Whale Anatomy: Your Comprehensive Whale Guide
Delving into whale anatomía reveals a complex interplay of biological adaptations crucial for the survival of cetaceans in aquatic environments. Marine Biology research institutions, like the Woods Hole Oceanographic Institution, dedicate significant resources to understanding the physiological features of various whale species. Blubber, a specialized adipose tissue, provides insulation, buoyancy, and energy storage, showcasing the remarkable adaptations of whale anatomy. Sonar systems, employed by researchers, allow detailed investigations into the internal structures of whales, furthering our knowledge of their evolutionary adaptations.
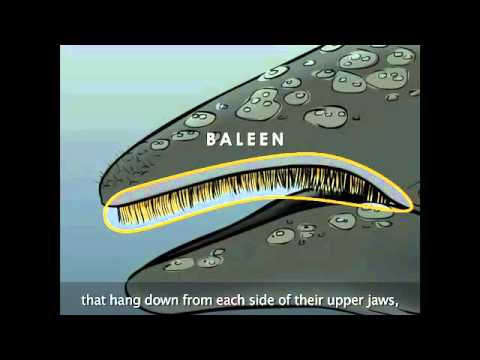
Image taken from the YouTube channel OrcasEnLibertadWeb , from the video titled Whale Anatomy - NOAA OceanToday .
Unveiling the Mysteries of Whale Anatomy and Physiology
Cetaceans, a highly specialized group of marine mammals, present a captivating subject for scientific inquiry. Their evolutionary journey from terrestrial ancestors to fully aquatic beings is a testament to the power of natural selection.
Understanding their anatomy and physiology is not merely an academic exercise. It's a critical necessity for effective conservation and for unraveling the intricate tapestry of life on Earth.
Overview of Cetaceans: Evolution and Diversity
Cetaceans, belonging to the order Cetacea, are characterized by their streamlined bodies, flippers, and horizontal tail flukes. Their evolution traces back roughly 50 million years to land-dwelling ancestors, likely artiodactyls (even-toed ungulates).
Fossil evidence reveals a gradual transition through intermediate forms, showcasing the development of aquatic adaptations. The two primary suborders, Odontoceti (toothed whales) and Mysticeti (baleen whales), represent distinct evolutionary paths.
Odontocetes, such as dolphins, porpoises, and beaked whales, possess teeth and utilize echolocation for hunting. Mysticetes, including baleen whales like the blue whale, humpback whale, and right whale, filter-feed using baleen plates.
The sheer diversity within Cetacea is astonishing. From the colossal blue whale, the largest animal on Earth, to the diminutive vaquita, a critically endangered porpoise, each species occupies a unique ecological niche.
Importance of Understanding Whale Anatomy and Physiology
A comprehensive understanding of whale anatomy and physiology is essential for a multitude of reasons. Crucially, it informs and enhances conservation strategies.
By grasping how whales function, we can better assess the impact of environmental stressors, such as pollution, climate change, and habitat degradation. Knowledge of their physiological limitations and sensitivities allows for more targeted and effective conservation measures.
Furthermore, studying whale anatomy and physiology provides invaluable insights into evolutionary processes. Whales represent a remarkable example of adaptive radiation. Their bodies demonstrate how mammals can successfully colonize and thrive in the marine environment.
Comparative physiology also benefits significantly from cetacean research. Examining the unique adaptations of whales, such as their diving physiology or acoustic communication, can inform our understanding of physiological principles applicable to other species, including humans.
Historical Context: Contributions of Sir Richard Owen and Johannes Peter Müller
The study of whale anatomy and physiology has a rich history, with significant contributions from pioneering scientists. Sir Richard Owen (1804-1892), a renowned British anatomist and paleontologist, made extensive studies of cetacean anatomy.
Owen's detailed descriptions of whale skeletons and organs laid a foundational basis for subsequent research. Johannes Peter Müller (1801-1858), a German physiologist and comparative anatomist, also contributed significantly to our early understanding of cetacean biology.
Müller's work on the circulatory and respiratory systems of marine mammals, including whales, provided crucial insights into their adaptations for aquatic life. These early researchers provided the crucial groundwork that modern cetacean biology continues to build upon.
External Anatomy: Form and Function Above the Surface
Having introduced the broad scope of cetacean biology, we now turn our attention to the immediately observable: the external anatomy of whales. These features, honed by millions of years of aquatic adaptation, provide critical insights into their lifestyle, behavior, and evolutionary history. From the insulating blubber to the propulsive flukes, each structure plays a vital role in the whale's survival.
Blubber: The Multifaceted Adipose Layer
Blubber, the thick layer of adipose tissue beneath the skin, is perhaps one of the most critical adaptations for whales. This layer serves three primary functions: insulation, buoyancy regulation, and energy storage.
Insulation
In the frigid waters that many whale species inhabit, maintaining a stable body temperature is paramount. Blubber acts as an incredibly effective insulator, reducing heat loss to the surrounding environment.
