Solute Potential: Simple Guide for Plant Lovers
Hey there, plant enthusiasts! Have you ever wondered how water moves from the soil, through those amazing roots, and all the way up to the tippy-top leaves? Well, a big part of that story involves something called solute potential! Think of it this way: pure water loves to move towards areas with more "stuff" dissolved in it—like when you add fertilizer to your garden, changing the solute potential around the roots and helping your plants drink up. Scientists at institutions like the University of California, Davis, study solute potential to understand plant water relations better, especially when using tools like pressure chambers to measure leaf water potential, which is closely linked to solute potential. Even the famous plant physiologist Paul Kramer emphasized the significance of solute potential in his research on plant and soil water relationships. Understanding solute potential unlocks a whole new level of plant parenthood, ensuring your green buddies thrive!
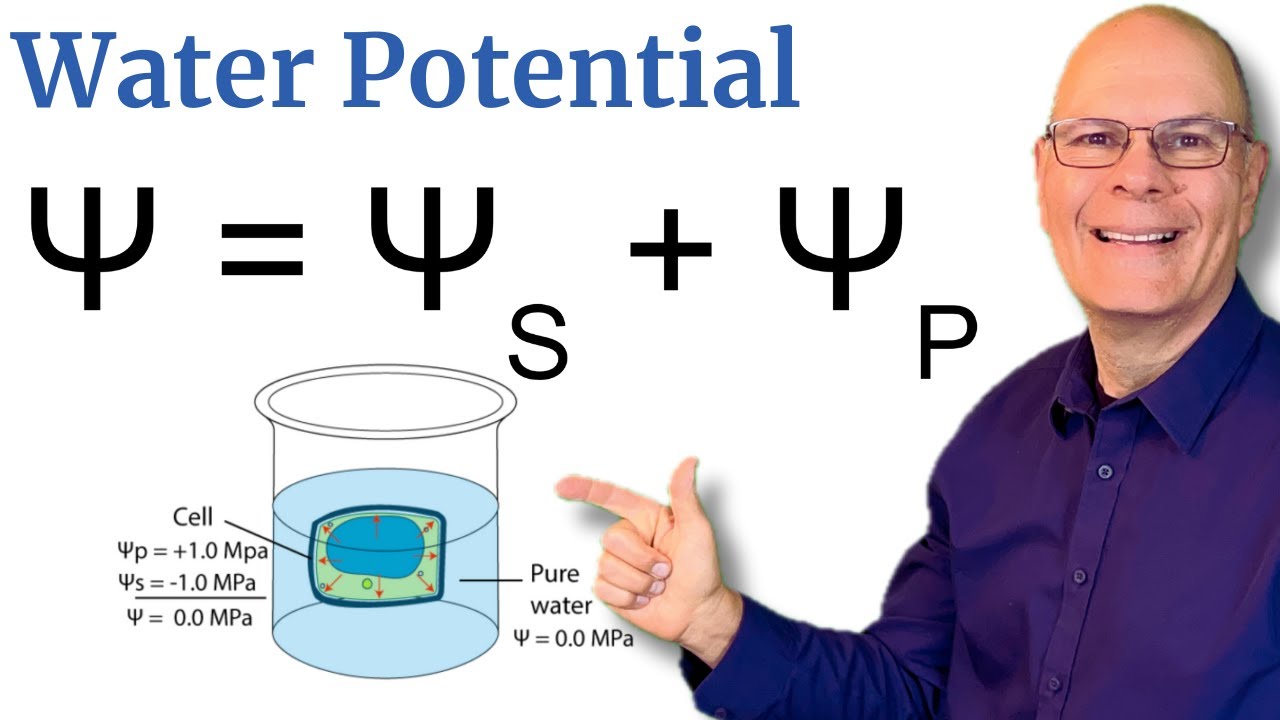
Image taken from the YouTube channel sciencemusicvideos , from the video titled Understand Water Potential! 5 Minute Explanation for AP Bio .
The Unfolding Miracle: Water's Vital Role in the Green Kingdom
Water: it's more than just a thirst quencher.
For plants, it's the lifeblood, the foundation, and the architect of their very existence! It’s easy to overlook the sheer importance of this humble molecule, but without water, the vibrant tapestry of plant life would simply unravel.
