Sodium Neutrons: Unveiling the Secrets! 60/60
Nuclear reactors, as complex systems, utilize neutron moderators to control the speed of neutrons essential for sustained nuclear reactions. Understanding the behavior of neutron cross-sections, a crucial aspect of nuclear physics, is paramount in predicting reactor performance. Exploration of sodium neutrons reveals intricate relationships between sodium isotopes and neutron interactions, studied extensively at facilities like the Oak Ridge National Laboratory. Investigation into sodium neutrons requires a deep dive into theoretical models and experimental validation, to further understand nuclear energy applications.
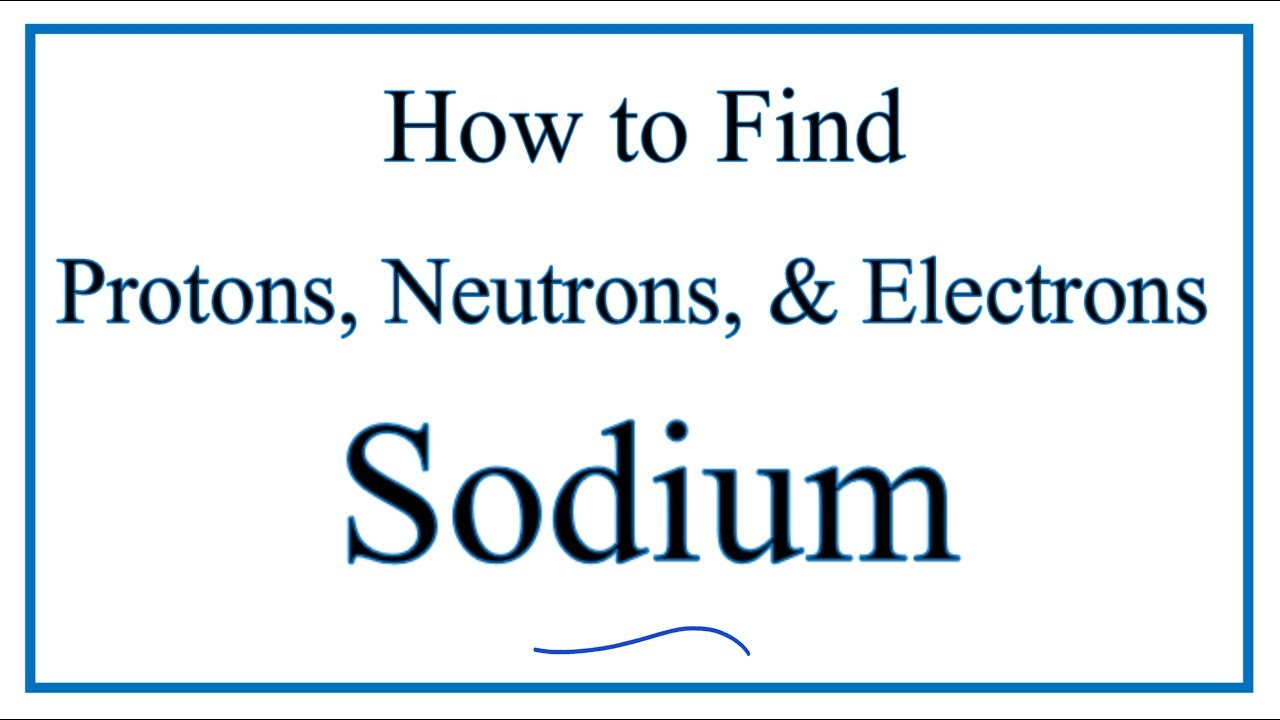
Image taken from the YouTube channel Wayne Breslyn (Dr. B.) , from the video titled How to find the Number of Protons, Electrons, Neutrons for Sodium (Na) .
Sodium and neutrons, seemingly disparate entities, are in fact deeply intertwined in the realm of nuclear physics and technology. Their interactions, governed by the fundamental laws of nature, underpin critical applications ranging from energy production to materials science. This exploration into their relationship will reveal the profound significance of their dance at the atomic level.
Sodium (Na): An Alkali Metal with a Nuclear Role
Sodium, denoted by the symbol Na, is an alkali metal characterized by its single valence electron. This electronic configuration dictates its high reactivity and its propensity to form ionic compounds. While familiar in everyday life, sodium's role extends far beyond table salt and streetlights.
Within the context of nuclear physics, sodium's nuclear properties become paramount. Its ability to interact with neutrons, either absorbing or scattering them, directly impacts the performance and safety of nuclear reactors. These interactions determine the rate of nuclear reactions and influence the overall neutron economy within a reactor core.
Neutrons: The Unsung Heroes of the Nucleus
Neutrons, neutral subatomic particles residing within the nucleus of an atom, are the linchpins of nuclear stability. Possessing a mass slightly greater than that of a proton, neutrons contribute significantly to the overall mass of an atom. Their presence is critical for overcoming the electrostatic repulsion between positively charged protons, holding the nucleus together.
