Water Thermal Conductivity: Secrets Revealed!
Understanding water thermal conductivity is crucial across numerous scientific and engineering disciplines. The National Institute of Standards and Technology (NIST) maintains extensive databases containing the values for the thermal properties of fluids, including water, which directly impacts the accuracy of simulations. Heat transfer efficiency, a key attribute related to water's ability to conduct heat, is fundamental in designing effective cooling systems. Furthermore, the work of Joseph Fourier, a pioneer in heat transfer analysis, provided the mathematical framework for understanding how water thermal conductivity governs temperature distribution within a system.
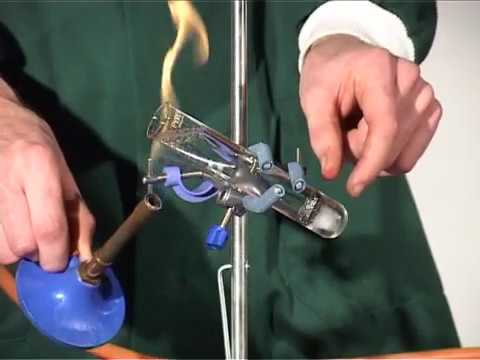
Image taken from the YouTube channel Scott Nowell , from the video titled 33. Experiment to show that water is a poor conductor of heat .
Water, seemingly simple in its chemical formula (H₂O), possesses a wealth of complex and often surprising properties. Among these is its ability to conduct heat, a characteristic known as thermal conductivity. This seemingly mundane property underpins a vast array of natural phenomena and technological applications, making it a subject of considerable scientific and engineering interest.
From regulating Earth's climate to enabling efficient cooling in power plants, water's thermal conductivity plays a more significant role in our lives than we often realize.
What is Water Thermal Conductivity?
Thermal conductivity, in general, refers to a material's ability to transfer heat. Specifically, water thermal conductivity is a measure of how readily heat flows through water due to a temperature difference. It is quantified as the amount of heat that passes through a unit area of water per unit time for a unit temperature gradient. Water has a relatively high thermal conductivity compared to other common liquids, a fact that's crucial for many natural and industrial processes.
Why Water's Thermal Conductivity Matters
The importance of water's thermal conductivity extends far beyond the laboratory.
In everyday life, it governs how quickly a pot of water heats up on the stove or how effectively a radiator warms a room.
Industrially, it's critical for the design of heat exchangers in power plants, chemical processing facilities, and HVAC systems. In the realm of climate, water's thermal conductivity influences ocean currents and the distribution of heat around the globe.
Consider the implications:
- Efficient heat dissipation in electronic devices.
- Optimization of geothermal energy extraction.
- Regulation of body temperature through sweating.
These are just a few examples of how a deep understanding of water's thermal conductivity is essential.
Exploring the Thermal Landscape of Water
This article aims to delve into the multifaceted world of water thermal conductivity. We will explore the fundamental principles that govern this property, uncover the factors that influence it, examine the techniques used to measure it, and showcase its diverse applications.
By the end of this exploration, you should have a comprehensive understanding of water thermal conductivity and its significance in the world around us.
The Fundamentals: How Water Conducts Heat
Water's ability to conduct heat efficiently is a cornerstone of numerous natural and industrial processes. But what are the underlying principles that govern this seemingly simple phenomenon?
Understanding how water conducts heat requires exploring the key factors at the molecular level that dictate its thermal properties.
Temperature's Influence on Thermal Conductivity
The relationship between temperature and water's thermal conductivity is not always straightforward. While it generally increases with temperature, the trend is not linear across the entire temperature spectrum.
At lower temperatures, the increase in thermal conductivity is more pronounced. This is because as temperature rises, water molecules gain kinetic energy, leading to more frequent and energetic collisions.
These collisions facilitate the transfer of heat through the liquid. However, at higher temperatures, the rate of increase in thermal conductivity tends to slow down.
This can be attributed to various factors, including changes in water's density and molecular structure.
Empirical data and graphical representations are essential for illustrating these non-linear effects and providing a quantitative understanding of the temperature-thermal conductivity relationship.
Molecular Structure and Hydrogen Bonding
Water's unique molecular structure (H₂O) and its extensive hydrogen bonding network are pivotal to its thermal conductivity. Each water molecule consists of two hydrogen atoms and one oxygen atom, arranged in a bent configuration.
This polarity allows water molecules to form hydrogen bonds with each other, creating a dynamic, three-dimensional network.
