Telescope Chromatic Aberration Meaning: The Ultimate Guide
Understanding telescope chromatic aberration meaning is crucial for any astronomer or telescope user seeking clear, high-quality images. Chromatic aberration, a phenomenon directly related to light dispersion, presents itself as color fringing around objects viewed through a telescope. Optical design, a core field within physics, tackles the challenge of minimizing this aberration. Refracting telescopes are particularly susceptible to chromatic aberration, which scientists and engineers work constantly to understand and mitigate. By understanding the fundamentals, and how it impacts optical instruments, you can improve your experience.
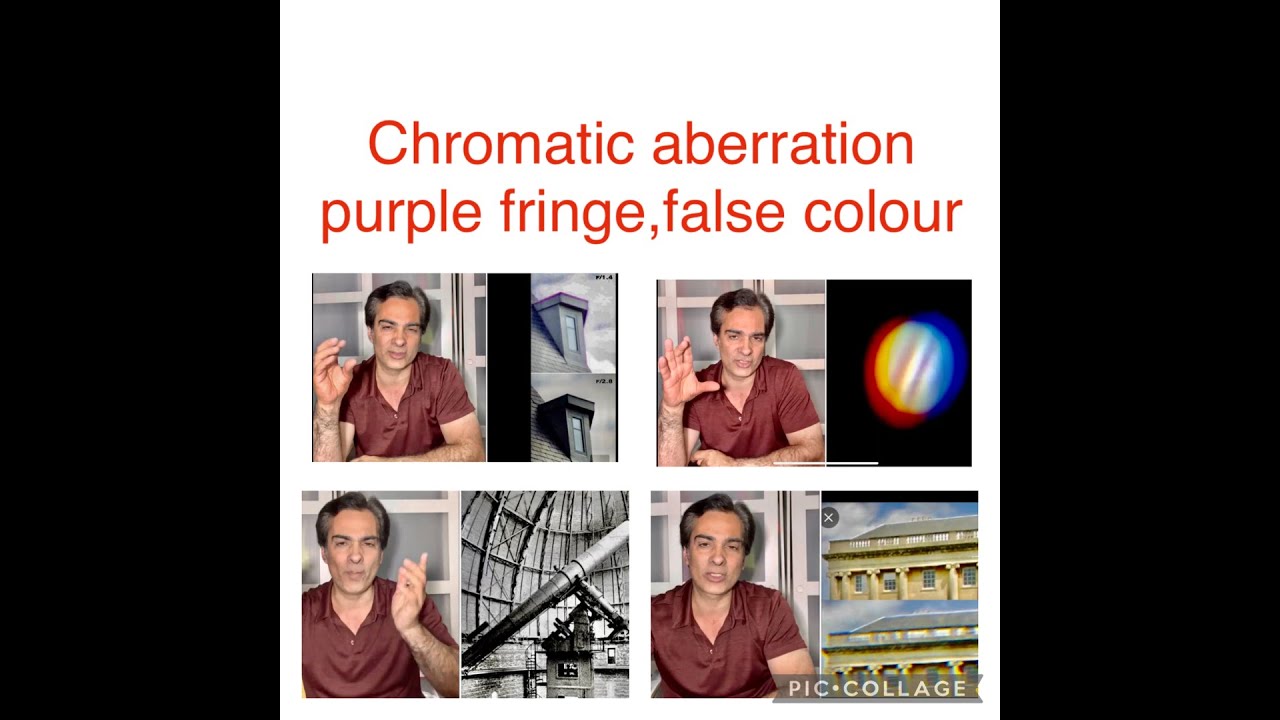
Image taken from the YouTube channel JoeJaguar City Smartphone Astronomy , from the video titled what is chromatic aberration? #astronomy #telescope #space #nasa #saturn #meade #optics #celestron .
Humankind has always gazed at the heavens, driven by an insatiable curiosity about the cosmos. Telescopes, our mechanical eyes to the universe, have opened up breathtaking vistas, revealing celestial wonders previously unseen.
From the shimmering rings of Saturn to the swirling nebulae light-years away, these instruments transform faint specks of light into tangible realities.
However, the quest for ever-clearer and more detailed astronomical images is ongoing. Image clarity is paramount in unlocking the full potential of telescopic observation. Without it, our ability to study and appreciate the universe is fundamentally limited.
The Allure of Astronomical Observation
Telescopes allow us to witness events that occurred millions or even billions of years ago. They are time machines, peering into the distant past and helping us understand the origins of the universe.
The profound beauty and sheer scale of the cosmos, brought to life through telescopes, inspire awe and a sense of our place within the grand cosmic tapestry.
