Attenuation Coefficient: Soundproof Your Home Now!
Acoustic comfort in residential spaces is increasingly valued, leading homeowners to seek effective soundproofing solutions. The attenuation coefficient, a crucial property of materials, dictates their sound-absorbing capabilities and is vital for creating quieter living environments. Understanding this coefficient helps homeowners select the right materials, like dense fiberglass insulation from Owens Corning, a leading manufacturer of building materials, for optimal sound dampening. Professionals at the Acoustical Society of America (ASA) study and publish data on the acoustic properties of various materials, providing a valuable resource for understanding and comparing attenuation coefficients. Sophisticated tools such as the Bruel & Kjaer Type 2250 sound level meter are utilized to measure sound levels accurately and determine the effectiveness of different soundproofing strategies, ultimately quantifying the impact of a material's attenuation coefficient on reducing noise.
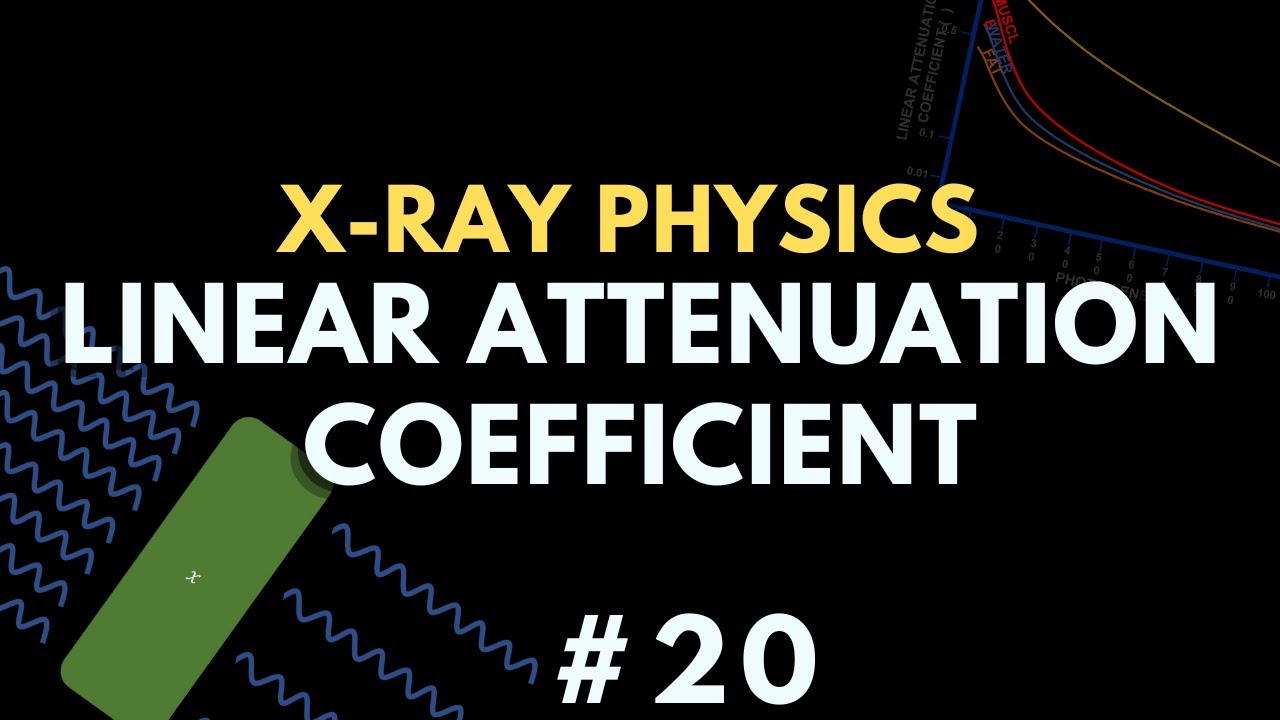
Image taken from the YouTube channel Radiology Tutorials , from the video titled Linear Attenuation Coefficient (LAC) and Mass Attenuation Coefficient | Radiology Physics Course #27 .