The lipid-rich composition of blubber provides a substantial barrier to thermal conductivity, allowing whales to thrive in otherwise uninhabitable conditions.
Buoyancy Control
The density of blubber, being less dense than water, contributes significantly to a whale's overall buoyancy. This allows whales to passively maintain their position in the water column, reducing the energy expenditure required for staying afloat.
Energy Reserve
During periods of fasting or migration, whales rely on their blubber reserves for sustenance. The blubber stores a vast amount of energy in the form of lipids, providing a readily available source of fuel when food is scarce. The breakdown of blubber also produces metabolic water, aiding in hydration.
Blowhole: The Gateway to Respiration
Whales, being mammals, must breathe air to survive.
The blowhole is the external opening of the respiratory tract, allowing whales to efficiently exchange gases at the surface.
Location and Structure
The location and number of blowholes vary depending on the species. Toothed whales (Odontocetes) possess a single blowhole, while baleen whales (Mysticetes) have two.
This difference reflects variations in the internal anatomy of the nasal passages.
Respiratory Function
The blowhole's primary function is to facilitate rapid inhalation and exhalation at the surface. The muscular flaps surrounding the blowhole create a tight seal when submerged, preventing water from entering the respiratory tract.
Species-Specific Variations
The morphology of the blowhole can be a useful tool for species identification. For example, the North Atlantic right whale has a distinctive V-shaped blowhole spray pattern.
These variations reflect subtle differences in nasal passage anatomy and respiratory mechanics.
Flippers: Steering and Maneuvering in the Deep
Whale flippers are homologous to the forelimbs of terrestrial mammals, demonstrating their evolutionary origin.
The internal bone structure of the flipper retains the pentadactyl (five-digit) limb pattern, a characteristic feature of tetrapods.
Bone Structure
Despite their modified shape, flippers contain the same basic bones as a human arm: humerus, radius, ulna, carpals, metacarpals, and phalanges.
However, these bones are shortened and flattened, providing a rigid but flexible structure for maneuvering in water.
Steering and Control
Flippers are primarily used for steering, turning, and maintaining stability in the water. The broad surface area of the flippers allows whales to generate lift and drag, enabling precise control over their movements. Some species also use their flippers for communication and social interactions.
Flukes: The Engine of Propulsion
The flukes, or tail fins, are the primary propulsive structures in whales. These powerful appendages generate thrust through vertical oscillations, propelling the whale through the water.
Structure and Composition
Unlike the dorsal fin, which is supported by cartilage, flukes are composed entirely of dense connective tissue. This provides the strength and flexibility needed to withstand the forces generated during swimming.
Propulsion Mechanism
The flukes generate thrust by moving up and down in a rhythmic motion. As the flukes move through the water, they create a pressure difference that propels the whale forward. The angle and frequency of fluke strokes can be adjusted to control speed and direction.
Dorsal Fin: Stabilization and Recognition
The dorsal fin, located on the back of the whale, serves primarily as a stabilizer, preventing rolling and improving hydrodynamic efficiency.
Stabilization Function
By increasing the whale's surface area, the dorsal fin enhances stability and reduces drag. This is particularly important for fast-swimming species that need to maintain a streamlined body position.
Species Identification
The size, shape, and markings on the dorsal fin can vary significantly between species and even individuals.
These variations provide a valuable tool for researchers studying whale populations and behavior.
Internal Anatomy: Feeding and Skeletal Structures
Having explored the external features that define a whale's interaction with its environment, we now delve deeper, venturing into the realm of internal anatomy. These structures, often unseen but no less crucial, reveal the intricate mechanisms that support their aquatic existence. From specialized feeding apparatus to skeletal vestiges of their terrestrial past, the internal anatomy of whales offers a fascinating window into their evolutionary journey and ongoing adaptation.
Baleen Plates: Filter-Feeding Mechanism in Baleen Whales
Baleen whales, the gentle giants of the ocean, possess a unique feeding adaptation: baleen plates. These plates, numbering in the hundreds, hang from the upper jaw, forming a sieve-like structure.
Structure and Composition
Baleen plates are composed of keratin, the same protein that forms our fingernails and hair. Each plate is triangular, with a broad base embedded in the upper jaw and a fringed, bristle-like edge that filters food from the water.
The plates are arranged in parallel rows, creating an efficient filtering system. The size, shape, and number of baleen plates vary among different baleen whale species, reflecting their specific dietary preferences.
Filter-Feeding Mechanism
The mechanism of filter-feeding is a remarkable display of efficiency. Baleen whales take in large gulps of water containing their prey, primarily krill, small fish, or zooplankton.
They then use their tongue to force the water out through the baleen plates, trapping the prey inside. The whale then swallows the accumulated food, obtaining the energy needed for their massive size and long migrations. Different techniques are employed, such as skim feeding (right whales), gulp feeding (rorquals), and bottom suction (gray whales).