The Importance of Image Quality
In astronomical viewing, image quality dictates the level of detail we can observe. Sharp, clear images are essential for discerning subtle features on planets, resolving faint galaxies, and accurately measuring the properties of distant stars.
Poor image quality obscures these details, hindering scientific discovery and diminishing the visual experience.
Chromatic Aberration: An Optical Obstacle
One of the most common and frustrating obstacles to achieving optimal image quality in telescopes is chromatic aberration. This optical defect manifests as unwanted color fringing around bright objects, blurring details and reducing overall sharpness.
Chromatic aberration, if left uncorrected, can significantly degrade the viewing experience. It transforms potentially stunning celestial images into disappointing, color-smeared approximations. Understanding and mitigating chromatic aberration is therefore essential for any serious astronomer or telescope user.
One of the most common and frustrating obstacles to achieving optimal image quality in telescopes is chromatic aberration. This optical defect manifests as unwanted color fringing around bright objects, blurring details and reducing overall sharpness. To truly grasp how this distortion arises, we need to first explore the fundamental properties of light itself.
Understanding the Fundamentals of Light and Refraction
Light, the very medium through which we perceive the universe, behaves in fascinating ways. One of its most crucial characteristics is its ability to bend, a phenomenon known as refraction. Refraction is the key to understanding how lenses work, and, therefore, is essential for grasping the origins of chromatic aberration.
What is Refraction?
Refraction is the bending of light as it passes from one transparent medium to another, such as from air into glass. This bending occurs because light travels at different speeds in different media.
Imagine a beam of light as a marching band approaching a muddy field at an angle. The side of the band that hits the mud first will slow down, causing the entire band to pivot slightly. Similarly, when light enters glass, it slows down, causing it to change direction. This change in direction is refraction.
The Role of Wavelength in Refraction
Now, here’s where things get interesting. Not all light bends the same amount. The degree to which light is refracted depends on its wavelength, which we perceive as different colors.
Blue light, with its shorter wavelength, bends more than red light, with its longer wavelength. This difference in bending is crucial for understanding chromatic aberration.
Defining the Light Spectrum
White light, such as sunlight, isn't a single color, but a mixture of all the colors of the rainbow. These colors, arranged by their wavelengths, form the light spectrum.
Think of a rainbow after a rain shower. It’s a stunning visual representation of the light spectrum, caused by sunlight being refracted and reflected by water droplets in the atmosphere. The colors always appear in the same order because each color is bent by a specific amount, according to its wavelength.
Connecting Light Dispersion to the Splitting of White Light
The separation of white light into its component colors is called dispersion. Prisms famously demonstrate this effect; when white light enters a prism, the different colors are refracted at different angles, creating a beautiful spectrum.
This same principle applies to lenses. Because different colors of light are refracted differently, they don't all converge at the same focal point after passing through a lens. This is the root cause of chromatic aberration, resulting in those unwanted color fringes we see in our telescopic images.
One might wonder, with this differential bending of light, what the practical implications are for viewing the cosmos through a telescope. This brings us to the core of the issue: chromatic aberration.
Chromatic Aberration: A Deep Dive into Color Fringing
Chromatic aberration is an optical defect that arises from the failure of a lens to focus all colors to the same convergence point.
It's the reason why, instead of crisp, clear images of celestial objects, you might see unwanted color halos around stars or planets.
This phenomenon is especially noticeable in refracting telescopes, which rely on lenses to gather and focus light.
Defining Chromatic Aberration: The Rainbow's Unwanted Appearance
At its most basic, chromatic aberration manifests as color fringing or blurring in an image.
Imagine viewing a bright star through a telescope.
Instead of seeing a sharp, white point of light, you might observe a halo of color surrounding the star, typically with blue or purple on one side and red or yellow on the other.
This occurs because the lens bends different colors of light at slightly different angles, causing them to focus at different points along the optical axis.
The result is a blurred image with distracting color artifacts.
The Impact on Image Quality: Loss of Sharpness and Clarity
Chromatic aberration degrades image quality in several significant ways:
-
Reduced Sharpness: Color fringing blurs fine details, making it difficult to resolve small features on planets or faint stars in nebulae.
-
Decreased Contrast: The unwanted colors reduce the contrast between bright and dark areas in the image, washing out subtle details.
-
Eye Strain: The distorted colors and lack of sharpness can lead to eye strain and discomfort during extended viewing sessions.
Ultimately, chromatic aberration diminishes the overall viewing experience, preventing you from fully appreciating the beauty and detail of the night sky.