In our increasingly noisy world, the concept of sound attenuation has moved from a niche concern of acoustic engineers to a vital consideration for architects, designers, and homeowners alike. But what exactly is sound attenuation, and why is it so important?
Defining Sound Attenuation and Its Significance
At its core, sound attenuation refers to the reduction of sound energy as it travels through a medium or space. This reduction can occur through various mechanisms, including absorption, reflection, and diffusion. Effectively, attenuation is about making sound quieter, less intrusive, and more manageable.
The significance of sound attenuation stems from its impact on human comfort, productivity, and well-being. Excessive noise exposure can lead to a range of negative consequences, from sleep disturbances and stress to impaired cognitive function and even hearing loss. By implementing sound attenuation strategies, we can create environments that are more conducive to focus, relaxation, and overall health.
The Relevance of Sound Attenuation Across Diverse Environments
The need for sound attenuation is not confined to any single type of environment. Rather, it is a universal concern that spans residential, commercial, and industrial settings.
-
Residential Spaces: In homes, sound attenuation can improve sleep quality, reduce distractions, and create a more peaceful living environment. This is especially crucial in multi-family dwellings where noise from neighbors can be a significant issue.
-
Commercial Spaces: In offices, sound attenuation can enhance productivity, reduce stress, and improve communication. Open-plan offices, in particular, often require careful acoustic design to minimize distractions and maintain privacy.
-
Industrial Settings: In factories and other industrial environments, sound attenuation is essential for protecting workers from noise-induced hearing loss and other health problems. It can also improve communication and safety by reducing background noise levels.
Factors Influencing Sound Attenuation
Several factors influence the effectiveness of sound attenuation measures. These include the properties of the materials used, the design of the space, and the frequency of the sound.
-
Materials: Different materials have different sound absorption and transmission loss characteristics. Soft, porous materials like acoustic foam tend to be good at absorbing sound, while dense, massive materials like concrete are effective at blocking sound transmission.
-
Design: The design of a space can also have a significant impact on sound attenuation. Factors such as room shape, ceiling height, and the placement of sound-absorbing materials can all affect the way sound behaves in a room.
-
Frequency: Sound attenuation is often frequency-dependent. Some materials and designs are more effective at attenuating high-frequency sounds, while others are better at attenuating low-frequency sounds. Understanding the frequency content of the noise you are trying to reduce is crucial for selecting the right attenuation strategies.
Understanding the Building Blocks: Fundamental Properties of Sound
In our quest for effective sound attenuation, it's essential to first understand the fundamental properties that govern sound behavior. Sound isn't just a monolithic entity; it's a complex phenomenon characterized by frequency, amplitude, wavelength, and impedance. Mastering these concepts is the first, critical step in crafting effective sound control strategies.
Frequency: The Highs and Lows of Sound
Frequency, measured in Hertz (Hz), dictates the pitch of a sound. A higher frequency corresponds to a higher pitch, while a lower frequency equates to a lower pitch. This distinction is crucial in sound attenuation, as different materials are more effective at blocking different frequencies.
For example, dense, massive materials are generally better at blocking low-frequency sounds (like bass), while porous materials are more effective at absorbing high-frequency sounds (like speech). Understanding the frequency content of the noise you're trying to mitigate is therefore paramount in selecting the correct attenuating material.
Amplitude: The Loudness Factor
Amplitude refers to the intensity or loudness of a sound wave. It's directly related to the amount of energy the wave carries. Amplitude is measured in decibels (dB), a logarithmic scale where even small increases in dB represent significant increases in sound intensity.
Controlling amplitude is obviously a key aspect of sound attenuation. Reducing the amplitude of a sound wave diminishes its perceived loudness, making the environment more comfortable. Strategies to reduce amplitude often involve barriers, absorbers, and vibration damping techniques.
Wavelength: Fitting the Pieces Together
Wavelength is the distance between two consecutive crests or troughs of a sound wave. It's inversely proportional to frequency: lower frequencies have longer wavelengths, and higher frequencies have shorter wavelengths.
Wavelength plays a significant role in how sound interacts with materials. For example, a sound wave with a long wavelength may easily diffract around a small barrier. The size and shape of acoustic treatments, such as diffusers and absorbers, must be carefully considered in relation to the wavelengths they are intended to manage.