Let's dive into why water isn't just important, but absolutely essential.
Water: The Quintessential Life Force
Plants aren't just decorative additions to our world, they are complex chemical laboratories. Water serves as:
-
A crucial solvent, dissolving nutrients from the soil.
-
A reactant in photosynthesis, powering the creation of sugars.
-
A transportation medium, carrying these sugars and essential minerals throughout the plant.
Without water's dissolving and transporting capabilities, nutrients would remain locked in the soil, and the energy created by photosynthesis would be trapped within the leaves. Simply put, plant growth grinds to a halt without it.
The Intricacies of Hydro-Logistics
Water transport in plants is no simple feat.
It's a carefully orchestrated process involving osmosis, capillary action, and the cohesion-tension theory.
Imagine water molecules defying gravity, climbing hundreds of feet from the roots to the highest leaves! This complex system is susceptible to environmental stressors: drought, salinity, and even temperature fluctuations can disrupt the delicate balance.
Understanding the intricate details of water transport unlocks the ability to better assist plant health.
Journeying Through Water's World: An Exploration
This is why we're about to embark on a journey!
We will explore:
-
Water Potential: The concept that dictates the direction of water movement.
-
Water Movement: How water navigates the plant's intricate vascular system.
-
Plant Adaptations: The clever strategies plants employ to thrive in diverse water conditions.
Prepare to discover the science behind how plants capture, transport, and utilize water, ensuring their survival and shaping the world around us.
Let's get started!
[The Unfolding Miracle: Water's Vital Role in the Green Kingdom Water: it's more than just a thirst quencher. For plants, it's the lifeblood, the foundation, and the architect of their very existence! It’s easy to overlook the sheer importance of this humble molecule, but without water, the vibrant tapestry of plant life would simply unravel. Let's...]
Water Potential (Ψ): The Driving Force Explained
Water's journey through a plant is far from random. It's a carefully orchestrated flow, dictated by something called water potential (Ψ). Think of water potential as the plant's internal GPS, guiding water molecules where they're needed most. It's a crucial concept for understanding how plants hydrate, grow, and thrive.
Defining Water Potential: The Key to Plant Hydration
So, what exactly is water potential? In simple terms, it's the potential energy of water per unit volume relative to pure water at atmospheric pressure and room temperature. It essentially measures the "eagerness" of water to move from one location to another.
Water always moves from areas of high water potential (more "eager" water) to areas of low water potential (less "eager" water). Think of it like water flowing downhill; it's all about moving from higher energy to lower energy states! This difference in potential is what drives water transport throughout the entire plant.
Water Potential: The Energy Status of Water
Imagine water molecules jostling around in a plant cell. Their movement, influenced by various factors, determines the water potential. A higher water potential indicates that water molecules have more freedom to move, while a lower water potential suggests they are more restricted or bound.
This energy status dictates the direction of water movement. Understanding water potential helps us predict and even manipulate water flow within plants!
The Components of Water Potential: A Closer Look
Water potential isn't a single, monolithic force. It's actually the sum of different components, each contributing to the overall "eagerness" of water to move. The two main players are:
-
Solute Potential (Ψs): Also known as osmotic potential, this component reflects the effect of dissolved solutes on water potential. More solutes mean lower water potential.
-
Pressure Potential (Ψp): This component represents the physical pressure on the water. In plant cells, this is often positive (turgor pressure), pushing water and increasing water potential.
Understanding these components is crucial. They allow us to decipher the complex interplay of forces driving water transport within plants.
[[The Unfolding Miracle: Water's Vital Role in the Green Kingdom Water: it's more than just a thirst quencher. For plants, it's the lifeblood, the foundation, and the architect of their very existence! It’s easy to overlook the sheer importance of this humble molecule, but without water, the vibrant tapestry of plant life would simply unravel. Let's...]
Components of Water Potential: Solute, Pressure, and Turgor
Understanding water potential is like deciphering a plant's internal language. It’s a balancing act, a delicate interplay of forces that dictate the direction and intensity of water movement. Let's dive into the crucial components that shape this fundamental property: solute potential, pressure potential, and the resulting turgor pressure.
