Basal Taxon: Unlocking Evolutionary Ancestry Secrets
The field of phylogenetics employs rigorous methodologies to reconstruct the evolutionary history of life, and cladistics represents a key approach within this field. Basal taxon represents a lineage that diverged early in the evolutionary history of a group, often providing insights into the characteristics of ancestral forms. The Tree of Life project, an ambitious endeavor to map the relationships between all living organisms, relies heavily on the identification and placement of basal taxa. Charles Darwin's theory of evolution by natural selection provides the foundational framework for understanding how basal taxa, like all organisms, have diversified over time.
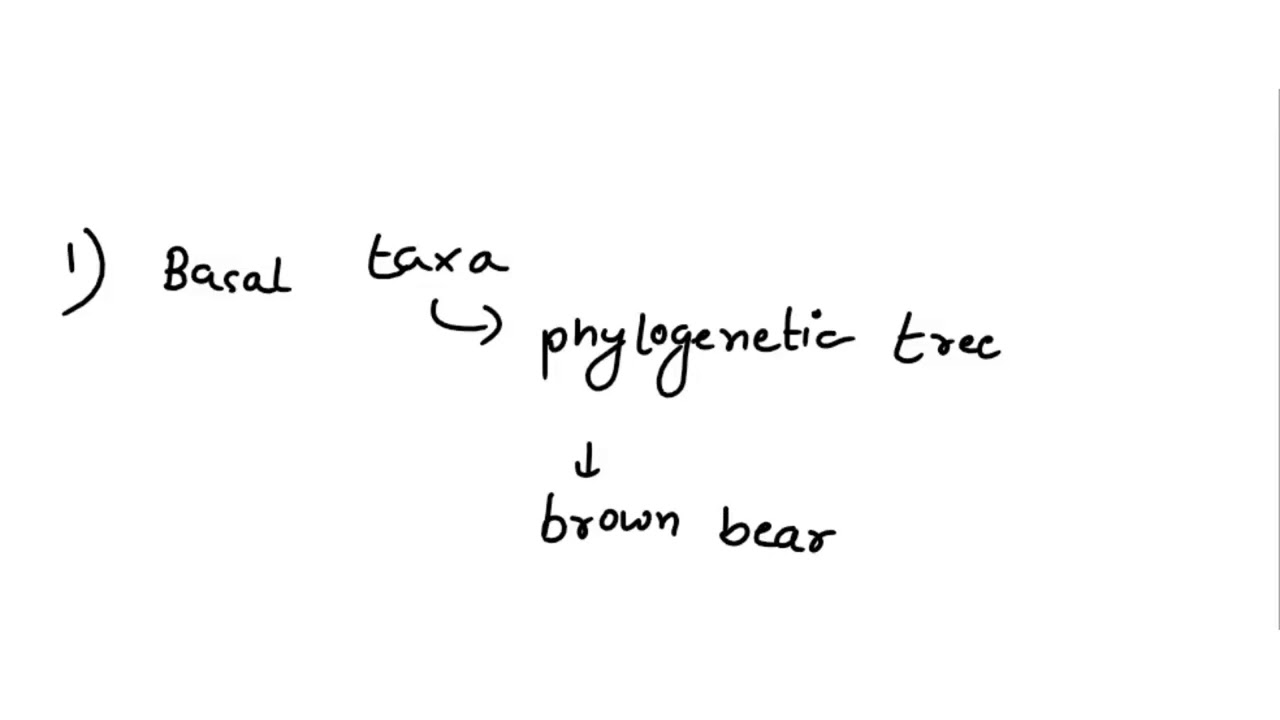
Image taken from the YouTube channel Harold Edwards , from the video titled Phylogeny, Basal taxa, Sister taxa, Heterochrony, Polytomy, Parsimony, Orthologous, Paralogous, Mon… .
Unraveling the Tree of Life with Phylogeny
Phylogeny, at its core, is the study of evolutionary relationships among organisms. It seeks to map the intricate connections that bind all life on Earth, offering a framework for understanding the grand tapestry of biodiversity.