Teeth: Structure and Function in Toothed Whales
In contrast to baleen whales, toothed whales (Odontocetes) possess teeth, which they use for capturing and processing prey. The structure and function of these teeth vary depending on the species and their dietary habits.
Tooth Structure and Types
Toothed whale teeth are typically homodont (uniform in shape) and monophyodont (one set of teeth throughout their lives). The teeth are conical and designed for grasping and holding prey.
Some species, like dolphins, have numerous small, sharp teeth for catching fish, while others, like sperm whales, have fewer, larger teeth primarily in the lower jaw, which are used for securing squid.
Function in Capturing and Processing Prey
Toothed whales use their teeth to seize their prey, preventing escape. They often swallow their prey whole, without chewing, relying on powerful stomach acids to break down the food. The positioning and size of the teeth are crucial for effective prey capture.
Rorqual Folds (Ventral Grooves): Engulfment Feeding Adaptation
Rorqual whales, a group of baleen whales that includes the blue whale and humpback whale, possess a unique anatomical feature called rorqual folds, also known as ventral grooves. These folds are longitudinal pleats of skin and tissue that run along the throat and chest of the whale.
Location and Structure
The rorqual folds extend from the lower jaw to the navel. They are highly elastic and can expand significantly, allowing the whale to engulf enormous amounts of water and prey in a single gulp.
Engulfment Feeding
During engulfment feeding, the rorqual whale swims towards a dense patch of prey and opens its mouth wide, taking in a massive volume of water and krill or small fish. The rorqual folds expand to accommodate this influx, increasing the whale's oral cavity exponentially.
After engulfing the water, the whale contracts its throat muscles to expel the water through the baleen plates, trapping the prey inside. This adaptation allows rorquals to efficiently exploit dense food patches, consuming vast quantities of prey in a short amount of time.
Vestigial Pelvic Bones: Evolutionary Significance
One of the most compelling pieces of evidence for whale evolution is the presence of vestigial pelvic bones. These bones are small, non-functional remnants of the pelvis and hind limbs of their terrestrial ancestors.
Location and Structure
The vestigial pelvic bones are located deep within the whale's body, near the tail. They are not connected to the vertebral column, and they do not support any limbs.
They typically consist of a small, rod-shaped bone, sometimes accompanied by a rudimentary femur. The size and shape of the vestigial pelvic bones vary among different whale species.
Evolutionary Significance
The presence of vestigial pelvic bones provides strong evidence that whales evolved from land-dwelling mammals. These bones are homologous to the pelvic bones and hind limbs of other mammals, indicating a shared ancestry. The vestigial nature of these bones highlights the evolutionary transition from terrestrial locomotion to an entirely aquatic lifestyle.
Mandible: Lower Jaw Structure and Function
The mandible, or lower jaw, plays a critical role in the feeding and sensory capabilities of whales. Its structure and function vary depending on whether the whale is a toothed or baleen whale.
Mandible Structure
In toothed whales, the mandible is typically long and slender, with teeth embedded in sockets along its length. The shape and size of the mandible vary depending on the species' diet and hunting strategies.
In baleen whales, the mandible is large and robust, providing support for the baleen plates. The two halves of the mandible are connected by a flexible symphysis, allowing for a wide gape during feeding.
Role in Feeding and Sound Reception
The mandible is essential for capturing and processing prey in toothed whales. In some species, such as dolphins, the mandible is also involved in sound reception.
Fat-filled cavities within the mandible transmit sound vibrations to the inner ear, allowing the whale to detect and localize underwater sounds. This adaptation is particularly important for echolocation, a crucial hunting strategy for many toothed whales.
Hyoid Bone: Support for Tongue and Larynx
The hyoid bone is a small, U-shaped bone located in the throat of whales. It is not directly connected to any other bones, but it is suspended by ligaments and muscles.
Location and Function
The hyoid bone is situated between the mandible and the larynx, providing support for the tongue and larynx. Its primary function is to anchor the tongue muscles, facilitating tongue movement during feeding and vocalization.
Support for Tongue and Larynx
The hyoid bone plays a crucial role in supporting the tongue, enabling whales to manipulate food and swallow. It also supports the larynx, which contains the vocal cords.
In some whale species, the hyoid bone is involved in sound production, helping to modulate the frequency and intensity of their calls. The complex structure of the hyoid bones reflects their importance in whale communication.
Sensory Organs: Perception in the Aquatic Realm
Having explored the external features that define a whale's interaction with its environment, we now delve deeper, venturing into the realm of internal anatomy. These structures, often unseen but no less crucial, reveal the intricate mechanisms that support their aquatic existence. From specialized feeding apparatus to skeletal frameworks designed for hydrodynamic efficiency, the internal anatomy of whales is a testament to evolutionary adaptation. Now, we turn our attention to how whales perceive their surroundings, exploring the sensory organs that enable them to navigate and thrive in their aquatic world.
