
It can be time-consuming and expensive. The presence of metallic implants can be a contraindication due to the strong magnetic fields.
Patients with claustrophobia may also find the confined space of the MRI scanner challenging. MRI is also less sensitive to acute hemorrhage compared to CT scans.
Computed Tomography (CT) Scan: Rapid and Accessible Imaging
CT scanning utilizes X-rays to create cross-sectional images of the brain. It is a widely available and relatively inexpensive imaging modality.
Strengths of CT Scan
CT scans are particularly useful in emergency situations, as they can be acquired rapidly.
They are also highly sensitive to detecting acute hemorrhage, making them the preferred imaging modality for initial evaluation of head trauma or suspected stroke.
CT scans are less susceptible to artifacts from metallic implants compared to MRI.
Limitations of CT Scan
The primary drawback of CT scanning is the use of ionizing radiation.
Repeated CT scans can increase the risk of radiation-induced health problems.
CT scans also offer lower soft tissue contrast compared to MRI, making it more difficult to visualize subtle anatomical details or subtle abnormalities.
Diffusion Tensor Imaging (DTI): Mapping White Matter Tracts
DTI is a specialized MRI technique that allows for the visualization and quantification of white matter tracts in the brain. It measures the diffusion of water molecules along nerve fibers.
Strengths of DTI
DTI provides valuable information about the integrity and organization of white matter pathways, such as the corpus callosum.
It can be used to detect subtle white matter abnormalities that may not be apparent on conventional MRI. DTI is particularly useful in studying conditions like traumatic brain injury, multiple sclerosis, and neurodevelopmental disorders.
Limitations of DTI
DTI is a more complex imaging technique compared to conventional MRI and CT scanning.
Image acquisition and processing can be challenging, and the interpretation of DTI findings requires specialized expertise. DTI is also susceptible to artifacts from motion and magnetic field inhomogeneities.
The interpretation of results requires specific training and knowledge.
Pathologies and the Midsagittal View: Stroke, TBI, and Hydrocephalus
Following the ventricular system, the regulator of homeostasis, our exploration of the midsagittal brain view extends to other noteworthy structures, each playing a unique role in the orchestra of neurological functions. While the corpus callosum, brainstem, and other key regions... Understanding how these structures are affected by various pathologies is crucial for accurate diagnosis and treatment planning. The midsagittal view offers a valuable perspective for visualizing and assessing the impact of conditions like stroke, traumatic brain injury (TBI), hydrocephalus, and brain tumors.
The Devastating Impact of Stroke
Stroke, a leading cause of disability and mortality, often manifests with distinct midline findings on neuroimaging. Ischemic strokes, resulting from a blockage of blood supply, can affect the anterior cerebral artery (ACA), leading to infarction of medial frontal lobe structures visible in the midsagittal plane.
Hemorrhagic strokes, caused by bleeding in the brain, can also have profound effects on midline structures.
The presence of blood within the ventricles, detected through imaging, can obstruct CSF flow and increase intracranial pressure. Early detection and intervention are critical in minimizing the neurological damage caused by stroke.
Traumatic Brain Injury: A Multifaceted Challenge
Traumatic brain injury (TBI) can result in a range of injuries visible in the midsagittal view, including diffuse axonal injury (DAI), contusions, and hematomas. DAI, often caused by acceleration-deceleration forces, can disrupt axonal connections throughout the brain, impacting consciousness and cognitive function.
Midline shift, a displacement of midline structures due to swelling or hemorrhage, is a critical indicator of increased intracranial pressure and potential herniation. The severity of midline shift is directly correlated with the patient's neurological outcome.
Hydrocephalus: Disrupting CSF Dynamics
Hydrocephalus, an abnormal accumulation of cerebrospinal fluid (CSF) within the brain, can lead to ventricular enlargement and increased intracranial pressure. Obstructive hydrocephalus, caused by a blockage in the CSF pathways, can be readily visualized in the midsagittal view.
The site of obstruction, such as the cerebral aqueduct or the fourth ventricle, can be identified and targeted for treatment. Communicating hydrocephalus, where the obstruction is outside the ventricular system, results in diffuse ventricular enlargement.
Early diagnosis and intervention through shunt placement or endoscopic third ventriculostomy can prevent irreversible brain damage.
Brain Tumors: A Spectrum of Midline Involvement
Brain tumors can arise in or near midline structures, leading to a variety of neurological deficits. Tumors of the pituitary gland, pineal gland, or hypothalamus can disrupt hormonal function, vision, and autonomic control.
Meningiomas, tumors arising from the meninges, can compress adjacent brain structures, including the corpus callosum and brainstem. The midsagittal view is essential for assessing the size, location, and extent of tumor involvement, guiding surgical planning and radiation therapy.
Imaging Modalities in Diagnosis
Magnetic resonance imaging (MRI) is the gold standard for visualizing midline pathologies due to its superior soft tissue resolution. Computed tomography (CT) scans are valuable for detecting acute hemorrhage and skull fractures in TBI.