Its significance extends far beyond academic curiosity, impacting fields as diverse as medicine, agriculture, and conservation.
The Profound Significance of Phylogeny
Understanding evolutionary relationships is crucial for tracing the origins and spread of infectious diseases. By constructing phylogenetic trees of viruses and bacteria, scientists can pinpoint the sources of outbreaks and develop effective strategies for containment.
Moreover, phylogeny informs conservation efforts by identifying species that are most at risk of extinction and prioritizing areas with high evolutionary distinctiveness.
This knowledge allows us to make informed decisions about resource allocation and habitat preservation. It also facilitates crop improvement by identifying wild relatives of domesticated plants that may possess valuable traits like disease resistance or drought tolerance.
A Historical Perspective: Darwin and Haeckel
The seeds of phylogenetic thinking were sown in the mid-19th century with the publication of Charles Darwin's On the Origin of Species. Darwin's theory of evolution by natural selection provided the conceptual foundation for understanding how species change over time and how new species arise from pre-existing ones.
He also recognized the importance of common ancestry, suggesting that all life on Earth is ultimately descended from a single, universal ancestor.
Ernst Haeckel, a German biologist and philosopher, further popularized the idea of phylogeny through his elaborate "genealogical trees" of life. While Haeckel's trees were based on limited data and often reflected his own biases, they played a crucial role in visualizing evolutionary relationships and inspiring future generations of scientists.
Core Concepts: Evolutionary Biology, Common Ancestry, and Divergence
Phylogeny is deeply rooted in the principles of evolutionary biology, the overarching theoretical framework that explains the diversity and adaptation of life.
A central tenet of evolutionary biology is the concept of common ancestry. This postulates that all living organisms share a common ancestor from which they have diversified over millions of years.
This concept is supported by a wealth of evidence, including the universality of the genetic code and the presence of homologous structures in diverse organisms.
Divergence, the process by which populations evolve into distinct species, is another key concept in phylogeny. As populations become isolated from one another, they accumulate genetic differences that can eventually lead to reproductive incompatibility and the formation of new species.
Decoding Evolutionary History: Methods in Phylogenetic Analysis
Having established the foundational importance of phylogeny, the next logical step involves understanding the methodologies employed to reconstruct evolutionary relationships. This involves a diverse toolkit, ranging from classical comparative methods to cutting-edge molecular techniques. Understanding these methods allows for a more critical evaluation of phylogenetic hypotheses.
Cladistics: Mapping Shared Ancestry
Cladistics forms the cornerstone of modern phylogenetic analysis. At its heart, cladistics is a system of classification that groups organisms based on shared derived characteristics, or synapomorphies. These traits are inherited from a common ancestor and distinguish a particular clade (a group consisting of an ancestor and all its descendants) from other lineages.
This approach contrasts with earlier methods that relied on overall similarity, which could be misleading due to convergent evolution.
Central to the development of cladistics was Willi Hennig, a German entomologist. Hennig's rigorous methodology provided a systematic framework for inferring evolutionary relationships based on character data. His work revolutionized the field of systematics and laid the groundwork for the modern phylogenetic approach.
Visualizing Evolutionary Relationships: Phylogenetic Trees
The results of cladistic analyses are typically represented visually as phylogenetic trees, also known as cladograms. These diagrams depict the hypothesized evolutionary relationships among a group of organisms.
Understanding the Components of a Cladogram
Cladograms are composed of several key components:
-
Nodes: Represent the common ancestors from which different lineages diverged. The position of a node reflects the hypothesized point in evolutionary history when the ancestral population split into two or more distinct groups.
-
Root: Represents the most ancestral lineage in the tree. It is the starting point from which all other lineages ultimately derive.
-
Outgroup: A taxon that is closely related to, but not within, the group of organisms being studied. The outgroup serves as a reference point for rooting the tree and for determining the polarity of character evolution (i.e., whether a character state is ancestral or derived).