Whales, having transitioned from terrestrial ancestors, have undergone significant sensory adaptations to effectively perceive their underwater environment. While vision and olfaction, crucial for many terrestrial mammals, are less prominent in whales, other senses have evolved to take precedence. Among these remarkable adaptations, the melon stands out as a pivotal organ for echolocation, allowing toothed whales to "see" with sound.
The Melon: An Acoustic Lens
The melon, a structure composed primarily of lipids (fats), is located in the forehead region of toothed whales. Its size and shape vary among species, reflecting differences in their echolocation capabilities and habitat preferences. Compositionally, the melon is not a homogenous mass; rather, it contains complex arrangements of lipids, varying in density and distribution.
These intricate arrangements are critical for its function.
The melon serves as an acoustic lens, focusing and directing the outgoing sound waves emitted by the whale. These sound waves, generated in the nasal passages, pass through the melon, which shapes them into a focused beam.
The specific lipid composition and architecture of the melon influence the frequency and directionality of the sound beam, enabling the whale to control and optimize its echolocation signals. By manipulating the shape of the melon, whales can adjust the focus of their sound beam, allowing them to scan their environment and pinpoint the location of objects with remarkable accuracy.
Echolocation is not simply about emitting sounds; it is a sophisticated process of interpreting the returning echoes. When the sound waves encounter an object, such as prey or a navigational hazard, they bounce back as echoes. These echoes are received by the whale, primarily through specialized fats in the mandible (lower jaw).
The returning signals are then transmitted to the inner ear and processed by the brain, providing the whale with detailed information about the object's size, shape, distance, and density. The melon, therefore, plays a critical role not only in sound production but also in the overall efficiency and precision of the echolocation process.
Other Sensory Adaptations
While the melon and echolocation represent a pinnacle of sensory adaptation in whales, other senses also contribute to their perception of the aquatic world.
Hearing Adaptations
Whales have evolved remarkable hearing adaptations for underwater sound reception. Unlike terrestrial mammals, sound travels faster and farther in water, making it an ideal medium for communication and environmental awareness. Whales lack external ears; instead, they receive sound vibrations through specialized fats in their lower jawbones (mandible).
These vibrations are then transmitted to the inner ear, which is isolated from the skull to reduce interference from bone conduction. The inner ear of whales is highly sensitive, allowing them to detect a wide range of frequencies and to discern subtle differences in sound that can provide valuable information about their environment.
Tactile Reception
Touch is another important sense for whales, particularly for close-range interactions and social bonding. While the distribution of tactile receptors across the whale's body is not fully understood, studies have shown that they are particularly sensitive around the head, mouth, and genital regions.
These tactile receptors allow whales to detect subtle changes in water pressure and temperature, as well as to engage in tactile communication with other individuals. Calves, in particular, rely on tactile interactions with their mothers for reassurance and guidance. The sense of touch, therefore, plays a crucial role in the social lives and overall well-being of whales.
Physiological Systems: Adapting to an Aquatic Lifestyle
Having explored the sensory organs that allow whales to perceive their environment, we now turn our attention inward, examining the physiological systems that sustain their lives beneath the waves. These systems, honed by millions of years of evolution, represent a remarkable suite of adaptations to the challenges of an aquatic existence.
Respiratory System: Breath-Holding Champions
Whales are renowned for their ability to hold their breath for extended periods and dive to great depths. This remarkable feat is made possible by several key adaptations within their respiratory system.
Adaptations for Deep Diving
Whales possess a significantly higher blood volume per body mass than terrestrial mammals, allowing for increased oxygen storage. Their lungs, while not proportionally larger, are highly elastic and collapse completely during deep dives. This expels air from the alveoli, preventing nitrogen from dissolving into the bloodstream and thus avoiding decompression sickness (the "bends").
Furthermore, whales exhibit selective vasoconstriction, reducing blood flow to non-essential organs during dives, thus conserving oxygen for the brain, heart, and muscles. They also have a higher concentration of myoglobin, an oxygen-binding protein, in their muscles. This allows for greater oxygen storage within muscle tissue.
Lung Structure and Function
Whale lungs are structured to maximize oxygen uptake efficiency. The alveoli, the tiny air sacs where gas exchange occurs, have a high surface area. This design facilitates rapid and efficient transfer of oxygen from the air into the bloodstream.
Whales also exhibit a unique breathing pattern, exchanging a large percentage of the air in their lungs with each breath. This rapid and efficient ventilation allows them to quickly replenish oxygen stores before diving again.
Circulatory System: Oxygen Delivery Under Pressure
The circulatory system plays a critical role in oxygen transport and distribution throughout the whale's body, particularly during prolonged dives.
