Sugar's Journey: Where Does Sugar Enter Blood?

Following digestion, the small intestine emerges as the primary site where sugar enters the blood, marking a critical juncture in nutrient absorption. The enzyme sucrase, produced in the small intestine, plays a pivotal role in breaking down sucrose into simpler sugars like glucose and fructose. Glucose, an essential monosaccharide, then crosses the intestinal lining into the bloodstream, initiating its distribution throughout the body. This process is closely monitored and regulated by hormones such as insulin, secreted by the pancreas, ensuring that blood sugar levels remain within a balanced range.
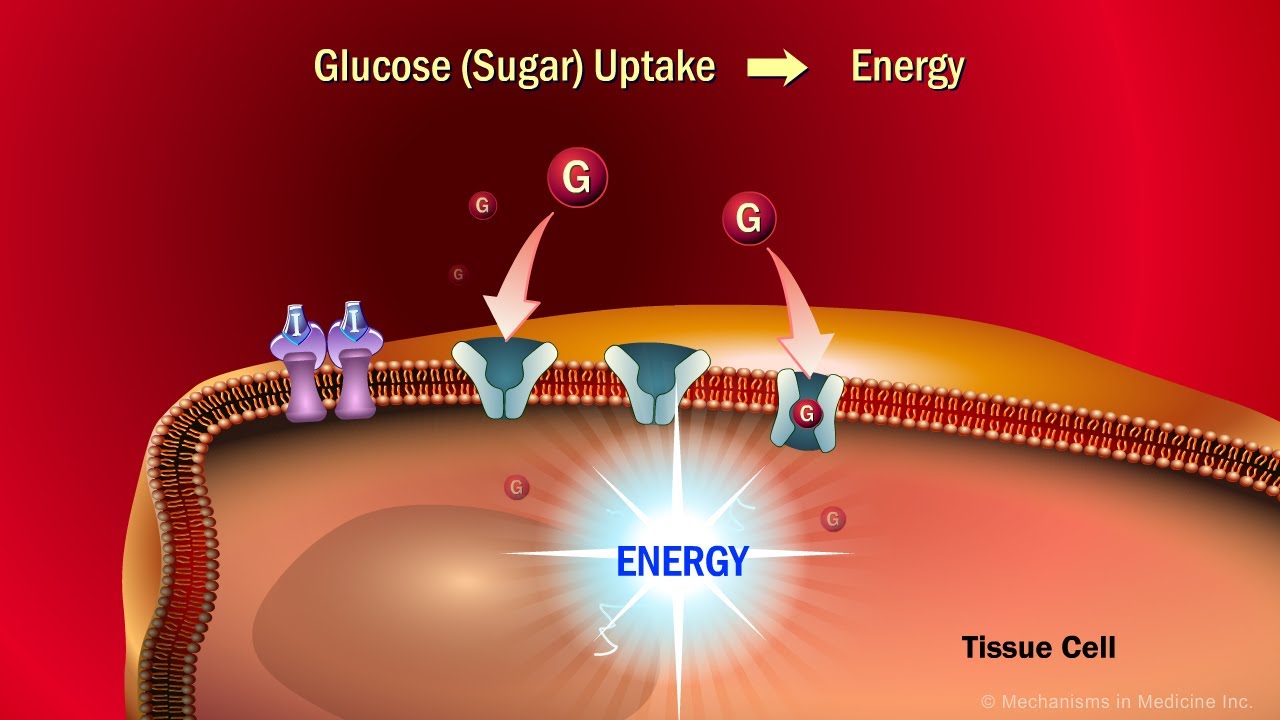
Image taken from the YouTube channel Mechanisms in Medicine , from the video titled The Role of Insulin in the Human Body .
The Symphony of Sugar: Energy, Absorption, and Regulation
Glucose, often simplified as "blood sugar," is far more than a mere measurement on a lab report. It is the lifeblood of our cellular energy system, the primary fuel that powers every action, thought, and physiological process within the human body.