Character Analysis: Distinguishing Homology from Homoplasy
Phylogenetic inference hinges on the careful analysis of character data. A character is a heritable attribute of an organism that can exist in different forms, known as character states.
For example, the presence or absence of a tail is a character, while "tail present" and "tail absent" are the character states.
A critical step in phylogenetic analysis is distinguishing between homology and homoplasy.
-
Homologous characters are similar due to shared ancestry. For example, the bones in the forelimbs of mammals (e.g., humans, bats, whales) are homologous, as they are derived from a common ancestor.
-
Homoplasious characters, on the other hand, are similar due to independent evolution, often as a result of similar environmental pressures. This is also known as convergent evolution. An example is the wings of birds and bats. Although both structures enable flight, they evolved independently and do not share a common evolutionary origin.
Distinguishing between homology and homoplasy is crucial for accurate phylogenetic reconstruction.
Molecular Phylogenetics: The Power of Genes
The advent of molecular biology has revolutionized phylogenetic analysis. Molecular phylogenetics utilizes data from genes and proteins to infer evolutionary relationships. This approach offers several advantages over traditional morphological methods.
The Role of Molecular Data
Molecular data provides a vast amount of information that can be used to reconstruct phylogenies, especially with the development of high-throughput sequencing. DNA sequences, in particular, are highly amenable to quantitative analysis.
The Molecular Clock
The molecular clock is a technique that uses the rate at which mutations accumulate in DNA to estimate the time of divergence between lineages. The assumption is that, for a given gene, the rate of mutation is relatively constant over time.
By calibrating the molecular clock with fossil data or other independent estimates of divergence times, it is possible to infer the timing of evolutionary events.
Phylogenomics
Phylogenomics represents a further advancement in molecular phylogenetics. It involves using genomic data to infer evolutionary relationships. By analyzing entire genomes, phylogenomics can provide a more comprehensive and robust picture of evolutionary history than traditional methods that rely on a limited number of genes. Phylogenomics helps in resolving even the most difficult phylogenetic questions.
The Interdisciplinary Nature of Phylogeny: Drawing from Diverse Fields
Having established the foundational importance of phylogeny, the next logical step involves understanding the methodologies employed to reconstruct evolutionary relationships. This involves a diverse toolkit, ranging from classical comparative methods to cutting-edge molecular techniques. However, phylogenetic analysis does not exist in a vacuum; it is fundamentally interdisciplinary, drawing strength and insights from a multitude of scientific fields.
The power of phylogenetic inference lies in its ability to synthesize data from diverse sources, integrating evidence from the fossil record, anatomical structures, and vast molecular datasets. This multifaceted approach allows for a more robust and comprehensive understanding of evolutionary relationships than any single discipline could provide on its own.
Paleontology: Unearthing Evolutionary Timelines
Paleontology, the study of prehistoric life through fossil remains, offers a unique temporal dimension to phylogenetic studies. Fossils provide direct evidence of extinct organisms, revealing ancestral forms and transitional stages that are often missing from the extant biota.
The fossil record helps to calibrate molecular clocks, providing minimum age constraints for divergence events. Moreover, fossil discoveries can challenge existing phylogenetic hypotheses, forcing scientists to re-evaluate evolutionary relationships in light of new morphological and ecological data.
Comparative Morphology: Deciphering Structural Signatures of Evolution
Comparative morphology, the analysis of anatomical structures across different species, plays a crucial role in identifying homologous traits – features inherited from a common ancestor. These shared characters, whether skeletal elements, organ systems, or developmental patterns, provide valuable evidence for grouping organisms together in phylogenetic trees.
However, morphological data can also be misleading. Homoplasy, the independent evolution of similar traits in unrelated lineages, can obscure true phylogenetic relationships. Careful character analysis, distinguishing between homology and homoplasy, is essential for accurate phylogenetic reconstruction.
Bioinformatics: Harnessing the Power of Computational Analysis
The rise of molecular phylogenetics has been fueled by advancements in DNA sequencing technology and the concurrent development of sophisticated bioinformatics tools. Bioinformatics provides the computational framework for analyzing vast amounts of molecular data, identifying patterns of sequence similarity and difference, and constructing phylogenetic trees based on statistical algorithms.