Diffusion tensor imaging (DTI) can assess the integrity of white matter tracts, including the corpus callosum, in patients with TBI or stroke. These advanced imaging techniques provide critical information for diagnosis, prognosis, and treatment planning.
The Blood-Brain Barrier: Protecting the Midline Structures
Following the exploration of pathologies affecting the midsagittal structures, it is critical to address the gatekeeper responsible for maintaining the central nervous system's delicate environment: the blood-brain barrier (BBB). This sophisticated interface, a highly selective permeability barrier, separates the circulating blood from the brain extracellular fluid in the central nervous system (CNS). Its integrity is paramount for optimal neuronal function and shields the vulnerable midline structures from harmful substances, pathogens, and fluctuations in systemic conditions.
The BBB: A Selective Guardian
The BBB is not merely a physical barrier; it's a dynamic and metabolically active interface. It is primarily composed of brain endothelial cells connected by tight junctions, which severely restrict paracellular transport.
These tight junctions are far more complex and restrictive than those found in other tissues.
Furthermore, the BBB incorporates astrocytes, pericytes, and a specialized basement membrane, creating a neurovascular unit that regulates what enters and exits the brain.
This intricate structure ensures that only essential nutrients, such as glucose and amino acids, can pass through via specific transport mechanisms.
Simultaneously, it prevents the entry of toxins, inflammatory cells, and large molecules that could disrupt neuronal function.
Relevance to Midline Structures
The midline structures, including the corpus callosum, hypothalamus, and parts of the brainstem and cerebellum, are particularly vulnerable to disruptions in the BBB.
The hypothalamus, for instance, relies on circumventricular organs, specialized brain regions lacking a fully functional BBB, to sample the blood and regulate hormonal balance and homeostasis.
This inherently makes it more susceptible to circulating toxins if the BBB elsewhere is compromised.
Inflammation within the BBB can lead to neuroinflammation, a process implicated in a range of neurological disorders.
Moreover, damage to the BBB can exacerbate conditions such as stroke, where the influx of blood components into brain tissue can worsen neuronal damage.
Clinical Implications of BBB Dysfunction
BBB dysfunction is implicated in numerous neurological and psychiatric disorders, including multiple sclerosis (MS), Alzheimer's disease, epilepsy, and traumatic brain injury (TBI).
In MS, the breakdown of the BBB allows immune cells to infiltrate the CNS, leading to demyelination and neurodegeneration.
In Alzheimer's disease, BBB disruption contributes to the accumulation of amyloid-beta plaques and neurofibrillary tangles, hallmarks of the disease.
BBB permeability is also increased after TBI, potentially leading to vasogenic edema and secondary brain injury.
Imaging the Blood-Brain Barrier
Visualizing the integrity of the BBB in vivo is crucial for diagnosing and monitoring various neurological conditions.
Contrast-enhanced MRI is a commonly used technique to assess BBB permeability.
In this method, a contrast agent, such as gadolinium, is injected into the bloodstream, and its leakage into brain tissue indicates BBB breakdown.
Advanced imaging techniques, such as dynamic contrast-enhanced MRI and diffusion-weighted imaging, provide more detailed information about BBB function and permeability.
Therapeutic Strategies Targeting the BBB
Given the importance of the BBB in brain health, several therapeutic strategies are being developed to modulate its function.
These strategies aim to either enhance drug delivery to the brain or restore BBB integrity in neurological disorders.
Approaches include using nanoparticles to transport drugs across the BBB, temporarily disrupting the BBB with focused ultrasound, and developing drugs that target specific BBB transporters.
Furthermore, research is focused on identifying compounds that can strengthen tight junctions and reduce BBB permeability in conditions characterized by BBB breakdown.
Tools of the Trade: Brain Atlases and Neuroimaging Software
Following the exploration of pathologies affecting the midsagittal structures, it is essential to turn our attention to the indispensable tools and techniques that empower neuroscientists and clinicians alike to dissect and decipher the intricacies of the brain. These tools range from standardized anatomical reference guides to cutting-edge computational platforms designed for visualizing and analyzing neuroimaging data.
Brain Atlases: Navigational Charts of the Mind
Brain atlases serve as foundational resources, providing standardized anatomical frameworks for locating and identifying specific brain structures. These atlases are not merely static maps; they represent a synthesis of anatomical knowledge gleaned from extensive histological studies and neuroimaging data.
Historically, brain atlases were based on post-mortem dissections, offering detailed, yet inherently variable, representations of the human brain. The advent of in vivo neuroimaging has revolutionized atlas creation, enabling the development of probabilistic atlases that account for inter-subject variability in brain structure.
Types of Brain Atlases
Several types of brain atlases cater to diverse research and clinical needs:
-
Cytoarchitectonic Atlases: Based on cellular organization, these atlases define brain regions according to distinct cytoarchitectural features. The Brodmann atlas is a classic example, delineating cortical areas based on variations in neuronal layering.