The Impact of Solutes: Diluting Water's Potential
Imagine dropping a spoonful of sugar into a glass of water. The water is still there, but its "purity," its ability to do work, has been slightly diminished. That's essentially what happens in plant cells with the addition of solutes!
Dissolved substances like sugars, salts, and minerals reduce the water potential. They bind to water molecules, making them less available to move freely.
What is Solute Potential (Ψs)?
This effect is quantified as solute potential, also known as osmotic potential. Crucially, solute potential is always a negative value.
The more solutes present, the more negative the solute potential, and the stronger the drive for water to move into that area. Think of it as water being "drawn" towards higher solute concentrations.
Pressure Potential: Squeezing Water into Action
While solutes decrease water potential, pressure does the opposite! Imagine squeezing a water balloon – you're increasing the pressure inside. This is analogous to pressure potential in plant cells.
Turgor Pressure: The Force Within
Pressure potential (Ψp) represents the physical pressure on a solution. In plant cells, this is primarily turgor pressure – the pressure exerted by the cell membrane against the cell wall.
Turgor pressure is a positive force, pushing water outwards and contributing to a higher overall water potential. It's this pressure that gives plants their rigidity and keeps them standing tall!
Turgor Pressure: The Key to Rigidity and Growth
Turgor pressure isn't just about structural support; it's vital for many plant processes. Without sufficient turgor pressure, plants wilt. Think of a sad, drooping houseplant desperately in need of water.
Turgor pressure maintains cell rigidity, providing the framework for leaves to capture sunlight and stems to support their weight. It also plays a critical role in stomatal opening, enabling gas exchange for photosynthesis.
Essentially, turgor pressure is the engine that drives cell expansion and, therefore, plant growth. It's a constant, dynamic force that shapes a plant's form and function!
Osmosis: Water's Journey Across Cell Membranes
We've explored how water potential acts as the driving force behind water movement. Now, let's zoom in and witness the process itself.
That process, the unsung hero of cellular hydration? Osmosis!
What is Osmosis? The Simple Explanation
At its core, osmosis is the diffusion of water across a semi-permeable membrane. Imagine a delicate barrier that allows water molecules to pass freely, but restricts the movement of larger solute molecules (like sugars or salts). That's a semi-permeable membrane in action!
Water will naturally flow from an area where it's highly concentrated (high water potential) to an area where it's less concentrated (low water potential). Think of it like water flowing downhill!
Osmosis and Plant Cells: Why It Matters
This seemingly simple process is absolutely crucial for plants.
Osmosis is how plant cells maintain turgor pressure, transport nutrients, and stay alive! It's how water gets from the soil and into the cells that need it!
Without osmosis, plants would wilt, wither, and ultimately, fail to thrive.
High Water Potential vs. Low Water Potential
Let's break down that "high to low" movement a bit more. Remember water potential from our previous discussion?
-
High Water Potential: This usually means a lower solute concentration. More "free" water molecules are available to move.
-
Low Water Potential: This usually means a higher solute concentration. Water molecules are more tightly bound to the solutes, reducing their freedom to move.
The Interplay of Solute and Pressure
Now, let's reconnect osmosis to those key components of water potential: solute potential and pressure potential.
Think of solute potential as the "pull" of solutes attracting water, and pressure potential as the "push" of water already inside the cell. Osmosis is the balancing act between these forces!
-
Solute Potential: A high solute concentration lowers the water potential. This creates a stronger osmotic pull, drawing water into the area.
-
Pressure Potential: A high pressure (like turgor pressure) increases the water potential. This can counteract the solute pull, slowing or even reversing the flow of water.
In essence, osmosis is water's clever way of evening things out, ensuring that plant cells are properly hydrated and can perform their vital functions.
Solutions and Cells: Environmental Impacts
We've explored how water potential acts as the driving force behind water movement. Now, let's zoom in and witness the process itself. That process, the unsung hero of cellular hydration? Osmosis!
How External Solutions Affect Plant Cells
It's a wild world out there, and the solutions surrounding plant cells are constantly changing. Are you ready to learn how plant cells respond to various solutions? Let's jump right into it.
Hypertonic Solutions: The Great Escape
Imagine a plant cell plopped into a hypertonic solution – a solution with high solute concentration outside the cell. Water, always striving for equilibrium, will rush out of the cell and into the surrounding solution.