From the simplest muscle contraction to the complex computations of the brain, glucose is the indispensable energy currency that keeps us alive and functioning.
Understanding how our bodies obtain, manage, and regulate this crucial fuel is essential for maintaining optimal health and preventing a wide range of metabolic disorders.
The Body's Glucose Orchestra: A High-Level View
The human body has evolved an incredibly sophisticated system for extracting glucose from food, distributing it to cells, and maintaining a stable blood sugar level. This system involves a carefully orchestrated interplay of organs, hormones, and intricate biochemical pathways.
This intricate regulatory mechanism involves a complex network of interconnected processes.
Here’s a simplified overview of the key players:
-
Small Intestine: This is the primary site for glucose absorption from digested food. Specialized cells lining the intestinal wall actively transport glucose into the bloodstream.
-
Liver: Acting as the central glucose processing and storage hub, the liver either stores excess glucose as glycogen or releases glucose into the bloodstream as needed to maintain stable levels.
-
Pancreas: This crucial gland produces two key hormones – insulin and glucagon – that work in tandem to regulate blood sugar levels.
-
Insulin: Released when blood sugar rises, insulin acts as a key that unlocks cells, allowing glucose to enter and be used for energy or stored for later use.
-
Glucagon: Released when blood sugar falls too low, glucagon signals the liver to break down stored glycogen and release glucose into the bloodstream.
This delicate balance is vital for ensuring that cells receive a constant supply of energy while preventing the damaging effects of excessively high or low blood sugar levels.
Why Understanding Glucose Matters
A deeper understanding of this "symphony of sugar" is crucial for proactively managing our health. By understanding the processes of glucose absorption and regulation, individuals can make informed dietary choices and lifestyle modifications to support optimal metabolic function.
Poorly managed blood sugar can lead to a cascade of health problems, including type 2 diabetes, heart disease, nerve damage, and kidney disease.
Therefore, knowledge of glucose metabolism empowers individuals to take control of their health and proactively prevent or manage these conditions.

Understanding this fundamental process provides insights into how dietary choices impact overall health and well-being. By making informed choices about what we eat, we can better support our body's natural ability to regulate blood sugar, fostering a healthier and more energetic life.
The Digestive Cascade: Unlocking Glucose from Our Food
With a foundational understanding of glucose's central role established, we now turn our attention to the fascinating journey of how this crucial energy source is liberated from the foods we consume. The digestive system acts as a highly efficient disassembly line, meticulously breaking down complex carbohydrates into simpler sugars ready for absorption.
Carbohydrates: From Complex to Simple
Carbohydrates, a macronutrient class found abundantly in grains, fruits, vegetables, and legumes, are the primary dietary source of glucose. These molecules exist in various forms, ranging from the complex structures of starches and fibers to the simpler forms of disaccharides (like sucrose and lactose) and monosaccharides (like glucose and fructose).
The digestive process begins in the mouth, where salivary amylase initiates the breakdown of starches into smaller polysaccharides. This process continues in the small intestine, where pancreatic amylase further breaks down these polysaccharides into disaccharides. Enzymes located on the surface of the small intestinal cells, such as sucrase, lactase, and maltase, then cleave these disaccharides into their constituent monosaccharides, including, most importantly, glucose.
The Small Intestine: A Master of Absorption
The small intestine is specifically designed for optimal nutrient absorption, featuring a series of structural adaptations that dramatically increase its surface area. The inner lining of the small intestine is folded into circular folds called plicae circulares, which increase the surface area.
Even further, the surface of these folds are covered in tiny finger-like projections called villi. Each villus, in turn, is covered with even smaller microscopic projections called microvilli, forming a "brush border" that significantly expands the absorptive surface.
This massive surface area ensures that the small intestine can efficiently absorb the glucose and other nutrients released from digested food.