-
Probabilistic Atlases: Constructed from large neuroimaging datasets, these atlases provide probabilistic maps indicating the likelihood of a particular voxel belonging to a specific brain structure. They are crucial for group-level analyses and accounting for anatomical variability.
-
Connectivity-Based Atlases: Derived from diffusion tensor imaging (DTI) or functional connectivity analyses, these atlases delineate brain regions based on their patterns of structural or functional connectivity. They offer insights into the brain's network organization.
Importance in Research and Clinical Practice
Brain atlases are indispensable tools for neuroimaging research, enabling researchers to:
- Precisely localize brain activity: Identify the anatomical regions activated during cognitive tasks or in response to stimuli.
- Compare findings across studies: Standardize anatomical referencing to facilitate meta-analyses and replication efforts.
- Guide stereotactic neurosurgery: Plan surgical interventions with pinpoint accuracy.
In clinical settings, atlases aid in:
- Identifying anatomical abnormalities: Detect structural changes associated with neurological disorders.
- Assessing lesion location and extent: Determine the impact of stroke, trauma, or tumors on specific brain regions.
- Planning radiation therapy: Target tumors while minimizing damage to surrounding healthy tissue.
Neuroimaging Software: Decoding Brain Activity and Structure
Neuroimaging software encompasses a broad range of computational tools designed for processing, analyzing, and visualizing neuroimaging data obtained from techniques such as MRI, CT, and PET. These software packages offer sophisticated algorithms for image reconstruction, artifact removal, statistical analysis, and data visualization.
Key Functionalities of Neuroimaging Software
Modern neuroimaging software platforms provide a suite of functionalities essential for extracting meaningful information from brain images:
- Image Preprocessing: Corrects for distortions, motion artifacts, and other sources of noise in the data.
- Segmentation: Automatically or semi-automatically identifies and delineates different brain structures.
- Statistical Analysis: Performs statistical tests to identify significant differences in brain activity or structure between groups or conditions.
- Visualization: Generates 2D and 3D renderings of brain data, allowing for interactive exploration and presentation of results.
Popular Neuroimaging Software Packages
Several widely used neuroimaging software packages are available, each with its strengths and weaknesses:
-
SPM (Statistical Parametric Mapping): A comprehensive toolbox for fMRI data analysis, offering a wide range of statistical models and preprocessing options.
-
FSL (FMRIB Software Library): Another popular fMRI analysis package, known for its user-friendly interface and advanced diffusion imaging tools.
-
FreeSurfer: A powerful tool for cortical surface reconstruction and analysis, enabling detailed measurements of cortical thickness and surface area.
-
AFNI (Analysis of Functional NeuroImages): A versatile software package for fMRI analysis, known for its emphasis on data visualization and interactive exploration.
The Future of Neuroimaging Analysis
The field of neuroimaging analysis is rapidly evolving, driven by advances in machine learning and artificial intelligence. Deep learning algorithms are increasingly being used to automate image segmentation, predict disease outcomes, and uncover novel patterns of brain activity.
Furthermore, the integration of multi-modal neuroimaging data (e.g., combining fMRI with EEG or MEG) promises to provide a more comprehensive understanding of brain function and dysfunction. These advancements hold the potential to transform our ability to diagnose and treat neurological and psychiatric disorders.
Video: Midsagittal Brain: Anatomy, Function & Clinical View
FAQs: Midsagittal Brain
What structures are best viewed in a midsagittal view of the brain?
The midsagittal view reveals key structures like the corpus callosum (connecting hemispheres), the thalamus (sensory relay), the hypothalamus (hormonal control), the pituitary gland (hormone secretion), and the brainstem. These are prominently visible because the midsagittal ion of the brain cuts directly down the midline.
How does the midsagittal view help understand brain function?
By exposing structures centrally located within the brain, the midsagittal view allows doctors to understand how different regions coordinate functions. For example, you can understand the role of structures like the pineal gland that help regulate sleep-wake cycles, or the role of the cingulate gyrus in emotion processing, from a midsagittal ion of the brain.
What clinical information can be gained from a midsagittal brain scan?
A midsagittal scan can reveal abnormalities like tumors in the pituitary gland, lesions in the corpus callosum (e.g., due to multiple sclerosis), or structural anomalies affecting cerebrospinal fluid flow. These observations become clear when you view the brain from this specific midsagittal ion.
Why is the corpus callosum important, and how is it visible in a midsagittal slice?
The corpus callosum is a massive bundle of nerve fibers connecting the left and right cerebral hemispheres, enabling communication between them. The corpus callosum appears in a midsagittal ion of the brain as a large, C-shaped structure right above the thalamus.
So, there you have it – a glimpse into the fascinating world of the midsagittal section of the brain! Hopefully, this has given you a better understanding of its intricate anatomy, essential functions, and clinical relevance. It's truly remarkable how this single plane reveals so much about what makes us, well, us.