These hydrogen bonds act as pathways for efficient heat transfer. Vibrational energy, which manifests as heat, can propagate rapidly through the hydrogen-bonded network.
The strength and density of these hydrogen bonds are temperature-dependent, influencing the overall thermal conductivity. The more extensive and robust the hydrogen bonding network, the more effectively water can conduct heat.
Heat Transfer Mechanisms in Water
Heat can be transferred through matter via three primary mechanisms: conduction, convection, and radiation. In the case of water, all three mechanisms can play a role, but conduction is the dominant mode at the molecular level.
- Conduction involves the transfer of heat through direct molecular contact, where kinetic energy is passed from one molecule to another.
- Convection involves heat transfer through the movement of fluids (liquids or gases).
- Radiation involves the transfer of heat through electromagnetic waves and is less significant within the bulk of water.
Within water, heat is primarily conducted through the rapid propagation of vibrational energy along the hydrogen-bonded network. Convection becomes more relevant when considering larger volumes of water subject to temperature gradients, leading to density differences and fluid motion.
Thermal Diffusivity Explained
Thermal diffusivity is a measure of how quickly a material can adjust its temperature to changes in its surroundings. It is intrinsically linked to thermal conductivity, representing the ratio of thermal conductivity to the product of density and specific heat capacity.
α = k / (ρ Cp)
Where:
- α = Thermal Diffusivity
- k = Thermal Conductivity
- ρ = Density
- Cp = Specific Heat Capacity
A higher thermal diffusivity indicates that a material can rapidly dissipate or absorb heat.
Water's thermal diffusivity reflects its ability to quickly equilibrate its temperature within a given volume. A high thermal conductivity and relatively high specific heat capacity contribute to its thermal diffusivity.
Specific Heat Capacity (Cp) and Density: The Supporting Cast
Specific heat capacity (Cp) is the amount of heat required to raise the temperature of a unit mass of a substance by one degree Celsius (or Kelvin). Water has a relatively high specific heat capacity, meaning it can absorb a significant amount of heat without experiencing a large temperature change.
Density, defined as mass per unit volume, also plays a crucial role. It affects the amount of substance available to conduct heat within a given space.
Together, specific heat capacity and density, in conjunction with thermal conductivity, determine water's overall heat transfer characteristics.
A high specific heat capacity allows water to store a considerable amount of thermal energy, while its density influences the rate at which this energy can be transported via conduction. These properties make water an exceptionally effective medium for heat transfer and temperature regulation in both natural and engineered systems.
Water's remarkable capacity to conduct heat, dictated by its molecular structure and influenced by temperature, isn't the whole story. External factors can also play a significant role, subtly yet definitively altering its thermal conductivity. These influences, often overlooked, can be crucial in specific applications and environments. Understanding these hidden variables provides a more complete picture of water's thermal behavior.
Hidden Influences: Factors Affecting Thermal Conductivity
Beyond temperature and molecular structure, water's thermal conductivity is susceptible to external factors. Pressure and the presence of impurities can significantly alter its thermal properties.
Pressure Effects
Pressure, often an understated variable, can impact water's thermal conductivity. Under typical, everyday conditions, the effect is minimal.
However, in scenarios involving extreme pressure variations, such as deep-sea environments or specialized industrial processes, the changes become noticeable. The influence of pressure is complex and depends on the specific temperature range.
Pressure-Induced Density Changes
Increased pressure forces water molecules closer together, increasing its density. This denser packing facilitates more frequent molecular collisions.
The result is enhanced thermal conductivity under these conditions. The magnitude of this effect depends on the magnitude of the pressure change.
Competing Factors at High Pressures
At extremely high pressures, the relationship can become more complex.
Water's molecular structure can be altered, possibly hindering the efficiency of heat transfer. Empirical data is essential to fully understand the relationship between extreme pressures and thermal conductivity.
Impact of Impurities
Pure water, as studied in laboratory settings, is rarely found in real-world applications. Dissolved solids, gases, and other impurities are almost always present and inevitably influence thermal conductivity.
The type and concentration of these impurities determine the extent of their effect.
Dissolved Solids
Dissolved salts and minerals can alter water's thermal conductivity. Generally, the presence of dissolved solids increases thermal conductivity.
This is because many solids have higher thermal conductivities than water. The ions introduced by these solids can also affect the hydrogen bonding network.
However, the magnitude of the increase is often relatively small at typical concentrations.