Where Does It Occur? Refracting Telescopes and Lens Design
Chromatic aberration is most prevalent in refracting telescopes. These telescopes use lenses as their primary objective to gather and focus light.
The design of a single-element lens inherently leads to this chromatic distortion, as we've established that each wavelength of light bends differently as it passes through.
While reflecting telescopes, which use mirrors instead of lenses, are not immune to other types of aberrations, they are inherently free from chromatic aberration.
The Role of Focal Length: A Critical Parameter
The severity of chromatic aberration is related to the focal length of the lens.
A shorter focal length typically results in more noticeable chromatic aberration.
This is because the steeper curves of a short focal length lens cause greater dispersion of light.
Conversely, a longer focal length lens, with its gentler curves, tends to exhibit less chromatic aberration, although it can still be present.
Therefore, understanding the relationship between focal length and chromatic aberration is crucial in telescope selection and design.
Chromatic aberration, as we've seen, significantly impacts image quality in telescopes. But the story of how we came to understand and overcome this challenge is a fascinating journey through the history of science, involving some of the most brilliant minds ever to gaze at the stars.
A Historical Perspective: The Scientists Who Tackled Chromatic Aberration
The quest to eliminate chromatic aberration is interwoven with the history of optics itself. Early telescope designs were plagued by this issue, leading scientists to grapple with the fundamental nature of light and its interaction with lenses. Two figures stand out prominently in this narrative: Isaac Newton and John Dollond.
Isaac Newton's Initial Understanding and Challenges with Chromatic Aberration
Isaac Newton, a towering figure in scientific history, made significant contributions to our understanding of light and optics. Through his famous prism experiments, he demonstrated that white light is composed of a spectrum of colors.
This discovery, while groundbreaking, led him to a rather pessimistic conclusion regarding refracting telescopes. Newton believed that chromatic aberration was an inherent property of lenses and could not be corrected. He reasoned that since lenses refract different colors of light at different angles, it would be impossible to bring all colors to a single focal point.
Based on this conviction, Newton shifted his focus to reflecting telescopes, which use mirrors instead of lenses to gather and focus light.
Mirrors, unlike lenses, do not suffer from chromatic aberration because they reflect all colors of light at the same angle. Newton's reflecting telescope, a testament to his ingenuity, became a popular alternative to refractors for a time.
However, the limitations of early mirror technology, such as difficulty in producing perfectly shaped and reflective surfaces, eventually led to a renewed interest in refractors, but with a crucial difference: a focus on correcting chromatic aberration.
The Contributions of John Dollond in the Development of Achromatic Lenses
While Newton believed chromatic aberration was insurmountable, John Dollond, an English optician, proved him wrong. Dollond challenged Newton's assertion by demonstrating that it was possible to combine lenses made of different types of glass to reduce chromatic aberration.
Dollond's key innovation was the achromatic lens, which consists of two or more lenses with different refractive indices. Typically, this involves combining a convex lens made of crown glass with a concave lens made of flint glass.
Crown glass has a relatively low refractive index and low dispersion, while flint glass has a higher refractive index and higher dispersion. By carefully selecting the curvatures and materials of the lenses, Dollond found that he could bring two colors of light (typically red and blue) to the same focal point.
While the achromatic lens did not eliminate chromatic aberration entirely, it significantly reduced it, resulting in much sharper and clearer images compared to single-lens telescopes.
Dollond's invention revolutionized telescope design and paved the way for the development of larger and more powerful refracting telescopes. His work marked a pivotal moment in the history of optics, demonstrating that scientific dogma could be challenged and overcome through ingenuity and experimentation.
A telescope marred by chromatic aberration presents a frustrating view, scattering the starlight you long to see clearly. Fortunately, the persistent efforts of scientists and engineers have yielded remarkable technological solutions. These advancements, primarily in lens design and optical coatings, have dramatically reduced, and in some cases virtually eliminated, chromatic aberration, allowing for stunningly clear astronomical observations.
Technological Solutions: Correcting Chromatic Aberration Through Lens Design
The quest for sharper, clearer images in telescopes has driven significant innovation in lens technology. Two primary types of lenses, achromatic and apochromatic, stand at the forefront of this battle against chromatic aberration. Supplementing these lens designs are advanced optical coatings, playing a crucial role in maximizing light transmission and further enhancing image quality.
Achromatic Lenses: A Dual Approach
The achromatic lens represents a significant early step in correcting chromatic aberration. These lenses are constructed from two separate pieces of glass with different refractive indices. Typically, one element is a convex lens made of crown glass, which has relatively low dispersion. The second is a concave lens made of flint glass, which exhibits higher dispersion.