Oxygen Storage Adaptations
As mentioned, whales have several key adaptations for oxygen storage. These include a higher blood volume, increased myoglobin concentration in muscles, and the ability to store oxygen in the spleen. The spleen acts as a reservoir of red blood cells, which can be released into circulation during dives to boost oxygen-carrying capacity.
Heart and Blood Vessel Characteristics
The whale heart is a powerful pump, capable of maintaining circulation under extreme pressure. Their arteries are highly elastic, allowing them to expand and contract to regulate blood flow and withstand the pressure changes associated with diving. During a dive, the heart rate slows dramatically (bradycardia), reducing oxygen consumption and conserving energy.
Digestive System: Processing a Marine Diet
The digestive system of whales is adapted to efficiently process a diet of marine organisms, ranging from tiny krill to large fish and squid.
Stomach Compartments and Digestion
Whales possess a multi-chambered stomach. This design aids in the breakdown of tough or chitinous exoskeletons of prey. The first chamber typically stores food. Succeeding chambers secrete digestive enzymes and acids to break down proteins, fats, and carbohydrates.
Pancreas and Liver Functions
The pancreas produces enzymes that aid in the digestion of proteins, fats, and carbohydrates. The liver plays a critical role in metabolizing fats and detoxifying harmful substances. Both organs are essential for maintaining metabolic balance and extracting maximum nutritional value from their diet.
Digestive Differences Between Species
The digestive systems of baleen whales and toothed whales reflect their different diets. Baleen whales, which filter feed on krill and other small organisms, have a simpler digestive system compared to toothed whales, which consume larger, more complex prey. Toothed whales have sharper teeth that enable them to capture and consume a wider variety of prey. This also requires a digestive system that can process proteins, fats, and other complex compounds.
Nervous System: Intelligence and Echolocation
The nervous system of whales is highly developed. It supports their complex social behavior, intelligence, and specialized sensory abilities.
Brain Structure and Function
Whales possess large and complex brains. These brains exhibit a high degree of encephalization, particularly in areas associated with social cognition, communication, and sensory processing. Studies have revealed complex folding patterns in the cerebral cortex, a feature associated with higher intelligence.
Echolocation: Mechanism and Significance
Toothed whales have developed echolocation, a biological sonar system. This system allows them to navigate and hunt in dark or murky waters. They emit high-frequency clicks and then interpret the echoes that bounce back from objects in their environment. The melon, a specialized structure in the forehead, focuses and directs these sound waves. Echolocation provides detailed information about the size, shape, distance, and movement of potential prey, making it an indispensable tool for survival.
Musculoskeletal System: Power and Grace in Motion
The musculoskeletal system of whales is highly adapted for efficient swimming.
Adaptations for Swimming
The streamlined body shape of whales reduces drag, allowing them to move through the water with minimal resistance. Their powerful tail flukes generate thrust. The flippers provide steering and stability. Tendons also play a huge role. Stiff tendons reduce the amount of muscular energy required for these animals to swim.
Bone and Muscle Structure
Whale bones are dense and strong, providing support for their massive bodies. Their muscles are arranged in layers and are highly vascularized, allowing for efficient oxygen delivery. The vertebral column is flexible, enabling the undulating movements that propel them through the water. The caudal peduncle, the region just before the tail flukes, is particularly muscular, providing the power needed for sustained swimming and rapid bursts of speed.
Whale Species and Comparative Anatomy: A Closer Look at Diversity
Having explored the physiological systems that allow whales to thrive in their aquatic environment, it becomes clear that the selective pressures acting upon different whale lineages have resulted in an incredible array of anatomical and physiological adaptations. Examining individual whale species, within a comparative framework, provides a powerful lens through which to understand these variations.
This section delves into specific case studies, showcasing how distinct anatomical and physiological features allow various whale species to thrive in unique ecological niches. Each example highlights the intimate relationship between form and function, illuminating the diverse strategies that have allowed whales to conquer the world's oceans.
Blue Whale ( Balaenoptera musculus ): Anatomical Extremes
The blue whale, Balaenoptera musculus, stands as the largest animal on Earth, representing the epitome of anatomical scale. Its sheer size dictates a number of unique physiological challenges and adaptations.
Its heart, for example, can weigh upwards of 180 kg, and its circulatory system must effectively pump blood to every corner of its immense body. This requires an efficient vascular network and adaptations for maintaining blood pressure even at great depths.
Blue whales feed almost exclusively on krill, utilizing their baleen plates to filter vast quantities of these tiny crustaceans from the water. The enormous size of their mouths and the highly specialized structure of their baleen are critical adaptations for this feeding strategy.
Humpback Whale (Megaptera novaeangliae): Unique Physical Traits
Humpback whales, Megaptera novaeangliae, are renowned for their elaborate songs and acrobatic displays. These behaviors are underpinned by a suite of unique physical traits.
Their exceptionally long pectoral fins, which can reach up to one-third of their body length, play a crucial role in maneuvering and generating thrust, particularly during bubble-net feeding. These fins, supported by elongated bone structures, provide remarkable agility.