Think of it like this: the cell is a crowded party, and the outside world is a vast, empty dance floor. Water molecules, eager for more space, bail!
Hypotonic Solutions: A Cellular Flood
On the flip side, picture a cell swimming in a hypotonic solution, one with low solute concentration outside the cell. Now, the water wants to get into the cell to equalize things.
This is like being in a desert and finally stumbling upon an oasis. Your body craves water and absorbs it as quickly as possible. This influx of water is crucial for maintaining turgor pressure, the internal pressure that keeps plants upright and perky.
Isotonic Solutions: A State of Balance
Finally, we have isotonic solutions where the solute concentration inside the cell equals the concentration outside. In this Goldilocks zone, there's no net movement of water, and the cell maintains a happy equilibrium.
The Salinity Challenge: When Salt Attacks
Now, let's talk about a major environmental challenge: salinity. Salinity impacts solute potential, which is the overall water potential in plants! Salinity can be devastating for plants.
High Salinity, Low Water Potential
High salinity means there's a ton of salt in the soil solution. Salt messes with the water potential in a big way: the more salt, the lower the water potential.
This makes it incredibly difficult for plants to pull water from the soil because the water outside the plant has a lower water potential than inside the plant.
Impact on Water Uptake
Imagine trying to drink through a straw that's blocked. High salinity acts like that blockage, making it harder and harder for plants to suck up the water they desperately need.
Water uptake becomes slowed, and that can lead to dehydration and all sorts of problems.
Plasmolysis: The Shrinking Cell Phenomenon
What happens when a plant cell loses too much water in a hypertonic environment? The answer is Plasmolysis!
The Shrunken Cell
In a hypertonic solution, water rushes out of the cell, and the plasma membrane pulls away from the cell wall. It's like a deflating balloon inside a rigid box.
This shrinking is plasmolysis, and it's a dire situation for the plant cell.
Wilting and Cell Damage
Plasmolysis leads to wilting, the droopy, sad state of a dehydrated plant. The loss of turgor pressure means the plant can no longer stand upright.
If plasmolysis persists, it can cause permanent cell damage and even death. This is why managing salinity and water availability is so critical for plant health.
The struggle is real, but understanding these processes is the first step in helping our green friends thrive!
Water Uptake: From Soil to Root Hairs
We've explored how water potential acts as the driving force behind water movement. Now, let's zoom in and witness the process itself. That process, the unsung hero of cellular hydration? Osmosis!
It's a wild world out there, and the solutions surrounding plant cells play a critical role in their well-being. Water uptake, the very foundation of a plant's life, begins with water's journey from the soil into the plant's root system.
Let's dive into the nitty-gritty of this fascinating process!
The Root's Amazing Absorption Abilities
The journey starts in the soil, where water resides as a solution containing dissolved minerals and nutrients.
Plants, being the resourceful beings they are, have evolved intricate mechanisms to extract this life-giving liquid.
This is where the roots come in, more specifically the root hairs! These tiny, hair-like extensions of the root epidermis are where the magic truly happens.
From Soil Solution to Root Epidermis: A Step-by-Step Guide
The first step is simple diffusion. Water moves from areas of higher water potential (the soil) to areas of lower water potential (inside the root cells).
Because plants can influence their cell's water potential, roots are able to pull water into them.
Think of it like a crowded concert! People naturally flow from less crowded areas to more crowded areas. Water does the same, moving down the water potential gradient.
It is vital that the plant's roots stay in contact with enough water.
Root Hairs: The Unsung Heroes of Water Absorption
Root hairs significantly increase the surface area available for water absorption.
Imagine trying to absorb water with a sponge versus trying to absorb the same water with a thousand tiny cotton swabs. Which is going to be more efficient?
These microscopic structures act like tiny straws, reaching into the soil pores to maximize water uptake. Root hairs are able to increase the surface area of a root system by orders of magnitude.
This increased surface area allows the plant to access a larger volume of soil, ensuring a steady supply of water for survival and growth. It also allows for more mineral ion uptake.
It's a brilliant evolutionary adaptation that has allowed plants to thrive in diverse terrestrial environments!