Impedance: The Resistance to Sound
Acoustic impedance is a measure of a material's resistance to the passage of sound waves. It's determined by the material's density and the speed of sound within it.
Large impedance mismatches between two materials cause sound waves to be reflected rather than transmitted. This principle is utilized in layered soundproofing systems, where alternating materials with differing impedance levels can dramatically reduce sound transmission. Understanding impedance is critical in designing effective barriers and decoupling structures.
By understanding these four fundamental properties of sound – frequency, amplitude, wavelength, and impedance – we lay the groundwork for developing and implementing effective sound attenuation solutions.
The Science of Silence: Mechanisms of Sound Reduction
The pursuit of effective sound attenuation hinges on understanding the underlying mechanisms that govern sound reduction. These mechanisms primarily fall into two categories: sound absorption and sound transmission loss (STL). Each plays a distinct role in mitigating unwanted noise, and a comprehensive approach to acoustic design necessitates a nuanced understanding of both.
Sound Absorption: Dissipating Sound Energy
Sound absorption is the process by which a material converts sound energy into other forms of energy, typically heat. This conversion reduces the amount of sound energy reflected back into a space, thereby decreasing reverberation and overall noise levels.
The effectiveness of a sound-absorbing material is determined by its absorption coefficient, a value between 0 and 1 that represents the proportion of sound energy absorbed by the material. A coefficient of 1 indicates perfect absorption, while 0 indicates complete reflection.
Porosity and Structure: Key to Absorption
The porosity and structure of a material are crucial factors in its ability to absorb sound. Porous materials, such as acoustic foam and mineral wool, contain interconnected air voids that allow sound waves to penetrate the material. As sound waves travel through these voids, friction between the air molecules and the material's fibers converts sound energy into heat.
The size, shape, and orientation of these pores significantly impact the material's absorption characteristics. Materials with a complex, tortuous pore structure tend to be more effective at dissipating sound energy across a wider range of frequencies.
Common Sound-Absorbing Materials
Several materials are widely used for their sound-absorbing properties.
Acoustic foam, with its open-celled structure, is a popular choice for recording studios and home theaters. It effectively absorbs mid- to high-frequency sounds, reducing echoes and reverberation.
Mineral wool and fiberglass insulation are commonly used in wall and ceiling cavities to absorb sound energy and improve sound isolation.
Acoustic panels, often constructed with a fiberglass or mineral wool core and wrapped in fabric, are another effective solution for reducing reverberation in offices, classrooms, and other large spaces.
Sound Transmission Loss (STL): Blocking Sound Propagation
Sound Transmission Loss (STL) refers to the reduction in sound energy as it passes through a barrier, such as a wall or floor. Unlike sound absorption, which dissipates sound energy, STL aims to block the transmission of sound from one space to another.
Defining and Measuring STL
STL is typically measured in decibels (dB) and represents the difference in sound pressure level between the source room and the receiving room.
A higher STL value indicates a greater reduction in sound transmission. The measurement of STL is conducted in controlled laboratory settings using specialized equipment, ensuring accurate and comparable data across various materials and constructions.
Mass, Material Properties, and the Mass Law
The mass of a barrier is a primary determinant of its STL. According to the mass law, the STL of a barrier increases with its mass per unit area. This principle highlights the effectiveness of dense materials like concrete and brick in blocking sound transmission.
However, material properties beyond mass also play a significant role. Stiffness, damping, and internal resonances can all influence the STL of a barrier.
Strategies for Maximizing STL
Several strategies can be employed to maximize STL in building design.
Layering materials of different densities and properties can create a more effective sound barrier than a single layer of the same total mass. This approach leverages the differing acoustic properties of each layer to attenuate sound waves across a broader frequency range.
The use of resilient channels in wall and ceiling construction can decouple the drywall from the studs, reducing the transmission of vibrations and improving STL. These channels effectively break the direct path for sound transmission, minimizing flanking noise.
Introducing air gaps in wall or floor assemblies can significantly improve STL. The air gap acts as a decoupling element, preventing sound waves from directly propagating through the structure.