The Role of Computational Tools
Software packages like MrBayes, RAxML, and BEAST empower researchers to perform complex phylogenetic analyses, incorporating diverse datasets and accommodating uncertainty in the data. These tools allow for the exploration of alternative phylogenetic hypotheses and the assessment of statistical support for different evolutionary scenarios.
Big Data and Phylogenetic Inference
The era of genomics has ushered in a new age of "phylogenomics," where entire genomes are used to infer evolutionary relationships. Analyzing these massive datasets requires advanced computational infrastructure and sophisticated algorithms. Phylogenomics offers the potential to resolve long-standing phylogenetic controversies and to reveal the intricate details of evolutionary history with unprecedented resolution.
In conclusion, the interdisciplinary nature of phylogeny is its greatest strength. By integrating data from paleontology, comparative morphology, and bioinformatics, researchers can construct a more complete and accurate picture of the tree of life, illuminating the evolutionary processes that have shaped the diversity of life on Earth.
Having established the foundational importance of phylogeny, the next logical step involves understanding the methodologies employed to reconstruct evolutionary relationships. This involves a diverse toolkit, ranging from classical comparative methods to cutting-edge molecular techniques. However, understanding the deep branches of the "Tree of Life" requires a careful examination of basal taxa, which offer unique insights into the early evolution of various lineages.
Understanding Basal Taxa: Glimpses into Early Evolutionary History
Basal taxa represent lineages that diverged early in the evolutionary history of a group. Their characteristics often reflect ancestral traits, providing valuable clues about the evolutionary transitions that occurred along different branches of the tree of life. By studying these organisms, scientists can gain a better understanding of the origin and diversification of life.
The Domains of Life: Archaea and Bacteria
Carl Woese's groundbreaking work in the 1970s revolutionized our understanding of life's earliest divisions. His ribosomal RNA (rRNA) sequencing revealed that prokaryotes were not a homogenous group, but rather comprised two distinct domains: Bacteria and Archaea.
This discovery challenged the long-held view of a simple prokaryote-eukaryote dichotomy. Archaea, initially found in extreme environments, are now known to inhabit a wide range of habitats.
Their unique cell membrane lipids, ribosomal structure, and metabolic pathways distinguish them from Bacteria and provide evidence of their ancient divergence. Woese's work underscored the importance of molecular data in refining phylogenetic classifications.
Porifera: The Ancient Sponges
Sponges (Porifera) occupy a pivotal position as one of the earliest-diverging animal lineages. Their simple body plan, characterized by a lack of true tissues and organs, reflects the ancestral state of multicellular animals. Sponges filter feed using specialized cells called choanocytes, which bear a striking resemblance to choanoflagellates – free-living, unicellular eukaryotes.
This similarity supports the hypothesis that sponges evolved from choanoflagellate-like ancestors. Their cellular organization and unique mode of development provide valuable insights into the evolution of animal multicellularity.
Studying sponges sheds light on the origin of key animal traits.
Lampreys: Jawless Vertebrates
Lampreys (Petromyzontiformes) represent a basal lineage within the vertebrates. These jawless fish possess a cartilaginous skeleton and a distinctive oral disc used for attaching to prey.
Their evolutionary position helps to illuminate the origin of jaws and bony skeletons in vertebrates. Examining lamprey anatomy and physiology can offer clues about the traits present in the earliest vertebrates.
Their existence offers a window into vertebrate evolution before the advent of jaws.
Ginkgo biloba: A Living Fossil
Ginkgo biloba, often referred to as a "living fossil," is the sole surviving species of a lineage that dates back over 270 million years. Its distinctive fan-shaped leaves and dichotomous venation are features that have remained relatively unchanged throughout its long history.
As a basal seed plant, Ginkgo provides insights into the evolution of seed plants before the diversification of modern conifers and flowering plants. Studying Ginkgo helps to understand the origins of seed plant characteristics and the evolutionary history of plant life.
