Melting Point of Stearic Acid: Key Properties
Stearic acid, a saturated fatty acid commonly utilized in the production of candles and cosmetics, exhibits a key physical property: the melting point of stearic acid. The melting point of stearic acid, typically around 69.3°C (156.7°F), is an important parameter analyzed using Differential Scanning Calorimetry (DSC) in quality control processes. Cargill, a major global supplier of stearic acid, provides detailed specifications that include precise melting point ranges to ensure product consistency. Variations in the melting point of stearic acid can indicate the presence of impurities or different polymorphic forms, factors extensively studied by organizations such as the American Oil Chemists' Society (AOCS).
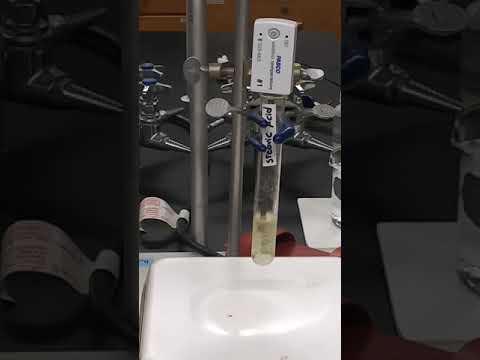
Image taken from the YouTube channel CHEM ZJ , from the video titled Freezing Point of Stearic Acid Part III .
Stearic acid, systematically known as octadecanoic acid, is a prevalent saturated fatty acid found abundantly in both animal and vegetable fats. Its chemical formula, CH3(CH2)16COOH, reveals a straight 18-carbon chain with a carboxyl group at one end, a structure that dictates its unique physical and chemical properties.
Beyond its chemical structure, stearic acid serves as a crucial building block in numerous industrial processes. It's a key ingredient in the manufacturing of:
- Candles.
- Cosmetics.
- Plastics.
- Pharmaceuticals.
Its versatility stems from its stability, lubricating properties, and ability to modify the texture and consistency of various products.
Understanding Stearic Acid's Melting Point: Why It Matters
Among its various characteristics, the melting point of stearic acid holds particular significance. The melting point is the specific temperature at which a substance transitions from a solid to a liquid state.
For stearic acid, this temperature window is critical for determining its behavior during processing, storage, and application. Understanding the factors that influence its melting point is paramount for achieving desired product characteristics and ensuring consistent performance.
Scope of Exploration: A Journey into Phase Transition
This discussion will delve into the intricacies of stearic acid's melting point, exploring the fundamental concepts and factors at play.
We will examine:
- The role of intermolecular forces.
- The influence of crystalline structure.
- The impact of impurities on its thermal behavior.
By understanding these factors, we aim to provide a comprehensive overview of the melting point of stearic acid and its implications across various industries.
Stearic Acid: A Deep Dive into Fundamental Properties
Stearic acid, systematically known as octadecanoic acid, is a prevalent saturated fatty acid found abundantly in both animal and vegetable fats. Its chemical formula, CH3(CH2)16COOH, reveals a straight 18-carbon chain with a carboxyl group at one end, a structure that dictates its unique physical and chemical properties.
Beyond its chemical structure, a deeper understanding of stearic acid’s properties is essential for appreciating its diverse applications. We will explore the chemical formula and its implications, the molecular weight and its effects, and the classification of stearic acid within lipid chemistry.
The Significance of the Chemical Formula (C18H36O2)
The chemical formula of stearic acid, C18H36O2, is more than just a symbolic representation. It encapsulates the very essence of its behavior and characteristics.
Each element and its quantity within the formula plays a critical role. The 18 carbon atoms (C18) form the backbone of the molecule, creating a long, nonpolar chain. This chain is primarily responsible for stearic acid's hydrophobic nature and its tendency to interact with other nonpolar substances.
The 36 hydrogen atoms (H36) saturate the carbon chain, meaning each carbon atom is bonded to the maximum number of hydrogen atoms possible. This saturation is a key feature of saturated fatty acids and contributes to the molecule's stability and relatively high melting point.
Finally, the two oxygen atoms (O2), along with one of the carbon atoms, form the carboxyl group (COOH). This functional group imparts a slightly polar character to one end of the molecule, allowing it to participate in hydrogen bonding and other polar interactions, albeit to a limited extent.
Molecular Weight: Impact on Physical Characteristics
The molecular weight of stearic acid is approximately 284.48 g/mol. This value represents the mass of one mole of stearic acid molecules and is calculated by summing the atomic weights of all the atoms in the chemical formula.
The molecular weight has a direct impact on several physical characteristics:
-
Melting Point: Higher molecular weight typically correlates with a higher melting point due to increased intermolecular forces.
-
Boiling Point: Similarly, a higher molecular weight leads to a higher boiling point.