By carefully selecting and combining these glasses, lens designers can bring two different wavelengths (typically red and blue) of light to a common focal point. This significantly reduces the color fringing seen in simpler lenses.
While achromatic lenses represent a substantial improvement, they don't eliminate chromatic aberration entirely. A small amount of color fringing, typically a yellowish or greenish hue, can still be visible, particularly around bright objects. These residual aberrations are known as secondary spectrum.
Apochromatic Lenses: Precision Correction
For truly demanding applications where the highest possible image quality is essential, apochromatic lenses provide superior performance. Apochromatic lenses (often referred to as "APO" lenses) take chromatic correction a step further than achromatic lenses.
These lenses are designed to bring three wavelengths of light (red, green, and blue) to a single focal point. This virtually eliminates the secondary spectrum, resulting in images with exceptional sharpness and color fidelity.
Material Innovations in Apochromatic Lenses
Achieving this level of correction often requires the use of specialized, exotic glasses, such as extra-low dispersion (ED) glass or fluorite crystal. These materials exhibit unique optical properties that allow for finer control over light dispersion.
Design Complexity in Apochromatic Lenses
The construction of apochromatic lenses is also more complex, often involving three or more lens elements. These elements are precisely shaped and positioned to minimize aberrations across the entire visible spectrum.
The result is an image that is virtually free of color fringing, revealing subtle details and vibrant colors with remarkable clarity.
The Role of Optical Coatings
While lens design is crucial for correcting chromatic aberration, optical coatings play an equally important role in maximizing image brightness and contrast. These coatings are thin layers of material applied to the surface of the lens elements. They are designed to reduce reflections and increase the amount of light that passes through the lens.
Minimizing Reflections
Each time light passes through a lens surface, a small percentage is reflected away. This not only reduces the amount of light reaching the eye but also can create unwanted glare and ghost images. Optical coatings minimize these reflections, allowing more light to pass through the lens and improving overall image brightness.
Enhancing Contrast and Sharpness
By reducing reflections, optical coatings also improve image contrast and sharpness. This is because stray light, caused by reflections, can wash out details and reduce the clarity of the image. Coatings help maintain the purity of the light path, resulting in images with greater detail and definition.
Multi-Layer Coatings
Modern optical coatings often consist of multiple layers of different materials, each precisely engineered to optimize light transmission at specific wavelengths. These multi-layer coatings can dramatically improve the performance of a lens, particularly in challenging lighting conditions.
Chromatic Aberration Beyond Telescopes: A Wider Optical Reality
While chromatic aberration is often discussed in the context of telescopes, its effects are not limited to astronomical observation. This optical imperfection can manifest in a variety of optical instruments, impacting image quality across a broad spectrum of applications. Understanding its presence and effects in these other contexts is crucial for anyone working with or relying on optical systems.
Cameras: A Common Ground for Chromatic Aberration
Chromatic aberration is a well-known issue in photography. Camera lenses, like telescope lenses, are susceptible to this phenomenon due to the refractive properties of glass. When light passes through a lens, different wavelengths are bent at slightly different angles, leading to color fringing around high-contrast edges in an image.
Lateral and Longitudinal Chromatic Aberration in Photography
In photography, chromatic aberration typically manifests in two primary forms: lateral (or transverse) and longitudinal (or axial).
-
Lateral chromatic aberration appears as color fringes along the edges of the image, with colors shifting outwards from the center. This type is more pronounced toward the edges of the frame.
-
Longitudinal chromatic aberration results in different colors focusing at different distances from the lens. This leads to some areas of the image appearing sharp in one color channel but blurry in others.
Mitigating Chromatic Aberration in Camera Lenses
Camera lens manufacturers employ various techniques to minimize chromatic aberration.
These methods include using specialized glass types with low dispersion, incorporating multiple lens elements to correct for color fringing, and applying advanced optical coatings to improve light transmission. Software correction in post-processing is also commonly used to further reduce the visible effects of chromatic aberration.
Microscopes: Precision Optics and Aberration Control
Microscopes, essential tools in scientific research and diagnostics, also face the challenge of chromatic aberration. High-magnification microscopy demands extremely sharp and clear images, making aberration control paramount.
Chromatic aberration in a microscope can distort the appearance of cellular structures and other microscopic details, hindering accurate observation and analysis.
Apochromatic microscope objectives, similar in concept to apochromatic telescope lenses, are designed to bring three or more colors into focus at the same point. This provides significantly improved color correction compared to simpler achromatic objectives.