The Villus Structure and Nutrient Uptake
Within each villus is a network of capillaries and a lymphatic vessel called a lacteal. The capillaries absorb the glucose molecules into the bloodstream, where they can be transported to the liver and other tissues.
SGLT1: The Sodium-Glucose Link
The absorption of glucose across the intestinal lining is not a passive process. It relies on specialized transport proteins embedded in the cell membranes of the enterocytes, the epithelial cells lining the small intestine. One of the key players in this process is the Sodium-Glucose Co-Transporter 1 (SGLT1).
SGLT1 is a symporter, meaning it transports two substances across the cell membrane simultaneously. In this case, it transports one molecule of glucose along with two sodium ions from the intestinal lumen into the enterocyte. This process is driven by the electrochemical gradient of sodium, which is maintained by the sodium-potassium pump on the basolateral membrane of the enterocyte.
This co-transport mechanism allows the enterocyte to efficiently uptake glucose, even when the glucose concentration in the intestinal lumen is low. This active transport mechanism ensures that the body can effectively absorb glucose from the diet, regardless of the dietary intake.
Key Players: Enterocytes, GLUTs, and the Absorption Arena
Having navigated the broader landscape of digestion, it's time to zoom in on the microscopic machinery that makes it all possible. The absorption of glucose isn't a passive process; it's a carefully orchestrated cellular event, reliant on specialized cells, intricate structures, and dedicated transport proteins. Let's explore the vital roles of enterocytes, GLUTs, the brush border, and capillaries in this absorption arena.
Enterocytes: The Gatekeepers of Glucose Absorption
Enterocytes, the specialized epithelial cells lining the small intestine, are the primary workhorses of nutrient absorption. These cells possess a unique architecture optimized for capturing glucose and other simple sugars like fructose. Their apical membrane, facing the intestinal lumen, is densely packed with microvilli, forming the brush border. This brush border dramatically increases the surface area available for absorption, maximizing the opportunity for glucose to be captured.
Enterocytes don't discriminate; they absorb both glucose and fructose, albeit through different mechanisms. Glucose, as we've seen, primarily relies on the SGLT1 transporter for initial uptake, which is driven by a sodium gradient. Fructose, on the other hand, primarily utilizes the GLUT5 transporter.
Once inside the enterocyte, both sugars are then transported across the basolateral membrane (the side facing the bloodstream) via other GLUT transporters, allowing them to enter the capillaries and begin their journey to the liver.
GLUTs: The Glucose Transporter Family
The term "GLUT" refers to a family of facilitative glucose transporters, membrane proteins that enable glucose to cross cell membranes. They don’t require energy, instead relying on concentration gradients to shuttle glucose across the cell membrane. Different GLUT isoforms are expressed in various tissues throughout the body, each with distinct properties and roles.
Specific GLUT Transporters:
-
GLUT2: Present in the liver, pancreas, and small intestine, GLUT2 has a high capacity but low affinity for glucose. It plays a role in transporting glucose out of enterocytes into the bloodstream and in glucose sensing by pancreatic beta cells.
-
GLUT4: Found primarily in muscle and fat tissue, GLUT4 is insulin-dependent. Insulin stimulates the translocation of GLUT4 to the cell surface, enabling these tissues to take up glucose from the blood. This is the primary mechanism by which insulin lowers blood glucose levels.
-
GLUT5: Specific for fructose, GLUT5 is primarily expressed in the small intestine. As mentioned earlier, GLUT5 facilitates the absorption of fructose from the intestinal lumen into enterocytes.
The interplay between these different GLUT transporters ensures efficient glucose uptake and distribution throughout the body.
The Brush Border: Maximizing Surface Area
The brush border, formed by the microvilli on the apical surface of enterocytes, is a critical structural feature that amplifies the absorptive capacity of the small intestine. These tiny, finger-like projections increase the surface area available for nutrient absorption by as much as 600-fold. This increased surface area allows for a significantly greater number of transporters to be present, facilitating the efficient uptake of glucose and other nutrients.