Humpback whales also possess unique tubercles, or knobs, on their heads. These tubercles contain sensory hairs that may play a role in detecting prey or navigating through the water.
Gray Whale (Eschrichtius robustus): Migration Patterns
Gray whales, Eschrichtius robustus, are renowned for undertaking some of the longest migrations of any mammal. Their annual journey from Arctic feeding grounds to warmer breeding lagoons in Baja California and back again, covers thousands of kilometers.
This remarkable feat requires efficient energy storage and precise navigation skills. Gray whales accumulate vast reserves of blubber to fuel their migration, relying on internal cues and magnetic sense to return to their breeding grounds year after year.
Their adaptation to benthic feeding – scooping up sediment from the ocean floor to filter out invertebrates – is another distinguishing characteristic, shaping their snout morphology and baleen structure.
Sperm Whale (Physeter macrocephalus): Deep-Diving Adaptations
The sperm whale, Physeter macrocephalus, is a master of the deep. It boasts the ability to dive to depths of over 2,000 meters in search of squid.
This necessitates a suite of remarkable physiological adaptations, including a high myoglobin concentration in its muscles, which allows for efficient oxygen storage. Sperm whales are also able to slow their heart rate and selectively shunt blood flow to vital organs during deep dives.
Perhaps the most distinctive feature of the sperm whale is its spermaceti organ, a large cavity in its head filled with a waxy substance. The function of this organ is debated but likely involves buoyancy control, sound production, or both.
Orca/Killer Whale (Orcinus orca): Hunting Strategies
Orcas, Orcinus orca, also known as killer whales, exhibit a remarkable diversity of hunting strategies, reflecting their position as apex predators in many marine ecosystems. Different populations specialize in hunting different prey, including fish, seals, and even other whales.
Their sophisticated social structures and cooperative hunting behaviors are facilitated by their complex communication system, which relies on a variety of vocalizations. Their hunting techniques exhibit a high degree of cultural transmission.
The anatomy of orcas reflects their predatory lifestyle. Their powerful jaws and sharp teeth are ideal for capturing and subduing prey, while their streamlined bodies allow for efficient swimming at high speeds.
Beluga Whale (Delphinapterus leucas): Arctic Adaptations
Beluga whales, Delphinapterus leucas, are uniquely adapted to life in the Arctic. Their thick blubber layer provides insulation against the frigid waters, while their lack of a dorsal fin reduces heat loss and allows them to navigate under ice.
Belugas are also highly vocal, using a complex repertoire of sounds to communicate with each other in the murky Arctic waters. They possess a flexible neck, unique among whales, which allows them to maneuver more easily in ice-filled environments.
Furthermore, belugas exhibit the capacity to change colour from grey to pure white as they mature, which allows them to seamlessly blend in with their icy surroundings.
Narwhal (Monodon monoceros): The Tusk and Its Function
The narwhal, Monodon monoceros, is best known for its elongated tusk, which is actually a modified tooth that protrudes from the upper jaw of males (and occasionally females).
The function of the tusk has long been a source of speculation. Current research suggests that it may serve as a sensory organ, allowing narwhals to detect changes in water temperature and salinity. The tusk is highly innervated and possesses a porous structure that allows for the uptake of water.
The tusk also plays a role in social signaling and male-male competition. Narwhals are found in Arctic waters and, like belugas, possess adaptations that enable them to endure the harsh and frigid conditions.
Minke Whale (Balaenoptera acutorostrata): Feeding Habits and Adaptations
Minke whales, Balaenoptera acutorostrata, are among the smallest baleen whales, found in oceans across the world. They display a range of feeding behaviors, including gulp feeding, skimming, and bottom suction.
Their relatively small size and agile movements allow them to exploit a variety of prey sources, from small schooling fish to krill. Minke whales possess a streamlined body and powerful tail flukes that allow for efficient propulsion through the water.
Their ability to perform high-speed pursuits of prey in various aquatic environments has allowed them to survive in many of the Earth’s oceanic ecosystems.
Research Methodologies: Unveiling Whale Secrets
Having explored the physiological systems that allow whales to thrive in their aquatic environment, it becomes clear that the selective pressures acting upon different whale lineages have resulted in an incredible array of anatomical and physiological adaptations. Examining individual specimens through scientific research methodologies, traditional and modern, has been vital in understanding these complex organisms.
Traditional Techniques: The Foundation of Cetacean Research
Before the advent of sophisticated imaging technologies, our understanding of whale anatomy and physiology relied heavily on direct observation and manual techniques. Dissection and histology formed the cornerstones of this foundational research.
Dissection: Detailed Anatomical Studies
Dissection, the meticulous process of carefully cutting into and separating tissues and organs, provides invaluable insights into the macroscopic structure of whales. This technique, while seemingly basic, allows researchers to observe the precise arrangement of muscles, bones, and organs within the animal's body.