Phylogenetic Toolkit: Software, Databases, and Resources
[Having established the foundational importance of phylogeny, the next logical step involves understanding the methodologies employed to reconstruct evolutionary relationships. This involves a diverse toolkit, ranging from classical comparative methods to cutting-edge molecular techniques. However, understanding the deep branches of the "Tree of Life" would remain an abstract endeavor without the sophisticated computational resources that enable researchers to analyze vast datasets and construct robust phylogenetic trees. This section will explore the essential software, databases, and resources that are indispensable for modern phylogenetic analysis.]
Phylogenetic Software: Reconstructing the Tree of Life
Phylogenetic inference relies heavily on computational power and sophisticated algorithms to analyze genetic and morphological data. Several software packages have emerged as cornerstones in the field, each employing different methodologies and statistical approaches. Understanding their strengths and limitations is crucial for conducting rigorous phylogenetic research.
MrBayes, for instance, is a widely used program that employs Bayesian inference to estimate phylogenetic trees. It utilizes Markov Chain Monte Carlo (MCMC) methods to sample from the posterior probability distribution of trees, allowing researchers to assess the uncertainty in their phylogenetic estimates. MrBayes is particularly valuable for its ability to incorporate complex models of molecular evolution, which can improve the accuracy of phylogenetic inference.
RAxML (Randomized Axelerated Maximum Likelihood) is another popular program, known for its speed and efficiency in performing maximum likelihood (ML) analyses. ML methods aim to find the tree that best explains the observed data, given a particular model of evolution. RAxML's ability to handle large datasets makes it a valuable tool for phylogenomics, where researchers analyze entire genomes to infer evolutionary relationships.
BEAST (Bayesian Evolutionary Analysis Sampling Trees) takes a different approach by explicitly incorporating time into phylogenetic analyses. It uses Bayesian MCMC methods to simultaneously estimate phylogenetic trees and divergence times, allowing researchers to explore the tempo of evolution. BEAST is particularly useful for studying the origins and spread of infectious diseases, as well as for dating evolutionary events in the distant past.
PAUP (Phylogenetic Analysis Using Parsimony*) represents a more traditional approach to phylogenetic inference, emphasizing parsimony, the principle that the simplest explanation is usually the best. While less computationally intensive than Bayesian or ML methods, parsimony can be useful for exploring phylogenetic relationships in smaller datasets or for testing hypotheses generated by other methods.
NCBI: The Genetic Sequence Repository
The National Center for Biotechnology Information (NCBI) serves as a central repository for genetic sequence data. Its databases, such as GenBank, contain an ever-growing collection of DNA and protein sequences from a vast array of organisms.
These databases are invaluable resources for phylogenetic research, providing researchers with the raw materials needed to reconstruct evolutionary relationships. Researchers can access sequences of genes, proteins, and entire genomes, then align these sequences to identify similarities and differences that reflect evolutionary history. NCBI also provides tools for searching, analyzing, and visualizing sequence data, making it an essential resource for any phylogenetist.
TreeBASE: A Repository of Phylogenetic Knowledge
While NCBI stores the raw sequence data, TreeBASE serves as a database specifically dedicated to storing and sharing published phylogenetic trees. This resource allows researchers to access previously published phylogenetic hypotheses, compare their own results with existing knowledge, and explore the diversity of life through an evolutionary lens.
TreeBASE can aid researchers in meta-analyses and comparative studies by allowing them to access a wide range of phylogenetic trees covering various taxa and evolutionary questions. The availability of these curated trees streamlines the research process and promotes collaboration within the phylogenetic community.
Phylogeny in Action: Integrating Evolutionary History into Systematics and Classification
Having established the foundational importance of phylogeny, the next logical step involves understanding how phylogenetic data is employed in real-world applications, specifically within the realms of systematics and classification. These disciplines are fundamentally transformed by the insights that phylogeny provides, offering a robust framework for understanding the intricate web of life. The advent of molecular phylogenetics, in particular, has revolutionized how we approach the classification of organisms and study biodiversity.