The Significance of the Root Zone
The root zone, the area of soil directly surrounding the plant's roots, is paramount for water relations.
This zone is a dynamic environment where the plant interacts with its surroundings, affecting water availability, nutrient uptake, and microbial activity.
- Water Availability: The water content of the root zone directly impacts water uptake. Dry soil means less water available for absorption.
- Nutrient Uptake: Water carries dissolved nutrients from the soil into the plant. A healthy root zone ensures adequate nutrient supply.
- Microbial Activity: The root zone is home to a diverse community of microorganisms that play a vital role in nutrient cycling and plant health.
The root zone environment is a delicate balance that plants continuously navigate. Factors such as soil type, irrigation practices, and the presence of other organisms all influence the availability of water and nutrients in this critical zone. The root zone contains many different soil horizons!
Maintaining a healthy root zone is essential for optimal plant water relations and overall plant health.
Transpiration: Water's Ascent and Exit
We've explored how water potential acts as the driving force behind water movement. Now, let's zoom in and witness the process itself. That process, the unsung hero of cellular hydration? Osmosis!
But there’s a third wheel involved in this story, and it’s called transpiration, the process where water exits the plant! Transpiration is so much more than just "sweating." It's the engine that drives water's incredible journey. Let’s find out how.
The Evaporative Engine: How Transpiration Works
Imagine a sunny summer day. Plants are constantly losing water, mostly through tiny pores on their leaves called stomata.
It's the equivalent of us sweating but on a massive scale!
From Leaf to Air: A Water Vapor Escape
This water loss isn’t just some random leakage; it's controlled evaporation.
The water inside the leaf, particularly in the mesophyll cells, changes from liquid to vapor. This creates a humid microenvironment, which then diffuses out into the drier atmosphere through the stomata.
Think of it like a crowded concert venue – things get steamy and eventually, that moisture has to escape.
Stomata: Gatekeepers of Transpiration
Stomata aren't just holes; they're dynamic gateways! They open and close depending on environmental conditions like light, humidity, and CO2 levels.
Guard cells surrounding each stoma control their aperture. It’s like a built-in system for water conservation.
Pretty cool, right?
The Cohesion-Tension Theory: Pulling Water Uphill
Now, here’s where the magic happens!
Transpiration creates a negative pressure, a tension, at the top of the plant (leaves). This tension pulls water upwards through the xylem, the plant's vascular plumbing.
Cohesion and Adhesion: Water's Superpowers
Water molecules are incredibly cohesive.
They stick to each other due to hydrogen bonds. This cohesion allows water to be pulled up like a continuous chain.
Adhesion also plays a role: water molecules cling to the walls of the xylem vessels, counteracting gravity’s pull.
These two properties combined makes transpiration possible.
It's like water molecules are tiny acrobats, holding hands and climbing a ladder against all odds!
Transpiration's Role in the Plant's Water Balance
Transpiration isn't just about water loss, it's crucial for overall plant health.
Water Uptake: A Continuous Cycle
The tension created by transpiration pulls water up from the roots.
This ensures a continuous supply of water and dissolved minerals from the soil.
It creates a dynamic flow, keeping everything hydrated and nourished.
Temperature Regulation: Staying Cool Under Pressure
Just like sweating cools us down, transpiration helps regulate plant temperature.
Evaporation requires energy (heat), so as water evaporates from the leaves, it cools the plant down, preventing overheating.
This is particularly important in hot, sunny environments. Plants are basically using water as their own personal air conditioner!
Balancing the Equation: Water In vs. Water Out
Plants need to carefully balance water uptake and water loss.
Too much transpiration, and the plant risks dehydration and wilting.
Too little, and it could lead to overheating and reduced nutrient uptake. It’s a delicate dance.
Factors Influencing Transpiration Rate
There's a lot that affects the rate of transpiration:
- Light: Higher light intensity usually increases transpiration (stomata open for photosynthesis).
- Temperature: Warmer temperatures increase the rate of evaporation.
- Humidity: High humidity reduces the driving force for evaporation.
- Wind: Wind removes humid air from around the leaves, increasing evaporation.
Plants are constantly responding to these environmental cues, adjusting their stomatal openings to maintain a healthy water balance.