The Arsenal of Acoustics: Materials and Products for Sound Attenuation
The pursuit of effective sound attenuation necessitates a deep understanding of the materials and products available. These tools, each with unique properties and applications, form the core of acoustic design. Let's explore the diverse "arsenal" at our disposal, examining their effectiveness, applications, and proper installation.
Acoustic Panels: Absorption and Aesthetics
Acoustic panels are engineered to absorb sound energy, reducing reflections and reverberation within a space. This leads to improved clarity and reduced noise levels.
Types and Applications
Fabric-wrapped panels are a popular choice for offices and studios, offering a wide range of colors and textures. Wood acoustic panels provide both sound absorption and an aesthetic appeal, often used in concert halls and auditoriums.
The application dictates panel placement. First reflection points (areas where sound first bounces off surfaces) are prime locations for panels.
Installation for Optimal Performance
Proper installation is critical. Panels are typically mounted on walls or ceilings using adhesives, impaling clips, or Z-clips. Spacing between panels can influence their effectiveness, especially at lower frequencies, so consult manufacturer guidelines.
Soundproof Curtains: A Layer of Defense
Soundproof curtains, also known as acoustic curtains, are designed to reduce sound transmission through windows and doors. While they don't completely block sound, they offer a noticeable improvement compared to standard curtains.
Design and Materials
These curtains are typically made from heavy, dense fabrics, often incorporating multiple layers and a blackout lining. The dense material helps to block sound waves, while the multiple layers provide additional insulation.
Effectiveness and Limitations
Soundproof curtains are most effective at blocking higher frequencies. Low-frequency sounds may still pass through. They’re a good option for reducing street noise or softening sounds from adjacent rooms.
Mass-Loaded Vinyl (MLV): Adding Weight to the Fight
Mass-loaded vinyl (MLV) is a dense, flexible material used to block sound transmission through walls, ceilings, and floors. Its high mass acts as a barrier, preventing sound waves from passing through.
Properties and Applications
MLV is commonly used in home theaters, recording studios, and industrial settings. It’s particularly effective at blocking mid- to low-frequency sounds.
Installation Techniques
MLV should be installed directly onto studs or joists before drywall or other finishing materials. Seams should be overlapped and sealed to prevent sound leaks.
Mineral Wool and Fiberglass Insulation: Filling the Gaps
Mineral wool and fiberglass insulation are widely used for their thermal and acoustic properties. They absorb sound energy within wall, ceiling, and floor cavities.
Properties and Advantages
Mineral wool, made from rock or slag, offers superior sound absorption compared to fiberglass. Fiberglass is a more economical option, but still provides noticeable sound reduction.
Installation Best Practices
Proper density and fill are crucial for optimal performance. Ensure the insulation fills the cavity completely without being overly compressed.
Acoustic Foam: Taming Reflections
Acoustic foam is designed to absorb sound reflections within a room, reducing reverberation and echo. It's commonly used in recording studios, home theaters, and vocal booths.
Types and Applications
Wedge and pyramid-shaped foam are popular choices for absorbing sound across a wide range of frequencies. Bass traps, thicker foam placed in corners, are specifically designed to absorb low-frequency sounds.
Placement Strategies
Placement is key for effectiveness. Focus on first reflection points and areas where sound tends to accumulate.
Soundproof Doors: Sealing the Entry
Soundproof doors are designed to minimize sound transmission between rooms or from the outside. They incorporate several features to achieve this.
Design Elements for Sound Reduction
Solid-core construction, tight seals, and perimeter gasketing are essential for soundproof doors. The solid core provides mass to block sound, while the seals prevent sound leaks around the edges.
Sealing and Gasketing
Proper sealing and gasketing are crucial. Use high-quality weather stripping around the door frame and a threshold seal at the bottom of the door.
Soundproof Windows: Blocking External Noise
Soundproof windows are designed to reduce noise intrusion from outside sources. They utilize specialized construction techniques to minimize sound transmission.
Construction Methods
Laminated glass, wider air gaps between panes, and multiple panes are common features of soundproof windows. Laminated glass has a layer of plastic between two panes of glass, which dampens sound vibrations.