The brush border is not just a passive surface; it's also the site of action for several digestive enzymes that further break down carbohydrates into simple sugars, preparing them for absorption.
Capillaries: The Highway to the Liver
Once glucose is transported across the basolateral membrane of the enterocyte, it enters the capillaries located within the villi of the small intestine. These capillaries are part of the hepatic portal system, which carries blood directly from the small intestine to the liver.
This direct route to the liver is crucial because it allows the liver to act as the first line of defense in regulating blood glucose levels. The liver can then either store the glucose as glycogen, release it back into the bloodstream, or convert it into other molecules as needed. The capillaries act as the crucial link between the absorption process in the small intestine and the liver's regulatory functions.
The Liver's Vital Role: Storage, Release, and Metabolic Control
Having successfully navigated the digestive process and absorbed glucose from the small intestine, our body now relies on a critical intermediary: the liver. This often-underestimated organ plays a pivotal role in maintaining glucose homeostasis, acting as both a reservoir and a regulator. It's here that the absorbed glucose is either stored for future use or processed for immediate energy needs, ensuring a constant and reliable supply for our cells.
The Portal Vein: Glucose's Highway to the Liver
The journey of glucose from the small intestine to the liver is direct and efficient, thanks to the hepatic portal vein. This specialized vessel collects blood rich in nutrients absorbed from the digestive tract, including glucose, and transports it directly to the liver.
This direct route is crucial, allowing the liver to be the first organ to process and metabolize these nutrients. This "first-pass" metabolism enables the liver to rapidly respond to changes in blood glucose levels, preventing drastic spikes or drops and maintaining a stable internal environment.
Glycogenesis: Stockpiling Glucose for a Rainy Day
When glucose levels are high, such as after a carbohydrate-rich meal, the liver steps in to store the excess. It does this through a process called glycogenesis, where glucose molecules are linked together to form a larger molecule called glycogen.
Glycogen acts as a readily available storage form of glucose. Think of it as the body's glucose savings account, ready to be tapped into when energy demands increase or when blood sugar levels start to decline.
Glycogenolysis: Releasing the Stored Energy
Conversely, when blood glucose levels fall, the liver initiates glycogenolysis. This is the breakdown of glycogen back into individual glucose molecules, which are then released into the bloodstream.
This process ensures that cells continue to receive a constant supply of glucose, even when we are fasting, exercising, or experiencing stress. It's a critical mechanism for maintaining brain function, as the brain relies almost exclusively on glucose for energy.
Gluconeogenesis: Creating Glucose from Scratch
The liver's role extends beyond simply storing and releasing glucose. It also has the remarkable ability to synthesize glucose from non-carbohydrate sources, such as amino acids, lactate, and glycerol, through a process called gluconeogenesis.
This is especially important during prolonged fasting or starvation, when glycogen stores are depleted. Gluconeogenesis ensures that the body can continue to produce glucose, even when dietary sources are scarce. This process is energetically expensive, highlighting its importance in survival.
The Liver: A Master Metabolic Controller
In conclusion, the liver is far more than just a storage depot for glucose. It is a dynamic and sophisticated metabolic hub, constantly monitoring blood glucose levels and adjusting its activities to maintain a delicate balance.
Through glycogenesis, glycogenolysis, and gluconeogenesis, the liver ensures that our cells receive a steady and reliable supply of glucose, supporting energy production and overall health. Understanding the liver's pivotal role in glucose metabolism is essential for appreciating the complexity and efficiency of the human body.
Hormonal Harmony: Insulin and Glucagon's Balancing Act
Having successfully navigated the digestive process and absorbed glucose from the small intestine, our body now relies on a critical intermediary: the liver. This often-underestimated organ plays a pivotal role in maintaining glucose homeostasis, acting as both a reservoir and a regulator. However, the liver doesn't operate in isolation. Its actions are precisely orchestrated by two key hormonal players: insulin and glucagon. These hormones, secreted by the pancreas, engage in a delicate dance to ensure blood glucose levels remain within a narrow, optimal range. This section will explore the intricacies of this hormonal interplay and its crucial role in metabolic health.