Essentially, transpiration acts as the linchpin of water movement. It’s a fantastic example of how plants have masterfully evolved to thrive in diverse environments, using nothing but water and a little bit of physics!
Living with Extremes: Plant Adaptations
Transpiration: Water's Ascent and Exit
We've explored how water potential acts as the driving force behind water movement. Now, let's zoom in and witness the process itself. That process, the unsung hero of cellular hydration? Osmosis!
But there’s a third wheel involved in this story, and it’s called transpiration, the process where water exits the plant's system. Plants aren't just passive recipients of water; they're actively adapting to the water availability (or lack thereof) in their environments. This section dives into some of the incredible strategies plants employ to thrive even when conditions get tough. Think of it as plant-life survival skills 101!
The Art of Osmoregulation
At the heart of plant adaptation lies osmoregulation: The ability to actively manage their internal osmotic pressure. Plants aren't just at the mercy of their surroundings.
They can tweak the concentration of solutes within their cells. Plants tweak this to ensure water flows in the direction they need, rather than the direction the exterior solutions force them. It's like having an internal thermostat for water balance!
This process is especially crucial in fluctuating environments where the external water potential can change rapidly.
Conquerors of Salinity: Halophytes to the Rescue!
Some plants don’t just tolerate salt. They embrace it! These salt-loving heroes are called halophytes. They've evolved ingenious ways to survive in soils where most plants would wither.
One common strategy? Salt exclusion. Some halophytes have specialized root structures that prevent the uptake of excess salt.
Other halophytes accumulate salt in specific compartments, like vacuoles, keeping it away from sensitive cellular processes. Then, there are those that excrete excess salt through special glands on their leaves. It's like they're sweating salt!
Dry Times? No Problem: Adaptations to Arid Environments
Arid environments present a different set of challenges: extreme water scarcity. Plants in these regions have developed a remarkable array of adaptations to conserve every precious drop.
Deep roots tap into groundwater sources far below the surface, while reduced leaf size minimizes water loss through transpiration.
Some plants, like succulents, have thick, fleshy leaves and stems that store water for long periods. Others employ ingenious strategies like CAM (crassulacean acid metabolism) photosynthesis. CAM helps reduce water loss by opening stomata only at night.
Coastal Resilience: Battling Salt Spray and Tides
Coastal environments combine the challenges of salinity with the added stress of salt spray and fluctuating water levels due to tides. Coastal plants need to be tough.
Many coastal species have salt-tolerant tissues and waxy coatings on their leaves to minimize salt uptake. The waxy coatings also protects against drying winds.
They often have specialized root systems that anchor them firmly in the sandy soil and withstand the force of waves.
Specific Environment Spotlight: Salt Marshes
Salt marshes are amazing natural water filters. They are also some of the most productive ecosystems on Earth. The plants living in salt marshes, such as Spartina grasses, are perfectly adapted to the harsh conditions.
They tolerate high salinity and periodic flooding by using strategies like salt excretion and aerenchyma. Aerenchyma allows for oxygen transport to submerged roots.
Navigating Agricultural Fields: The Impact of Irrigation
Irrigation, while essential for crop production in many regions, can inadvertently lead to soil salinization. This is especially true in arid and semi-arid areas where evaporation rates are high.
When irrigation water evaporates, it leaves behind salts that accumulate in the topsoil. Over time, this can create conditions that are unfavorable for plant growth.
Careful irrigation management is essential to prevent salinization and ensure the long-term sustainability of agricultural practices. This includes using appropriate irrigation techniques, monitoring soil salinity levels, and implementing drainage systems to remove excess salt.
The Role of Soil in Plant Water Relations
We've explored how plants ingeniously adapt to diverse environmental conditions, from the driest deserts to saline coastal regions. But let's dig deeper – quite literally! What about the silent partner in this dance, the very foundation that anchors our green friends and provides them with life's elixir? I'm talking, of course, about soil.
Soil isn't just dirt; it's a dynamic ecosystem, a complex matrix that profoundly influences a plant's ability to access water. Understanding how different soil types affect water availability is crucial for cultivating thriving, resilient plants. So, let's get our hands dirty and explore the fascinating world of soil and its impact on plant hydration!