Through careful dissection, scientists can measure organ sizes, identify anomalies, and map the complex networks of blood vessels and nerves. The insights gleaned from dissection studies have been crucial in understanding the functional relationships between different anatomical structures.
Histology: Microscopic Tissue Analysis
While dissection reveals the macroscopic organization of the whale body, histology provides a window into the microscopic world of tissues and cells. This technique involves preparing thin sections of tissue, staining them with specific dyes, and examining them under a microscope.
Histological analysis allows researchers to identify cell types, examine tissue structures, and detect abnormalities that may be indicative of disease or environmental stress. By studying the microscopic features of whale tissues, we can gain a deeper understanding of their function and how they are affected by various factors.
Modern Imaging Techniques: Non-Invasive Exploration
Modern imaging techniques have revolutionized the study of whale anatomy and physiology, offering non-invasive ways to visualize internal structures and processes. These methods have significantly expanded our ability to study these magnificent animals without causing harm.
Radiography (X-rays): Bone Structure Analysis
Radiography, or X-ray imaging, uses electromagnetic radiation to penetrate tissues and create images of dense structures, such as bones. This technique is particularly useful for studying the skeletal anatomy of whales, allowing researchers to identify fractures, bone deformities, and other skeletal abnormalities.
X-rays can also be used to estimate the age of a whale by examining the growth rings in their teeth or earplugs.
Computed Tomography (CT Scans): 3D Anatomical Imaging
Computed tomography (CT) scanning takes X-ray imaging to the next level by generating cross-sectional images of the body. These images can then be reconstructed to create detailed three-dimensional (3D) models of internal organs and tissues.
CT scans provide a much more comprehensive view of whale anatomy than traditional X-rays, allowing researchers to visualize the precise shape, size, and location of internal structures.
Magnetic Resonance Imaging (MRI): Soft Tissue Studies
Magnetic resonance imaging (MRI) uses strong magnetic fields and radio waves to create images of soft tissues, such as muscles, ligaments, and internal organs. MRI is particularly useful for studying the brain and nervous system of whales, as it can provide detailed images of these structures without the need for invasive procedures.
Ultrasound: Real-Time Imaging
Ultrasound imaging uses high-frequency sound waves to create real-time images of internal structures. This technique is non-invasive and relatively inexpensive, making it a valuable tool for studying whale physiology in the field.
Ultrasound can be used to monitor heart function, assess reproductive status, and examine the health of internal organs.
Advanced Technologies: Pushing the Boundaries of Knowledge
Advanced technologies are continually pushing the boundaries of what we can learn about whale anatomy and physiology. These cutting-edge techniques offer unprecedented insights into the cellular, acoustic, and genetic aspects of whale biology.
Electron Microscopy: Cellular Structure Analysis
Electron microscopy uses beams of electrons to create highly magnified images of cells and tissues. This technique allows researchers to visualize the ultrastructure of cells, including their organelles and other internal components.
Electron microscopy has been used to study the structure of whale skin, blubber, and other tissues, providing insights into their unique adaptations for life in the marine environment.
Sonar and Hydrophones: Acoustic Studies
Whales are highly vocal animals, and sound plays a crucial role in their communication, navigation, and foraging. Sonar and hydrophones are used to study whale acoustics, allowing researchers to record and analyze whale sounds, determine their source, and map their distribution.
These techniques have been instrumental in understanding whale communication, social behavior, and echolocation abilities.
Genetic Analysis: Evolutionary Relationships
Genetic analysis has revolutionized our understanding of whale evolution and relationships. By analyzing the DNA of different whale species, researchers can reconstruct their evolutionary history and identify the genes that are responsible for their unique adaptations.
Genetic studies have revealed that whales are most closely related to hippopotamuses, and they have provided insights into the genetic basis of their aquatic adaptations. These methodologies are indispensable for conservation efforts, identifying distinct populations and mitigating potential genetic bottlenecks.
Fields of Study and Key Concepts: Interdisciplinary Perspectives
Having explored the research methodologies used to unveil the secrets of whale anatomy and physiology, it becomes clear that the study of these magnificent creatures is inherently interdisciplinary. Examining individual specimens through dissection, employing advanced imaging, and running genetic analysis all combine to inform the core concepts that govern our understanding of whale biology. Understanding these connections requires navigating multiple scientific disciplines, synthesizing knowledge to appreciate the full complexity of cetacean life.
Cetology: The All-Encompassing Science of Whales
At the heart of whale studies lies cetology, the branch of marine mammal science dedicated entirely to cetaceans. This broad field encompasses everything from their behavior and ecology to their evolutionary history and conservation status. Cetology serves as the umbrella under which all other specialized disciplines converge when focusing on whales.