Multi-Pane Configurations
Double- or triple-pane windows with varying glass thicknesses further enhance sound reduction. The different thicknesses help to disrupt sound waves at different frequencies.
Green Glue (Damping Compounds): Quieting Vibrations
Green Glue is a viscoelastic damping compound used to reduce sound transmission through walls, ceilings, and floors. It works by converting sound energy into heat, reducing vibrations.
Use of Viscoelastic Adhesives
Green Glue is applied between layers of building materials, such as drywall or plywood. When sound waves cause these layers to vibrate, the Green Glue absorbs the energy and dissipates it as heat.
Application Techniques
Apply Green Glue in a consistent, zigzag pattern across the entire surface of the first layer of material. Then, attach the second layer, ensuring good contact across the entire surface.
Real-World Acoustics: Practical Applications and Case Studies
The pursuit of effective sound attenuation necessitates a deep understanding of the materials and products available.
These tools, each with unique properties and applications, form the core of acoustic design.
Let's explore the diverse "arsenal" at our disposal, examining how these principles translate into tangible solutions across various environments.
From the meticulous acoustic sculpting of recording studios to the immersive soundscapes of home theaters and the sound isolation demands of apartment living, real-world scenarios showcase both the challenges and the triumphs of applied acoustics.
This section examines these practical applications through illustrative case studies.
Recording Studios: Crafting the Perfect Sonic Environment
Recording studios are perhaps the most demanding environments when it comes to acoustic control.
The goal is to capture pristine audio, free from unwanted reflections, resonances, or external noise.
Acoustic design in these spaces revolves around achieving a neutral sound field, allowing engineers to accurately record and mix music.
Key Acoustic Design Principles
Several key principles guide the design of recording studio acoustics:
-
Diffusion: Scattering sound waves to create a more uniform sound field and minimize strong reflections. Diffusers are strategically placed to break up sound waves and prevent flutter echoes.
-
Absorption: Absorbing sound energy to reduce reverberation time (RT60) and prevent unwanted coloration. Bass traps are crucial for controlling low-frequency build-up in corners.
-
Isolation: Preventing external noise from entering the studio and internal sound from leaking out.
This often involves multi-layered wall construction, floating floors, and airtight seals around doors and windows.
Material Selection and Placement
Material selection is paramount in achieving optimal studio acoustics.
Commonly used materials include:
- Acoustic Foam: Used to absorb mid and high frequencies, typically placed on walls and ceilings.
- Bass Traps: Designed to absorb low frequencies, often placed in corners where bass tends to accumulate.
- Diffusers: Available in various shapes and sizes, used to scatter sound waves and create a more even sound field.
- Acoustic Panels: Fabric-wrapped panels that provide both absorption and aesthetic appeal.
The strategic placement of these materials is crucial.
For example, early reflection points (the first points where sound waves reflect off surfaces and reach the listener) are prime locations for absorption.
Home Theaters: Optimizing Sound and Minimizing Noise Leakage
Home theaters present a unique set of acoustic challenges.
The objective is to create an immersive cinematic experience within the confines of a residential space, all while minimizing noise disturbance to other occupants or neighbors.
Optimizing Sound Quality
Achieving high-fidelity sound in a home theater involves careful consideration of several factors:
-
Speaker Placement: Proper speaker placement is essential for creating a realistic soundstage. THX guidelines provide recommendations for speaker angles and distances.
-
Room Dimensions: Room dimensions can significantly impact sound quality, particularly at low frequencies. Room modes (standing waves) can create peaks and nulls in the frequency response.
-
Acoustic Treatment: Acoustic treatment is crucial for controlling reflections and reverberation.
Absorption is particularly important in smaller rooms to prevent excessive echo.
Minimizing Noise Leakage
Preventing sound from escaping the home theater is equally important.
Common noise leakage points include:
- Walls: Walls can be soundproofed by adding mass, decoupling layers, and sealing air gaps. Mass-loaded vinyl (MLV) and resilient channels are often used.
- Doors: Solid-core doors with tight seals are essential for sound isolation.