Dissolved Gases
Dissolved gases, such as oxygen, nitrogen, and carbon dioxide, have a more complex impact. The effect depends on the gas's solubility and its thermal properties relative to water.
In most cases, dissolved gases slightly decrease thermal conductivity.
This is because gases generally have lower thermal conductivities than liquids. Higher concentrations of dissolved gases can create microscopic bubbles that inhibit heat transfer.
Organic Contaminants
Organic contaminants, stemming from industrial waste or natural sources, can also influence thermal conductivity. The effects vary widely depending on the specific contaminant.
Some organic compounds may slightly increase thermal conductivity, while others can significantly decrease it.
Complex interactions between the organic molecules and water's hydrogen bonding network are often at play. Detailed analysis is needed to fully understand the impact of specific organic contaminants.
Practical Implications
The presence of impurities has significant practical implications for applications like cooling systems and heat exchangers. Even trace amounts of contaminants can affect the efficiency of heat transfer.
Regular monitoring and control of water purity are crucial for maintaining optimal system performance. Understanding the impact of specific impurities is essential for developing effective water treatment strategies.
Measuring the Unseen: Techniques and Challenges
Having examined the hidden influences that subtly alter water's thermal conductivity, the question becomes: how do scientists accurately quantify this elusive property? Measurement of water's thermal conductivity is far from straightforward. It demands careful consideration of experimental design, meticulous execution, and a thorough understanding of potential error sources.
Overview of Measurement Techniques
Several techniques have been developed to determine water's thermal conductivity, each relying on different principles and possessing its own strengths and limitations. Here, we will explore the most commonly employed methods.
The Hot Wire Method
The hot wire method is a widely used technique for measuring the thermal conductivity of fluids, including water. It involves immersing a thin, electrically heated wire (acting as both a heat source and a temperature sensor) into the water sample.
A constant or transient current is passed through the wire, generating heat. By monitoring the temperature change of the wire over time, the thermal conductivity of the surrounding water can be determined.
The underlying principle is based on the relationship between the heat generated by the wire, the temperature gradient established in the water, and the water's ability to conduct heat away from the wire. This method is valued for its relative simplicity and accuracy.
Transient Hot Wire Method
A variation of the hot wire method, the transient hot wire method, offers improved accuracy and faster measurement times.
Instead of maintaining a constant current, a short pulse of heat is applied to the wire. The temperature rise is monitored over a brief period, typically just a few seconds.
This transient approach minimizes the effects of convection, which can interfere with accurate measurements.
By analyzing the temperature response of the wire during this transient period, the thermal conductivity of the water can be precisely determined. The transient hot wire method is particularly well-suited for measuring the thermal conductivity of liquids, including water.
Other Measurement Techniques
While the hot wire and transient hot wire methods are prominent, other techniques exist. These include methods based on guarded hot plates and laser flash analysis.
These alternative approaches may be more suitable for specific applications or sample types, but are generally less common for routine measurements of water's thermal conductivity.
Challenges and Considerations
Despite the availability of various measurement techniques, accurately determining water's thermal conductivity presents several challenges. Careful attention to experimental design and standardized procedures is crucial for minimizing errors and ensuring reliable results.
Sources of Error
Potential sources of error include:
- Convection: Natural convection currents within the water can interfere with heat transfer, leading to inaccurate measurements.
- Radiation: Radiative heat transfer from the hot wire can also introduce errors, particularly at higher temperatures.
- Contact Resistance: Thermal contact resistance between the sensor and the water can impede heat flow and affect the accuracy of the measurements.
- Calibration: Proper calibration of the measurement apparatus is essential for ensuring accurate results.
Importance of Experimental Design
Careful experimental design is critical for minimizing these errors.
- Sample Purity: The purity of the water sample must be carefully controlled, as impurities can significantly affect thermal conductivity.
- Temperature Control: Precise temperature control is necessary to minimize temperature gradients and ensure accurate measurements.
- Apparatus Geometry: The geometry of the measurement apparatus must be carefully designed to minimize convection and radiation effects.
Standardized Procedures
Adherence to standardized procedures is crucial for ensuring the reproducibility and comparability of measurements. Organizations such as the American Society for Testing and Materials (ASTM) have developed standardized methods for measuring thermal conductivity. Following these standards helps minimize errors and ensures the reliability of the results.