Other Optical Instruments
Chromatic aberration can also affect the performance of other optical instruments, such as binoculars, spectrometers, and even the human eye. In each case, the degree to which it impacts image quality depends on the design and quality of the optical components involved.
The broader presence of chromatic aberration underscores its fundamental nature as a challenge in optics. While technological advancements continue to mitigate its effects, understanding its origins and characteristics remains essential for achieving optimal image quality across a diverse range of applications.
Chromatic aberration can be a frustrating issue, but thankfully, it’s often identifiable with careful observation. Let's delve into how to spot these unwanted color artifacts in your telescope and what they mean for your viewing experience.
Identifying and Assessing Chromatic Aberration in Your Telescope
Spotting chromatic aberration requires a keen eye and a methodical approach. The telltale signs often appear as unwanted color fringes around bright objects. Understanding these signs is the first step in assessing the severity of the problem.
Spotting Color Fringing: A Practical Guide
Color fringing is the most obvious indicator of chromatic aberration. Here's how to look for it:
-
Observe bright objects: Stars, planets, or even the lunar limb are excellent targets.
-
Focus carefully: Ensure your telescope is precisely focused. Chromatic aberration becomes more apparent with a sharp focus.
-
Look for color halos: Check for red, blue, or purple halos surrounding the object. These halos are the dispersed colors that haven’t converged to a single focal point.
-
Examine high-contrast areas: The edges of lunar craters or planetary features are prime locations to observe color fringing.
Assessing the Severity
Once you've identified color fringing, it's important to determine how much it affects your viewing experience.
A slight fringe might be tolerable, especially at lower magnifications. However, significant color fringing can severely degrade image quality, especially at higher magnifications.
Other Indicators of Chromatic Aberration
While color fringing is the most common sign, other indicators can also point to chromatic aberration:
-
Soft Images: Even when properly focused, images may appear soft or lack sharpness.
-
Color Bleeding: Colors may appear to bleed into adjacent areas, especially around bright objects.
-
Reduced Contrast: The overall contrast of the image might seem lower than expected.
The Impact on Resolution: How Chromatic Aberration Blurs the Details
Chromatic aberration directly impacts the resolution of your telescope. Resolution refers to the ability to distinguish fine details in an image.
When different colors are not focused at the same point, the image becomes blurred, reducing the level of detail you can see.
-
Loss of Fine Detail: Subtle features on planets or faint stars may become difficult or impossible to discern.
-
Reduced Sharpness: The overall sharpness of the image is compromised, leading to a less satisfying viewing experience.
Mitigating the Effects
While you might not be able to eliminate chromatic aberration entirely, you can take steps to minimize its impact.
-
Use lower magnifications: Chromatic aberration tends to be more pronounced at higher magnifications.
-
Employ filters: Certain filters can block specific wavelengths of light, reducing color fringing.
-
Consider a different eyepiece: Some eyepieces are designed to minimize chromatic aberration.
By carefully observing your telescope's images and understanding the signs of chromatic aberration, you can better assess its impact on your viewing experience and take steps to mitigate its effects. A clear, sharp image is the ultimate goal, and knowing how to identify and address chromatic aberration is a crucial step in achieving it.
Video: Telescope Chromatic Aberration Meaning: The Ultimate Guide
Telescope Chromatic Aberration: Frequently Asked Questions
Here are some frequently asked questions to further clarify the concept of telescope chromatic aberration.
What exactly is telescope chromatic aberration?
Telescope chromatic aberration is a type of optical defect that occurs in refracting telescopes. It happens because the lens bends different colors of light at slightly different angles. This causes colors to focus at different points, resulting in a blurry image with colored fringes around bright objects.
Why is telescope chromatic aberration more common in refracting telescopes?
Refracting telescopes use lenses to focus light. Lenses are more susceptible to chromatic aberration than mirrors, which are used in reflecting telescopes. The degree to which a lens bends light varies depending on the light's wavelength (color), leading to the color separation effect.
Can telescope chromatic aberration be completely eliminated?
While it's challenging to eliminate telescope chromatic aberration entirely in refracting telescopes, it can be significantly reduced. This is often done by using achromatic lenses, which are made from multiple lens elements with different refractive indices, effectively correcting the color separation. ED (extra-low dispersion) glass also helps reduce telescope chromatic aberration meaning clearer images.
How does telescope chromatic aberration affect the viewing experience?
Telescope chromatic aberration degrades image quality by introducing colored halos around objects, making details harder to see. It is especially noticeable when observing bright objects like the Moon or planets. Understanding telescope chromatic aberration meaning helps in choosing appropriate telescopes and/or filters to reduce its effects.