Moreover, neutrons are the primary drivers of nuclear reactions. Their ability to induce fission in certain isotopes, like uranium-235, forms the basis of nuclear power generation. The controlled chain reaction, sustained by neutrons, releases tremendous amounts of energy that can be harnessed for electricity production.
The Interplay: A Foundation for Innovation
The multifaceted relationship between sodium and neutrons is the central theme of this exploration. Their interactions are not merely theoretical curiosities, but rather the foundation for practical applications that shape our world. From the design of advanced nuclear reactors to the development of novel materials, understanding this interplay is crucial.
This article aims to unravel the complexities of this relationship, elucidating the fundamental principles that govern their interactions and showcasing the diverse applications that arise from their unique properties. By exploring the nuances of their dance at the atomic level, we can gain a deeper appreciation for their significance in shaping our technological landscape.
Sodium and Neutrons: A Tale of Two Particles
The controlled chain reaction, sustained by neutrons, represents just one facet of their complex relationship with other elements. Sodium, a seemingly ordinary metal, also plays a vital role in this nuclear narrative. Understanding the individual characteristics of these two particles is crucial before we can fully appreciate the significance of their interactions.
Sodium (Na): An Alkali Metal
Sodium, represented by the symbol Na, occupies a prominent position as an alkali metal in the periodic table. Its atomic number, 11, reflects the count of protons within its nucleus, balanced by an equal number of electrons in a neutral atom.
Atomic Structure and Electronic Configuration
The atomic structure of sodium dictates its chemical behavior. The nucleus, composed of 11 protons and typically 12 neutrons (for the common isotope Na-23), is surrounded by 11 electrons arranged in distinct energy levels or shells.
Specifically, sodium possesses an electronic configuration of 1s² 2s² 2p⁶ 3s¹. This single electron in the outermost (valence) shell is what defines its alkali metal characteristics and its propensity to readily form positive ions.
Key Properties and Reactivity
As an alkali metal, sodium exhibits a characteristic set of properties. It is a soft, silvery-white metal that can be easily cut with a knife. This softness is attributed to the relatively weak metallic bonding resulting from its single valence electron.
Sodium demonstrates exceptional thermal and electrical conductivity, making it valuable in various applications. However, its most notable attribute is its high reactivity. Sodium reacts vigorously with water, oxygen, and other elements, releasing significant heat and often forming flammable hydrogen gas.
Common Compounds
Due to its high reactivity, sodium is rarely found in its elemental form in nature. Instead, it exists primarily in various compounds.
Sodium chloride (NaCl), or common table salt, is perhaps the most well-known sodium compound, essential for biological functions and various industrial processes. Other important compounds include sodium hydroxide (NaOH), a strong base used in manufacturing and cleaning, and sodium carbonate (Na₂CO₃), or soda ash, utilized in glass production and detergents.
Neutrons: The Neutral Nucleons
Neutrons, as their name suggests, are electrically neutral subatomic particles found within the nucleus of an atom. Alongside protons, they constitute the nucleons, the fundamental building blocks of atomic nuclei.
Mass, Charge, and Spin
Neutrons possess a mass slightly greater than that of a proton, approximately 1.675 × 10⁻²⁷ kg. Unlike protons, which carry a positive charge, neutrons have no electric charge. This neutrality is crucial for their role in nuclear stability.
Neutrons also possess an intrinsic angular momentum called spin, a quantum mechanical property described by the spin number ½. This spin contributes to the overall angular momentum of the nucleus and influences nuclear properties.
Role in Nuclear Stability and Isotopes
The presence of neutrons within the nucleus is critical for overcoming the electrostatic repulsion between positively charged protons. Without neutrons, the repulsive forces would cause the nucleus to disintegrate.
The number of neutrons in a nucleus determines the isotope of an element. Isotopes are atoms of the same element that have the same number of protons but different numbers of neutrons. For example, sodium has several isotopes, including the stable Na-23 (11 protons, 12 neutrons) and the radioactive Na-24 (11 protons, 13 neutrons). The varying neutron numbers in isotopes influence their nuclear properties and stability.
The Dance of Interactions: Nuclear Physics Principles
Having established the individual characteristics of sodium and neutrons, the stage is set to explore the intricate dance of interactions that occur between them. These interactions, governed by the principles of nuclear physics, form the bedrock for understanding more complex applications such as nuclear reactors and material science investigations.
Foundations of Nuclear Interactions
At the heart of these interactions lies the nuclear force, a powerful force that binds protons and neutrons together within the nucleus, counteracting the electrostatic repulsion between the positively charged protons. This strong force operates over extremely short distances and is responsible for the stability of atomic nuclei.
Nuclear reactions occur when a nucleus interacts with another particle, such as a neutron. These reactions can lead to the formation of new isotopes, the release of energy, or the scattering of particles. The probability of a specific nuclear reaction occurring is quantified by its cross-section, a measure of the effective area that the nucleus presents to the incoming particle.