Cetologists often collaborate with experts from diverse backgrounds to gain a holistic understanding of their subjects. This includes not only biologists but also oceanographers, geneticists, and even social scientists, especially when investigating the impact of human activities on whale populations. The need for interdisciplinary collaboration in the field of cetology is paramount to conservation efforts, making it a cornerstone for protecting and understanding these animals.
Comparative Anatomy: Illuminating Evolutionary Relationships
Comparative anatomy plays a crucial role in understanding the evolutionary relationships between whale species and their terrestrial ancestors. By carefully examining the anatomical structures of different whales and comparing them to those of other mammals, scientists can trace the modifications that have occurred over millions of years as whales transitioned from land to water.
The presence of vestigial structures, such as the aforementioned pelvic bones, provides compelling evidence of this evolutionary journey. Furthermore, comparing the bone structure of whale flippers to that of the pentadactyl limb found in many terrestrial vertebrates reveals their shared ancestry.
Physiology: Unraveling the Mysteries of Bodily Functions
Physiology, the study of how living organisms function, is essential for comprehending the remarkable adaptations that allow whales to thrive in the marine environment. Understanding the mechanisms behind their deep-diving abilities, their unique thermoregulation strategies, and their efficient oxygen utilization requires in-depth knowledge of their physiological systems.
Studies of whale physiology often involve sophisticated techniques, such as monitoring heart rate, measuring blood oxygen levels, and analyzing metabolic rates. Such methods provide insights into the intricate processes that enable these animals to survive in extreme conditions.
Evolutionary Biology: Tracing the Path of Cetacean Development
Evolutionary biology provides the framework for understanding how whales have diversified over time and how natural selection has shaped their unique characteristics. By studying the fossil record, analyzing genetic data, and examining the anatomical and physiological traits of extant species, evolutionary biologists can reconstruct the evolutionary history of whales.
Key to this understanding is the concept of phylogeny, or the evolutionary relationships among different whale lineages. Phylogenetic analyses help to understand how different whale species are related to one another and how they evolved.
Biomechanics: Analyzing Movement and Mechanics
Biomechanics applies the principles of mechanics to the study of living organisms, offering insights into how whales move through the water and interact with their environment. Analyzing the hydrodynamics of their body shape, the mechanics of their flukes, and the forces involved in their swimming strokes allows researchers to understand the energetic efficiency of their movements.
Understanding the biomechanics of whale locomotion also has practical applications, such as informing the design of underwater vehicles and developing more effective conservation strategies. These studies enhance our understanding of marine propulsion and maneuvering.
Echolocation: A Sonic Sixth Sense
Echolocation is a remarkable sensory adaptation found in toothed whales, allowing them to navigate and hunt in dark or murky waters. Understanding the mechanism of echolocation, from the production of sound waves in the melon to their reception and interpretation by the brain, requires knowledge of anatomy, physiology, and acoustics.
This sonar-like system allows toothed whales to "see" their surroundings by analyzing the echoes of the sounds they emit, giving them a distinct advantage in their aquatic environment. The study of echolocation continues to be a fascinating area of research, shedding light on the sensory capabilities of these remarkable animals.
Adaptation: The Key to Survival
Adaptation is a central concept in whale biology, referring to the process by which organisms evolve traits that enhance their survival and reproduction in a specific environment. Understanding the various adaptations that whales have developed, from their streamlined body shape to their specialized respiratory systems, is crucial for comprehending their ecological success.
Adaptations can be anatomical, physiological, or behavioral, and they often represent a compromise between different selective pressures. For example, a whale's large size may provide protection from predators but also increase its energy demands.
Video: Whale Anatomy: Your Comprehensive Whale Guide
Frequently Asked Questions: Whale Anatomy
What are the key external differences between baleen whales and toothed whales?
Baleen whales possess baleen plates for filtering food, while toothed whales have teeth for catching prey. The blowhole also differs: baleen whales have two, whereas toothed whales have a single blowhole. Understanding these variations is crucial in appreciating whale anatomía.
How do whale bones differ from land mammal bones?
Whale bones are often denser than land mammal bones to counteract buoyancy in water. Also, hind limbs are vestigial or entirely absent, and forelimbs are modified into flippers. This adaptation is a key feature of whale anatomía.
What is the purpose of blubber in whales?
Blubber serves three vital functions: insulation to maintain body temperature in cold waters, energy storage for lean times, and contribution to buoyancy. This specialized tissue is a crucial element of whale anatomía.
How does a whale's respiratory system allow it to hold its breath for extended periods?
Whales have a higher blood volume and a greater concentration of myoglobin in their muscles compared to land mammals, allowing them to store more oxygen. This impressive adaptation allows efficient oxygen utilization. Understanding this element of whale anatomía clarifies their diving capabilities.
So, there you have it! A whirlwind tour of whale anatomy. Hopefully, you've gained a new appreciation for these magnificent creatures and a better understanding of what makes whale anatomia so unique. Now go forth and impress your friends with your newfound cetacean knowledge!