-
Viscosity: Stearic acid, with its relatively high molecular weight, exhibits a certain degree of viscosity in its liquid state.
-
Solubility: The molecular weight, combined with the nonpolar nature of the long carbon chain, influences the solubility of stearic acid in various solvents.
Stearic Acid as a Fatty Acid: Classification Within Lipid Chemistry
In the realm of lipid chemistry, stearic acid is classified as a fatty acid, specifically a saturated fatty acid. Fatty acids are carboxylic acids with long aliphatic tails, which can be either saturated or unsaturated.
Saturated vs. Unsaturated Fatty Acids
The distinction between saturated and unsaturated fatty acids lies in the presence or absence of double bonds between carbon atoms in the hydrocarbon chain. Saturated fatty acids, like stearic acid, have no double bonds, resulting in a straight, linear chain.
Unsaturated fatty acids, on the other hand, contain one or more double bonds, introducing kinks or bends in the chain. This difference in structure has significant consequences:
-
Packing Efficiency: Saturated fatty acids pack more tightly together than unsaturated fatty acids, leading to stronger intermolecular forces and higher melting points.
-
Physical State: At room temperature, saturated fatty acids are typically solid, while unsaturated fatty acids are often liquid.
Long-Chain Structure and Melting Point
The long-chain structure of stearic acid plays a crucial role in determining its melting point. The longer the carbon chain, the greater the surface area available for intermolecular interactions. These interactions primarily involve Van der Waals forces, specifically London Dispersion Forces (LDFs).
LDFs are temporary, induced dipoles that arise from fluctuations in electron distribution within the molecules. The strength of LDFs increases with the size and surface area of the molecule. Therefore, the long carbon chain of stearic acid allows for significant LDF interactions, resulting in a relatively high melting point compared to fatty acids with shorter chains. The forces between each molecule need more energy to break, hence the solid state at room temperature.
The Melting Point Concept: A Fundamental Phase Transition
Understanding the behavior of stearic acid necessitates a firm grasp of phase transitions, particularly the melting point. The melting point isn't merely a temperature; it's a critical property reflecting the underlying forces governing a substance's state of matter. Let's delve into the concept of melting point and its profound implications.
Defining the Melting Point
The melting point is precisely defined as the temperature at which a substance transitions from a solid state to a liquid state at a given pressure (typically atmospheric pressure). At this specific temperature, the solid and liquid phases exist in equilibrium. This equilibrium signifies a dynamic process where molecules are simultaneously transitioning between the solid and liquid states at equal rates.
The Solid-to-Liquid Phase Transition: A Molecular Perspective
The melting process is an endothermic phase transition, meaning it requires energy input, usually in the form of heat.
In the solid-state, molecules are held together by strong intermolecular forces, arranging themselves into a highly ordered crystalline structure.
As heat is applied, the molecules gain kinetic energy, vibrating more vigorously within their lattice positions.
At the melting point, the molecules possess enough energy to overcome these intermolecular forces. This allows them to break free from the ordered structure and move more freely, resulting in the liquid state.
The temperature remains constant during the phase change, as the added energy is used to break intermolecular bonds rather than increase the kinetic energy (temperature) of the molecules.
Only after all the solid has transitioned to a liquid will the temperature begin to rise again with further heating.
Importance of Melting Point: Substance Identification and Quality Control
The melting point serves as a crucial characteristic property for substance identification and quality control.
Each pure crystalline substance has a unique melting point, making it a valuable "fingerprint."
By comparing the experimentally determined melting point of a substance to known values, one can verify its identity.
Moreover, the sharpness of the melting point range provides insight into the purity of a substance.
A pure substance will exhibit a sharp, well-defined melting point, typically within a narrow temperature range (e.g., 1-2 °C).
The presence of impurities disrupts the crystal lattice, leading to a melting point depression and a broader melting point range. This makes melting point determination a routine method for assessing the purity of chemical compounds in various industries, from pharmaceuticals to materials science.
Decoding the Melting Point of Stearic Acid: Influential Factors
Understanding the behavior of stearic acid necessitates a firm grasp of phase transitions, particularly the melting point. The melting point isn't merely a temperature; it's a critical property reflecting the underlying forces governing a substance's state of matter. Let's delve into the complex interplay of factors that dictate stearic acid's melting point.
Intermolecular Forces: The Dominant Influence
The melting point of stearic acid is primarily governed by the strength of its intermolecular forces. These forces, although weaker than intramolecular bonds, dictate how readily molecules transition from a solid, ordered state to a liquid, more disordered state. The stronger the intermolecular forces, the more energy (heat) required to overcome them, and thus, the higher the melting point.
Van der Waals Forces and Molecular Attraction
Van der Waals forces play a crucial role in the attraction between stearic acid molecules. These forces encompass dipole-dipole interactions, dipole-induced dipole interactions, and, most significantly, London Dispersion Forces (LDFs).