The Pancreas: Endocrine Maestro
The pancreas, a gland located behind the stomach, has both digestive and hormonal functions. Its hormonal role is fulfilled by specialized clusters of cells called the islets of Langerhans. Within these islets reside alpha cells, which produce glucagon, and beta cells, which synthesize insulin.
These hormones are released directly into the bloodstream, allowing them to rapidly reach their target organs and exert their effects. This precise release mechanism is paramount for maintaining the necessary blood sugar levels.
Insulin: The Key to Cellular Uptake
Insulin's primary function is to lower blood glucose levels. It achieves this by acting as a "key" that unlocks the doors of cells, allowing glucose to enter and be used for energy or stored for later use. Specifically, insulin binds to receptors on cell membranes, triggering a cascade of events that ultimately leads to the translocation of GLUT4 transporters to the cell surface.
GLUT4 transporters act as channels that facilitate the movement of glucose from the bloodstream into the cell's interior.
This process is particularly important in muscle cells, where glucose is used for energy during physical activity, and in adipose tissue (fat cells), where glucose is converted into triglycerides for long-term energy storage. The liver also responds to insulin by taking up glucose and storing it as glycogen, a process known as glycogenesis.
Essentially, insulin promotes the storage of glucose and its utilization by cells, reducing its concentration in the bloodstream.
Glucagon: The Glucose Mobilizer
In contrast to insulin, glucagon works to increase blood glucose levels. It is released when blood sugar levels fall too low, signaling the liver to release stored glucose back into the bloodstream.
Glucagon primarily targets the liver, stimulating two key processes: glycogenolysis and gluconeogenesis. Glycogenolysis is the breakdown of glycogen into glucose, effectively releasing stored glucose back into circulation. Gluconeogenesis is the synthesis of glucose from non-carbohydrate sources, such as amino acids and glycerol, providing an additional source of glucose when glycogen stores are depleted.
By stimulating these processes, glucagon ensures that the body has a readily available supply of glucose, even when dietary intake is insufficient or when energy demands are high.
Feedback Loops: Maintaining the Balance
The interplay between insulin and glucagon is governed by intricate feedback loops that ensure blood glucose levels remain within a tight, homeostatic range.
When blood glucose levels rise, such as after a meal, the pancreas releases insulin. Insulin then facilitates glucose uptake by cells and promotes glycogen storage in the liver, effectively lowering blood glucose levels. As blood glucose levels fall, insulin secretion decreases.
Conversely, when blood glucose levels fall, the pancreas releases glucagon. Glucagon stimulates the liver to release glucose, raising blood glucose levels. As blood glucose levels rise, glucagon secretion decreases.
This elegant push-and-pull dynamic between insulin and glucagon creates a self-regulating system that prevents blood glucose levels from fluctuating too wildly. Disruptions to this system, such as in diabetes, can have profound consequences for health.
Therefore, understanding the mechanisms governing insulin and glucagon secretion and action is fundamental to appreciating the complexities of metabolic regulation and the importance of maintaining blood sugar control.
Dietary Dynamics: Fueling the System Wisely
Having navigated the intricate dance of hormonal regulation by insulin and glucagon, we now turn our attention to the source of it all: our diet. The food we consume is not just fuel; it's a complex symphony of sugars, starches, and fibers that profoundly influences our blood sugar levels and, consequently, our overall health. Understanding how different dietary components interact with our glucose metabolism is crucial for maintaining stable energy levels and preventing metabolic disorders.
The Sugar Spectrum: From Simple to Complex
Different food sources containing sugars have distinctly different effects on blood sugar. Simple sugars, like those found in processed foods, syrups, and fruit juices, are rapidly absorbed, leading to a swift spike in blood glucose.