Soil Type and Water Availability: It's All About Texture
Different soil types, with their varying particle sizes, dictate how easily water infiltrates, is retained, and becomes available to plants. This is where soil texture comes into play.
Think of it this way:
-
Sandy soils: These soils are composed of large particles with ample space between them. Water drains quickly, making them well-aerated but also prone to drying out rapidly. They offer little resistance, which means water is hardly retained.
-
Clay soils: These soils consist of tiny, tightly packed particles. They retain water exceptionally well but can become waterlogged and poorly aerated. They're like water-hoarding dragons, which means water is harder to extract.
-
Loamy soils: The Goldilocks of soil types! Loam is a balanced mixture of sand, silt, and clay particles. It offers good drainage, adequate water retention, and sufficient aeration, making it ideal for most plants. Think of them as soil superheroes.
The particle size distribution directly affects the capillary action within the soil. Smaller pores in clay soils allow for greater capillary action, drawing water upwards, but also holding it more tightly. Larger pores in sandy soils mean less capillary action and faster drainage.
The Marvel of Soil Structure
Beyond texture, soil structure – the arrangement of soil particles into aggregates – plays a significant role.
-
Well-structured soils: These soils have a crumbly, porous structure that promotes water infiltration, drainage, and aeration. They're like miniature sponges, ready to absorb and release water.
-
Poorly structured soils: These soils may be compacted, hindering water movement and root growth. Think of these as pavement for roots. Compaction leads to reduced pore space, limiting water infiltration and retention.
Organic matter, the decomposed remains of plants and animals, is a crucial ingredient for improving soil structure. It acts like a glue, binding soil particles together into stable aggregates, enhancing water infiltration and retention.
Organic Matter: The Soil's Hydration Hero
Increasing the organic matter content of your soil is one of the best things you can do for your plants.
Here's why:
-
Improved Water Retention: Organic matter acts like a sponge, absorbing and holding onto water.
-
Enhanced Soil Structure: It promotes the formation of stable soil aggregates, improving drainage and aeration.
-
Increased Nutrient Availability: Organic matter releases nutrients slowly, providing a sustained source of food for plants.
Amend your soil with compost, aged manure, or other organic materials to improve its water-holding capacity and overall health. Your plants will thank you!
Soil Solute Potential: A Hidden Influence
While we often focus on soil texture and structure, the solute potential of the soil solution also impacts water availability. High concentrations of salts or other dissolved substances in the soil lower the water potential, making it harder for plants to extract water.
This is particularly relevant in arid regions or areas with heavy fertilizer use. Excess fertilizer application can create a high solute concentration in the soil, effectively creating a "physiological drought" even when water is present.
-
Monitor Soil Salinity: Regularly test your soil to check for high salt levels.
-
Avoid Over-Fertilizing: Use fertilizers judiciously and follow recommended application rates.
-
Leach Excess Salts: In areas with high salinity, leach the soil with plenty of fresh water to flush out excess salts.
Understanding the complex interplay between soil type, texture, structure, organic matter, and solute potential is essential for optimizing plant water relations. By managing these factors effectively, you can create a soil environment that promotes healthy, vigorous growth and ensures that your plants have access to the water they need to thrive.
Wilting: A Visual Sign of Water Stress
[The Role of Soil in Plant Water Relations We've explored how plants ingeniously adapt to diverse environmental conditions, from the driest deserts to saline coastal regions. But let's dig deeper – quite literally! What about the silent partner in this dance, the very foundation that anchors our green friends and provides them with life's elixir? I'...]
Wilting. It's the droopy, disheartening sight no plant lover wants to see. But it's more than just an aesthetic issue. Wilting is a distress signal, a clear and visible indication that something is amiss in a plant's water balance. Let's break down what wilting really means and how it impacts plant health.
Decoding the Droop: What is Wilting?
At its core, wilting is the loss of rigidity in plant tissues. Healthy plants stand upright because their cells are full of water, creating turgor pressure against the cell walls. When a plant loses water faster than it can replenish it, this pressure decreases, causing the leaves and stems to sag.
Think of it like this: a fully inflated balloon stands tall and firm. But slowly let the air out, and the balloon collapses. Wilting is essentially the plant version of a deflated balloon.