Real-World Impact: Applications of Water Thermal Conductivity
Having explored the hidden influences that subtly alter water's thermal conductivity, the question becomes: how does an understanding of this property translate into tangible benefits across diverse fields? The answer lies in the myriad applications where water's ability to conduct heat plays a critical role, impacting everything from industrial processes to sustainable energy solutions.
Relevance in Applications
Water thermal conductivity is not merely an academic curiosity; it is a fundamental parameter that governs the performance of countless systems. Its influence is particularly pronounced in applications involving heat transfer, such as heat exchangers, cooling systems, and geothermal energy extraction.
Heat Exchangers
Heat exchangers, ubiquitous in various industries, rely on water's thermal conductivity to efficiently transfer heat between two fluids. These devices are essential components in power plants, chemical processing facilities, and HVAC systems, where they facilitate the heating or cooling of process streams. For instance, in a power plant, water is used to cool the steam exiting the turbine, allowing it to condense back into water and be pumped back to the boiler. A higher thermal conductivity of the cooling water directly translates to a more efficient condensation process, leading to increased power generation and reduced energy consumption.
Cooling Systems
Cooling systems, ranging from the radiators in automobiles to the massive cooling towers in data centers, depend on water's ability to absorb and dissipate heat. In data centers, which generate immense amounts of heat, water cooling is often employed to maintain optimal operating temperatures for the servers. By circulating water through heat sinks attached to the servers, the excess heat is transferred away, preventing overheating and ensuring reliable performance. Here, understanding water thermal conductivity is crucial for designing efficient cooling loops.
Geothermal Energy
Geothermal energy harnesses the Earth's internal heat to generate electricity or provide direct heating. Water or another fluid is circulated through underground reservoirs, where it absorbs heat from the surrounding rocks. This heated water is then brought to the surface and used to drive turbines or provide heat for buildings. The efficiency of geothermal energy extraction is directly related to the thermal conductivity of the water used as the heat transfer medium.
Optimizing System Performance
The ability to manipulate and optimize water's thermal conductivity, even to a small degree, can lead to significant improvements in system performance and efficiency. Several strategies can be employed to achieve this.
Additives and Nanofluids
Adding certain additives or nanoparticles to water can enhance its thermal conductivity. This approach has led to the development of nanofluids, which are engineered fluids containing nanoparticles suspended in a base fluid like water. Nanofluids have shown promising results in improving the heat transfer performance of various systems, including heat exchangers and cooling systems.
System Design and Configuration
Careful system design and configuration can also play a vital role in optimizing heat transfer. This includes selecting appropriate materials for heat exchanger surfaces, optimizing flow rates, and minimizing thermal resistance within the system. A thorough understanding of water's thermal conductivity allows engineers to make informed decisions about these design parameters, leading to improved performance.
Monitoring and Control
Implementing monitoring and control systems can help maintain optimal operating conditions and ensure efficient heat transfer. By continuously monitoring parameters such as temperature, flow rate, and pressure, these systems can automatically adjust operating parameters to maximize performance and prevent overheating or other problems.
By carefully considering these factors and leveraging our understanding of water's thermal conductivity, we can unlock its full potential and create more efficient, sustainable, and reliable systems for a wide range of applications. The real-world impact of this seemingly simple property is profound, shaping the way we generate power, cool our homes, and harness the Earth's resources.
Video: Water Thermal Conductivity: Secrets Revealed!
Water Thermal Conductivity: Frequently Asked Questions
Here are some common questions about water thermal conductivity, explained simply.
What exactly is water thermal conductivity?
Water thermal conductivity is a measure of how well water transfers heat. Simply put, it describes how quickly heat can move through water from a warmer area to a cooler area. This property is important in many natural processes and engineering applications.
Why does water thermal conductivity matter?
It's crucial for various reasons. For example, it affects how lakes and oceans regulate temperature, impacting aquatic life. In engineering, it's important for designing efficient cooling systems and heat exchangers. Understanding water thermal conductivity helps us predict and control heat transfer in these situations.
How does temperature affect water thermal conductivity?
Generally, water thermal conductivity increases with temperature, up to a certain point. Warmer water molecules move faster, facilitating more efficient heat transfer. However, this relationship isn't linear and can become complex near the boiling point.
Is water thermal conductivity different at different pressures?
Yes, pressure does influence water thermal conductivity, but the effect is typically smaller compared to temperature. Higher pressure generally increases the density of water, which can slightly enhance its ability to conduct heat. However, for most everyday applications, the pressure effect is negligible.