This rapid influx of glucose triggers a large insulin release, which, while initially effective in lowering blood sugar, can eventually lead to insulin resistance and other metabolic complications with chronic overconsumption.
Complex carbohydrates, found in whole grains, legumes, and starchy vegetables, are digested more slowly. This slower digestion provides a more gradual and sustained release of glucose into the bloodstream, reducing the burden on the pancreas and promoting better blood sugar control.
Glycemic Index and Glycemic Load: Decoding Food's Impact
To better understand the impact of different foods on blood sugar, the concepts of Glycemic Index (GI) and Glycemic Load (GL) were developed.
The Glycemic Index (GI) ranks carbohydrates on a scale from 0 to 100 based on how quickly they raise blood sugar levels after consumption, compared to a reference food like pure glucose or white bread. Foods with a high GI (70 or more) cause a rapid and significant rise in blood sugar, while those with a low GI (55 or less) have a more gradual effect.
However, GI alone doesn't tell the whole story. The Glycemic Load (GL) takes into account both the GI of a food and the amount of carbohydrate per serving. It provides a more accurate measure of a food's overall impact on blood sugar.
A food with a high GI but a low carbohydrate content might have a moderate GL, indicating a less dramatic effect on blood sugar than its GI alone would suggest. GL is calculated as (GI x grams of carbohydrate per serving) / 100. A GL of 20 or more is considered high, 11-19 is medium, and 10 or less is low.
The Fiber Factor: Slowing Down the Sugar Rush
Dietary fiber plays a crucial role in modulating glucose absorption rates. Fiber, particularly soluble fiber, forms a gel-like substance in the digestive tract, slowing down the absorption of glucose and preventing rapid spikes in blood sugar.
Foods rich in fiber, such as whole grains, fruits, vegetables, and legumes, not only have a lower GI and GL but also contribute to a feeling of fullness, which can aid in weight management and further improve blood sugar control.
By choosing fiber-rich foods over refined and processed options, we can promote a more stable and sustained release of glucose, reducing the risk of metabolic complications and supporting overall health.
Incorporating fiber into meals can significantly blunt the postprandial glucose response. This is especially relevant when consuming foods that might otherwise cause a rapid rise in blood sugar.
Ultimately, understanding how different food sources of sugar, coupled with the concepts of GI, GL, and the crucial role of dietary fiber, empowers us to make informed choices that fuel our bodies wisely, promote stable blood sugar levels, and support long-term health.
Video: Sugar's Journey: Where Does Sugar Enter Blood?
FAQs: Sugar's Journey
How does sugar from food get into my bloodstream?
After you eat, your digestive system breaks down food, including carbohydrates, into glucose (sugar). This glucose is absorbed primarily in the small intestine. It’s here where does sugar enter the blood, specifically through the intestinal walls.
What happens after sugar enters my bloodstream?
Once glucose is absorbed into the blood, the pancreas releases insulin. Insulin acts like a key, allowing glucose to enter cells throughout the body to be used for energy. Excess glucose can also be stored in the liver and muscles.
Does sugar from all foods enter the blood at the same rate?
No. Foods with a high glycemic index (GI) are digested quickly, causing a rapid spike in blood sugar levels. Foods with a low GI are digested more slowly, leading to a more gradual rise in blood sugar. The small intestine is still the place where does sugar enter the blood, but the rate varies.
What happens if I have too much sugar in my blood?
Chronically high blood sugar levels can lead to several health problems, including type 2 diabetes, heart disease, and nerve damage. Managing diet and exercise is important for maintaining healthy blood sugar levels after the small intestine is where does sugar enter the blood.
So, next time you're enjoying that sweet treat, remember the incredible journey sugar takes to fuel your body! From your mouth to your small intestine, that's where does sugar enter the blood, ready to provide you with energy. Pretty amazing, right?