The Culprits Behind the Collapse: Causes of Wilting
Several factors can trigger wilting, and understanding them is key to rescuing your thirsty plants. The most common cause, of course, is underwatering. If the soil is dry, the plant can't absorb enough water to maintain turgor pressure.
But wilting can also occur even when the soil seems moist. How is this possible?
-
Overwatering: Believe it or not, too much water can lead to wilting. Soggy soil deprives roots of oxygen, hindering their ability to absorb water. It's a counterintuitive form of drought stress!
-
Root Damage: Damaged roots, whether from pests, diseases, or physical injury, cannot efficiently take up water.
-
High Transpiration Rates: On hot, sunny, or windy days, plants lose water rapidly through transpiration. If water loss exceeds uptake, wilting occurs.
-
Salt Stress: High salt concentrations in the soil can lower the water potential, making it difficult for plants to absorb water, even if the soil is technically moist.
-
Pathogens: Certain pathogens can disrupt the plant's vascular system, blocking water transport and leading to wilting.
The Physiology of the Faint: How Wilting Works
Wilting isn't just a passive collapse; it's a physiological response to water stress. When water becomes scarce, the plant prioritizes survival.
-
Loss of Turgor Pressure: As mentioned, the initial trigger is the decline in turgor pressure within individual cells.
-
Plasmolysis: In severe cases, the cell membrane pulls away from the cell wall in a process called plasmolysis. This is often irreversible cell damage.
-
Stomatal Closure: Plants may close their stomata (tiny pores on leaves) to reduce water loss through transpiration. However, this also limits carbon dioxide intake, hindering photosynthesis.
-
Hormonal Signals: The plant produces hormones like abscisic acid (ABA), which signals for stomatal closure and other drought responses.
Beyond the Droop: Consequences of Prolonged Wilting
Wilting is a warning sign, and ignoring it can have serious consequences for plant health.
-
Reduced Photosynthesis: Stomatal closure, a common response to wilting, limits carbon dioxide uptake, hindering photosynthesis and reducing energy production.
-
Stunted Growth: Lack of water stress impairs cell division and expansion, leading to stunted growth and reduced yields (in crops).
-
Increased Susceptibility to Disease: Stressed plants are more vulnerable to pests and diseases.
-
Permanent Damage: Prolonged or severe wilting can cause irreversible damage to plant tissues, leading to leaf drop, branch dieback, and, ultimately, plant death.
-
Reduced Crop Yield: In agricultural settings, water deficits during critical periods of plant development such as flowering or grain filling can severely impact crop productivity.
So, the next time you see your plants looking a little droopy, don't ignore the signal. Investigate the cause, address the underlying issue, and give your plants the hydration they need to thrive!
Video: Solute Potential: Simple Guide for Plant Lovers
FAQ: Solute Potential for Plant Lovers
What exactly are solutes, and how do they impact water movement in my plants?
Solutes are dissolved substances like sugars and salts within a plant's cells. The more solutes there are, the lower (more negative) the solute potential. This lower solute potential draws water into the cell, as water moves from areas of high water potential to areas of low water potential.
Why is a "more negative" solute potential considered desirable for plant health?
A more negative solute potential means a higher concentration of solutes in the cell. This draws water into the cell more effectively. Plants need water to perform essential functions like photosynthesis and nutrient transport, so having a strong ability to draw water is crucial.
How does fertilization affect a plant's solute potential?
Fertilizers contain salts, which, when dissolved in the plant's cells, increase the solute concentration and lower (make more negative) the solute potential. However, over-fertilizing can lead to excessively negative solute potential outside the roots, causing water to move out of the plant, resulting in fertilizer burn.
Can understanding solute potential help me troubleshoot watering problems?
Yes! If a plant is wilting even with moist soil, the solute potential might be out of balance. Perhaps the soil has too many salts (high solute concentration, low water potential) due to over-fertilization. Understanding solute potential can help you adjust your watering and fertilization practices for optimal plant health.
So, next time you're tending to your green babies and notice some wilting, remember it might not just be about watering! Keep solute potential in mind, and consider if excessive fertilization or overly salty soil could be playing a role. A little understanding goes a long way in keeping those plants happy and thriving!