London Dispersion Forces: The Key Contributor
In the case of stearic acid, London Dispersion Forces (LDFs) are the predominant type of intermolecular force. These forces arise from temporary fluctuations in electron distribution, creating instantaneous dipoles that induce dipoles in neighboring molecules.
The strength of LDFs is directly proportional to the surface area of the molecule and its polarizability.
Stearic acid, with its long hydrocarbon chain, possesses a large surface area, leading to substantial LDF interactions. The longer the carbon chain, the greater the surface area and the stronger the LDFs, resulting in a higher melting point. This explains why stearic acid, with its 18-carbon chain, has a relatively high melting point compared to shorter-chain fatty acids.
Crystalline Structure and Packing Efficiency
Stearic acid molecules arrange themselves in a crystalline structure in the solid state. The specific arrangement of these molecules significantly impacts the melting point. The more efficiently the molecules pack together, the stronger the intermolecular forces and the higher the melting point.
A tightly packed crystal lattice requires more energy to disrupt, meaning the molecules need to overcome a greater energetic barrier to transition into the liquid phase. Lattice energy, which is the energy required to separate one mole of a solid ionic compound into gaseous ions, is therefore indirectly proportional to the melting point in the context of stearic acid crystal structures.
Impact of Impurities: Melting Point Depression
The presence of impurities invariably affects the melting point of stearic acid. Impurities disrupt the regularity of the crystal lattice. This disruption makes it easier to break the intermolecular forces holding the solid together, leading to a lower melting point than that of pure stearic acid.
This phenomenon is known as melting point depression. The extent of the depression depends on the nature and concentration of the impurities. Even small amounts of impurities can noticeably lower the melting point, making melting point determination a useful indicator of sample purity.
Polymorphism: Multiple Crystalline Forms
Stearic acid exhibits polymorphism, meaning it can exist in multiple crystalline forms, each with a distinct arrangement of molecules. These different crystalline structures, or polymorphs, arise due to variations in the packing arrangement of the fatty acid chains.
Each polymorph possesses a unique set of physical properties, including a different melting point. The stability of each polymorph depends on factors such as temperature, pressure, and solvent. Recognizing and controlling polymorphism is crucial in applications where specific melting behavior is desired, such as in pharmaceutical formulations.
Thermodynamics of Melting: Energy and Stability
Understanding the behavior of stearic acid necessitates a firm grasp of phase transitions, particularly the melting point. The melting point isn't merely a temperature; it's a critical property reflecting the underlying forces governing a substance's state of matter. Let's delve into the thermodynamic principles that govern the melting process of stearic acid and the energy considerations involved.
Basic Thermodynamic Principles in Phase Transitions
Phase transitions, such as melting, are governed by fundamental thermodynamic principles. These principles dictate the spontaneity and equilibrium of these processes. Thermodynamics provides the framework for understanding energy changes and molecular behavior during phase transitions.
Gibbs Free Energy (G) plays a central role. It determines whether a process will occur spontaneously at a given temperature and pressure. Melting occurs when the Gibbs Free Energy of the liquid phase becomes lower than that of the solid phase.
The relationship G = H - TS (where H is enthalpy, T is temperature, and S is entropy) highlights the interplay between energy (enthalpy) and disorder (entropy).
Enthalpy of Fusion: Breaking Intermolecular Bonds
The Enthalpy of Fusion (ΔHfus) is the amount of heat energy required to transform a substance from a solid to a liquid at its melting point. This energy is specifically used to overcome the intermolecular forces that hold the molecules in a fixed, crystalline structure in the solid phase. For stearic acid, a significant portion of ΔHfus is used to disrupt the Van der Waals forces, especially London Dispersion Forces, between the long hydrocarbon chains.
A higher Enthalpy of Fusion indicates stronger intermolecular forces that need to be overcome during melting. The ΔHfus value is a characteristic property, reflecting the strength of the solid-state packing. Its melting point provides insight into the overall stability of the stearic acid crystal lattice.
Thermal Stability and Degradation Pathways
Thermal stability refers to a substance's ability to resist decomposition or chemical change when exposed to heat. Stearic acid exhibits relatively good thermal stability under normal conditions. However, at elevated temperatures, it can undergo degradation through various pathways.
Potential Degradation Mechanisms
Oxidation can occur in the presence of oxygen. This can lead to the formation of volatile organic compounds (VOCs) and other byproducts. Cracking or thermal decomposition can also happen. This results in smaller hydrocarbon fragments and carbon dioxide.
The specific degradation products and rate depend on factors. These factors include: temperature, atmosphere, and the presence of catalysts. Understanding these pathways is crucial in applications involving high temperatures. Also crucial in applications involving prolonged exposure to heat.