Systematics: Unraveling the Tapestry of Life
Systematics, at its core, is the scientific discipline dedicated to classifying organisms and elucidating the evolutionary relationships among them. It aims to construct a hierarchical system that reflects the true history of life, moving beyond superficial similarities to uncover the underlying evolutionary connections. A central goal is to provide a framework to understand the diversity of life.
This pursuit involves not only identifying and describing species but also arranging them into a structured system of classification. Traditionally, systematics relied heavily on morphological characteristics, such as skeletal structures or leaf shapes, for determining relationships.
However, these features can be misleading due to convergent evolution, where unrelated organisms independently evolve similar traits in response to similar environmental pressures.
The Power of Phylogeny in Taxonomic Classification
Phylogeny offers a more robust and accurate approach to systematics by directly examining evolutionary history. Phylogenetic trees, constructed from molecular data or a combination of morphological and molecular evidence, depict the branching patterns of evolution. They reveal which species are most closely related and how different groups have diverged over time.
The integration of phylogenetic data into taxonomic classification has led to significant revisions in our understanding of the relationships between organisms. Taxa are now ideally grouped together based on their shared ancestry, reflecting the historical pattern of evolution rather than simply phenotypic similarity. The principle of monophyly (grouping organisms that share a single common ancestor) becomes paramount.
Redefining Taxonomic Groups: A Case Study
One compelling example of the impact of phylogeny on classification is the reclassification of protists.
Previously, protists were often grouped together as a single kingdom based on their shared characteristic of being eukaryotic organisms that are not plants, animals, or fungi.
However, phylogenetic analyses have revealed that protists are not a monophyletic group. The former "protist" kingdom encompasses diverse lineages that are more closely related to plants, animals, or fungi than they are to other protists. This resulted in the breakup of the "Protista" kingdom, distributing its members across different branches of the eukaryotic tree.
Resolving Evolutionary Relationships
The application of phylogenetics extends beyond simply reorganizing existing taxonomic groups. Phylogeny helps resolve previously uncertain evolutionary relationships and uncover cryptic diversity.
For example, within species or genera that appear morphologically uniform, phylogenetic analyses may reveal distinct evolutionary lineages that warrant recognition as separate species. This is particularly important in conservation efforts, as it ensures that unique evolutionary lineages are not overlooked.
By integrating evolutionary history into systematics and classification, we can gain a deeper and more accurate understanding of the diversity of life and the processes that have shaped it. Phylogeny provides the essential framework for a truly evolutionary-based classification system, moving us closer to a comprehensive and accurate representation of the tree of life.
Video: Basal Taxon: Unlocking Evolutionary Ancestry Secrets
FAQ: Basal Taxon - Unlocking Evolutionary Ancestry Secrets
What exactly is a basal taxon?
A basal taxon is a group in a lineage that diverged early in the evolutionary history of that lineage. It’s essentially the "root" of its branch. It branched off the earliest from the main lineage.
Why is studying a basal taxon important?
Studying a basal taxon gives us insights into the ancestral traits of a larger group. By examining the basal taxon, we can better understand the features that were present in the common ancestor before the lineage diversified.
How does identifying a basal taxon help build phylogenetic trees?
Identifying the basal taxon provides a crucial starting point for phylogenetic trees. It anchors the root of the tree, showing which characteristics likely evolved earliest, and allowing us to trace the evolutionary path to other species.
Can a basal taxon still be complex or "highly evolved"?
Yes, a basal taxon can be just as complex or "highly evolved" as any other organism. "Basal" simply refers to its position in the evolutionary tree, not its complexity. It means that the basal taxon's lineage branched off earliest.
So, the next time you're pondering the great tapestry of life, remember the unsung heroes: the basal taxon. They might not be the flashiest creatures around, but they hold keys to understanding where we all came from, a biological Rosetta Stone if you will! Exploring these earliest branches of the tree of life continues to be a fascinating journey, and who knows what evolutionary secrets the study of basal taxon will unlock next?