- Windows: Double-paned windows with laminated glass can significantly reduce sound transmission.
Case Study Example: Residential Home Theater
A homeowner converted their basement into a dedicated home theater.
To optimize sound, they installed acoustic panels on the walls and ceiling to reduce reflections.
Bass traps were placed in the corners to control low-frequency buildup.
To minimize noise leakage, they added a layer of MLV to the walls and installed a solid-core door with weather stripping.
The result was a significantly improved viewing experience with minimal disturbance to the rest of the house.
Apartment Buildings: Navigating the Challenges of Shared Living Spaces
Apartment buildings present some of the most complex acoustic challenges.
The close proximity of multiple living units necessitates effective sound isolation to ensure privacy and minimize noise complaints.
Common Noise Issues
Several types of noise transmission are common in apartment buildings:
- Airborne Noise: Noise that travels through the air, such as voices, music, and television.
- Impact Noise: Noise that is generated by direct impact, such as footsteps, dropped objects, and slamming doors.
- Flanking Noise: Noise that travels indirectly through building structures, such as walls, floors, and ceilings.
Effective Soundproofing Solutions
Addressing these noise issues requires a multi-faceted approach:
- Floor/Ceiling Assemblies: Installing resilient flooring underlayments or suspended ceilings can significantly reduce impact noise.
- Wall Assemblies: Adding mass to walls (e.g., with MLV or additional layers of drywall) and decoupling them with resilient channels can improve airborne sound isolation.
- Sealing Air Gaps: Sealing air gaps around doors, windows, and pipes is crucial for preventing flanking noise.
- Acoustic Doors and Windows: Upgrading to acoustic doors and windows can provide significant improvements in sound isolation.
Case Study Example: Soundproofing Apartment Units
An apartment complex was experiencing numerous noise complaints from tenants.
To address the issue, the building management implemented a comprehensive soundproofing plan.
This included adding a layer of MLV to the walls between units, installing resilient flooring underlayments, and sealing all air gaps.
The result was a significant reduction in noise transmission, leading to improved tenant satisfaction.
By examining these real-world applications and case studies, we gain a deeper appreciation for the practical challenges and effective solutions in the field of sound attenuation.
The principles of acoustics, when applied thoughtfully and strategically, can transform our living and working environments, creating spaces that are both functional and enjoyable.
Quantifying Quiet: Measurement and Analysis of Sound
[Real-World Acoustics: Practical Applications and Case Studies The pursuit of effective sound attenuation necessitates a deep understanding of the materials and products available. These tools, each with unique properties and applications, form the core of acoustic design. Let's explore the diverse "arsenal" at our disposal, examining how...]
Before embarking on any serious sound attenuation endeavor, it's crucial to understand how sound is measured and quantified. Subjective impressions of loudness can be misleading; objective measurements provide a solid foundation for informed decision-making. This section will demystify the process of sound measurement, focusing on the decibel scale and the practical application of sound level meters.
The Decibel Scale: A Relative Measure of Sound
The decibel (dB) is the unit used to express the relative intensity of sound. It's important to grasp that the decibel scale is logarithmic, not linear. This means that an increase of 10 dB represents a tenfold increase in sound intensity.
For instance, a sound at 60 dB is ten times more intense than a sound at 50 dB, and one hundred times more intense than a sound at 40 dB.
This logarithmic scale is used because it aligns more closely with human perception of loudness. The human ear can perceive an incredibly wide range of sound intensities, and the decibel scale compresses this range into a more manageable and intuitive format.
Understanding Sound Pressure Level (SPL)
The decibel scale is most commonly used to measure Sound Pressure Level (SPL). SPL represents the pressure variations in the air caused by sound waves. A higher SPL indicates a louder sound.
It's crucial to specify the reference pressure level when discussing decibel measurements. Typically, SPL is referenced to 20 micropascals (µPa), which is considered the threshold of human hearing. Therefore, 0 dB SPL doesn't mean the absence of sound, but rather a sound pressure equal to the threshold of human hearing.
Common examples of dB SPL levels include:
- A whisper (around 30 dB SPL).
- Normal conversation (around 60 dB SPL).