Heat Capacity of Stearic Acid
Heat capacity quantifies the amount of heat required to raise the temperature of a substance by a specific amount (usually 1 degree Celsius or Kelvin). Stearic acid possesses a characteristic heat capacity that varies with temperature and its physical state (solid or liquid).
Knowing the heat capacity is essential in applications where temperature control is important. This includes processes like:
- Molding
- Extrusion
- Formulation of cosmetic or pharmaceutical products.
A higher heat capacity means more energy is needed to change the temperature of the stearic acid. This can impact energy consumption and processing times in various applications.
Experimental Determination: Measuring the Melting Point of Stearic Acid
Understanding the behavior of stearic acid necessitates a firm grasp of phase transitions, particularly the melting point. The melting point isn't merely a temperature; it's a critical property reflecting the underlying forces governing a substance's state of matter. Let's delve into the experimental methodologies used to determine this crucial characteristic, focusing on both traditional and advanced techniques.
Traditional Melting Point Determination: A Visual Approach
The classical method for determining the melting point of stearic acid relies on visual observation using a melting point apparatus. This typically involves placing a small amount of the substance, usually in powdered form, into a thin-walled capillary tube.
This tube is then placed inside a heating block or a liquid bath, allowing for gradual and controlled heating.
Careful observation is crucial during this process.
The temperature at which the first drop of liquid is observed and the temperature at which the entire sample becomes liquid are recorded as the melting range. This range provides an indication of the purity of the sample.
A sharp, narrow melting range generally suggests a purer substance, while a broader range indicates the presence of impurities.
The Crucial Role of Accurate Temperature Measurement
The accuracy of melting point determination hinges on the precision of temperature measurement. Traditional methods often employ mercury thermometers, while more modern setups utilize calibrated electronic temperature sensors.
Calibration is paramount.
Thermometers must be accurately calibrated against known standards to ensure reliable readings.
Similarly, electronic sensors require regular calibration to minimize systematic errors. The thermometer or sensor should be positioned in close proximity to the sample to accurately reflect its temperature during the melting process.
Advanced Techniques: Unveiling Thermal Behavior with Precision
While traditional methods offer a simple and direct approach, advanced techniques provide more detailed and precise measurements of the melting point and other thermal properties of stearic acid.
Differential Scanning Calorimetry (DSC): A Powerful Analytical Tool
Differential Scanning Calorimetry (DSC) stands out as a particularly powerful tool. DSC measures the heat flow associated with phase transitions as a function of temperature.
In a DSC experiment, the sample and a reference material are heated or cooled at a controlled rate. The instrument measures the difference in heat flow required to maintain both the sample and the reference at the same temperature.
During the melting process, the sample absorbs heat, resulting in a measurable endothermic peak on the DSC curve.
The onset temperature of this peak is typically taken as the melting point. DSC offers several advantages over traditional methods.
It provides a more accurate and objective determination of the melting point, eliminating the subjectivity associated with visual observation. DSC can also provide information about the enthalpy of fusion, which is the amount of heat required to melt the substance.
This information can be valuable for understanding the thermodynamic properties of stearic acid and its behavior in various applications.
Furthermore, DSC can detect polymorphic transitions and other thermal events that may not be readily apparent using traditional methods. This makes it a valuable tool for characterizing the thermal behavior of complex materials.
Video: Melting Point of Stearic Acid: Key Properties
FAQs: Melting Point of Stearic Acid: Key Properties
What factors influence the reported melting point of stearic acid?
The reported melting point of stearic acid can vary slightly depending on the purity of the sample and the specific measurement method used. Polymorphism, the existence of multiple crystal structures, can also affect the observed melting point.
Why is knowing the melting point of stearic acid important?
Knowing the melting point of stearic acid is crucial for quality control in various industries. It helps confirm the identity and purity of the compound, ensuring it meets specifications for use in soaps, cosmetics, plastics, and pharmaceuticals.
How does the melting point of stearic acid compare to other fatty acids?
Stearic acid has a relatively high melting point compared to shorter-chain saturated fatty acids. This is due to its longer carbon chain, which increases intermolecular forces. The melting point of stearic acid is typically lower than longer-chain saturated fatty acids.
Does the melting point of stearic acid change with pressure?
Yes, like most substances, the melting point of stearic acid is affected by pressure. Increased pressure generally leads to a slightly higher melting point of stearic acid. This effect is usually only significant at very high pressures.
So, there you have it! Hopefully, this gives you a clearer picture of stearic acid and just how important its melting point is. Remember, the melting point of stearic acid generally sits around 69-70°C (156-158°F), but keep an eye on those specific formulations! Now you know what to look for, you're well-equipped to understand this common fatty acid a little better.