- A busy street (around 80 dB SPL).
- A rock concert (around 110 dB SPL or higher).
Prolonged exposure to sounds above 85 dB SPL can cause hearing damage, highlighting the importance of understanding and managing noise levels.
Sound Level Meters: Tools for Objective Assessment
A sound level meter (SLM) is an instrument designed to measure sound pressure levels accurately. These devices are essential for assessing noise levels in various environments and determining the effectiveness of sound attenuation measures.
How Sound Level Meters Work
Sound level meters typically consist of a microphone, a preamplifier, processing circuitry, and a display. The microphone detects sound pressure variations, converting them into electrical signals.
These signals are then amplified, processed, and displayed as decibel readings. Modern SLMs often incorporate digital signal processing (DSP) to provide more sophisticated analysis and filtering capabilities.
Key Features and Settings
Sound level meters come with various settings that affect the measurement results. Understanding these settings is critical for obtaining accurate and meaningful data.
Some important settings include:
-
Frequency Weighting (A, C, Z): Frequency weighting filters emphasize certain frequencies while attenuating others. A-weighting is commonly used to approximate the human ear's sensitivity to different frequencies, while C-weighting is more linear and suitable for measuring low-frequency sounds. Z-weighting is a zero weighting and measures all frequencies equally.
-
Time Weighting (Fast, Slow, Impulse): Time weighting determines how the SLM responds to fluctuating sound levels. Fast weighting has a short response time (125 ms), making it suitable for capturing rapidly changing sounds. Slow weighting has a longer response time (1 second), providing a more averaged reading. Impulse weighting captures very short bursts of sound.
-
Measurement Parameters (Leq, Lmax, Lmin): SLMs can measure various parameters, including:
- Leq (Equivalent Continuous Sound Level): The average sound level over a specific period.
- Lmax (Maximum Sound Level): The highest sound level recorded during the measurement period.
- Lmin (Minimum Sound Level): The lowest sound level recorded during the measurement period.
Proper Usage and Considerations
To obtain accurate and reliable measurements, it's essential to use sound level meters correctly. Here are some important considerations:
- Calibration: Regularly calibrate the SLM using a known sound source to ensure accuracy.
- Microphone Placement: Position the microphone at the appropriate height and distance from the sound source, avoiding obstructions.
- Environmental Conditions: Be aware of environmental factors such as wind and temperature, which can affect measurements.
- Measurement Duration: Record measurements for a sufficient duration to capture representative sound levels.
By understanding the principles of sound measurement and utilizing sound level meters effectively, you can gain valuable insights into the acoustic environment and make informed decisions regarding sound attenuation strategies. This objective data is paramount for achieving desired outcomes and creating quieter, more comfortable spaces.
Video: Attenuation Coefficient: Soundproof Your Home Now!
FAQs About Soundproofing Your Home
What is an attenuation coefficient and why is it important for soundproofing?
The attenuation coefficient is a measure of how effectively a material reduces the intensity of sound waves passing through it. A higher attenuation coefficient means the material absorbs or blocks more sound energy, making it a better choice for soundproofing.
How does the attenuation coefficient relate to the thickness of a material?
Generally, thicker materials have higher attenuation coefficients. The thicker the material, the more opportunity it has to absorb or reflect sound waves, thus reducing the sound that passes through.
Can I rely solely on a high attenuation coefficient for effective soundproofing?
While a high attenuation coefficient is important, it’s not the only factor. Proper installation and addressing all potential sound leaks (like gaps around doors and windows) are also crucial for effective soundproofing. Consider the frequency of the sound as well; some materials are better at blocking certain frequencies than others.
Where can I find the attenuation coefficient information for different soundproofing materials?
Manufacturers of soundproofing materials often provide the attenuation coefficient, sometimes listed as the Noise Reduction Coefficient (NRC) or Sound Transmission Class (STC), in their product specifications. Look for this information on their websites or in product brochures.
So, whether you're trying to block out noisy neighbors or create a home theater haven, understanding the attenuation coefficient and how different materials perform is key. Experiment, research, and get ready to enjoy the peace and quiet you deserve!