Neutron Interactions with Sodium Nuclei
Neutrons, being electrically neutral, can approach the positively charged nucleus without experiencing any Coulomb repulsion. This allows them to interact with nuclei in a variety of ways, including neutron capture and neutron scattering.
Neutron Capture
Neutron capture occurs when a neutron is absorbed by a sodium nucleus, resulting in the formation of a heavier isotope of sodium. For example, when a sodium-23 (23Na) nucleus captures a neutron, it becomes sodium-24 (24Na):
23Na + n → 24Na
The newly formed isotope, 24Na, is radioactive and undergoes beta decay with a half-life of approximately 15 hours. This neutron capture reaction is important to consider when sodium is used as a coolant in nuclear reactors, as the formation of radioactive 24Na can contribute to the overall radioactivity of the reactor system.
Neutron Scattering
Neutron scattering involves the collision of a neutron with a sodium nucleus, resulting in a change in the neutron's direction and energy. There are two main types of neutron scattering: elastic and inelastic.
Elastic Scattering
In elastic scattering, the total kinetic energy of the neutron and the sodium nucleus is conserved. The neutron simply bounces off the nucleus, transferring some of its momentum to the nucleus. This type of scattering is important for slowing down neutrons in nuclear reactors, as it allows the neutrons to reach thermal energies where they are more likely to induce fission.
Inelastic Scattering
In inelastic scattering, some of the neutron's kinetic energy is transferred to the sodium nucleus, exciting it to a higher energy state. The excited nucleus then decays back to its ground state, emitting a gamma ray. Inelastic scattering can play a role in the moderation of neutrons, as it reduces the energy of the neutron, while the emitted gamma ray is another factor to consider in reactor design.
Fission and Sodium Isotopes
While sodium is not typically considered a fissile material, meaning it cannot sustain a chain reaction of nuclear fission, it's important to examine its role in the context of fission. Naturally occurring sodium (23Na) does not undergo fission. While fast neutrons can theoretically induce fission in heavier isotopes of sodium, the probability of this occurring is extremely low, and it is not a significant factor in practical applications.
Having explored the fundamental interactions between sodium and neutrons, and the nuclear principles that govern them, we can now turn our attention to a practical application where these interactions are harnessed: Sodium-Cooled Fast Reactors. These reactors represent a significant advancement in nuclear technology, utilizing sodium's unique properties to achieve efficient energy production.
Sodium-Cooled Fast Reactors: Where Sodium and Neutrons Collide
Sodium-Cooled Fast Reactors (SFRs) represent a distinct class of nuclear reactors with the potential to revolutionize nuclear energy production. Their core purpose lies in generating electricity through nuclear fission, while simultaneously offering advantages in terms of fuel efficiency, waste management, and inherent safety features.
SFRs utilize liquid sodium as a primary coolant, a design choice driven by sodium's exceptional thermal properties and its relatively low impact on neutron behavior.
Sodium as a Coolant: Advantages and Challenges
The selection of sodium as a coolant in SFRs is not arbitrary; it stems from a carefully considered balance of its beneficial properties and the challenges associated with its use.
Advantages of Sodium Coolant
Sodium's high thermal conductivity is a key advantage, allowing for efficient heat removal from the reactor core. This is crucial for preventing overheating and maintaining stable operating conditions.
Additionally, sodium's low neutron absorption cross-section means it does not readily absorb neutrons, preserving the neutron economy within the reactor and enhancing its ability to sustain a chain reaction and breed more fuel.
Challenges of Sodium Coolant
Despite its benefits, sodium presents certain operational challenges.
Perhaps the most significant is its high reactivity with water and air. This necessitates careful design and engineering to prevent any contact between sodium and these substances, as such interactions can lead to violent reactions and the release of radioactive materials.
Furthermore, handling liquid sodium requires specialized equipment and procedures due to its opacity, corrosiveness, and the potential for activation, which turns it radioactive, requiring remote handling.
Neutron Economy within an SFR
The neutron economy refers to the balance of neutron production, absorption, and leakage within the reactor core. SFRs are designed to optimize this balance to achieve a high breeding ratio and efficient fuel utilization.
Breeding Ratio
The breeding ratio is a key metric for SFRs, indicating the reactor's ability to produce more fissile material than it consumes. A breeding ratio greater than one signifies that the reactor is breeding fuel, effectively extending the lifespan of nuclear fuel resources.
Neutron Spectrum
SFRs operate with a fast neutron spectrum, meaning that the neutrons within the reactor core have relatively high energies. This is achieved by minimizing the use of neutron moderators, which slow down neutrons.
A fast neutron spectrum is essential for achieving a high breeding ratio and for efficiently fissioning certain actinides present in nuclear waste.
Efficient Electricity and Energy Production
The design and operational characteristics of SFRs contribute to their potential for efficient electricity and energy production.
By achieving a high breeding ratio, SFRs can extend the lifespan of nuclear fuel resources and reduce the need for frequent refueling.
Furthermore, the fast neutron spectrum allows for the efficient fissioning of actinides, which are long-lived radioactive elements present in nuclear waste. This can contribute to reducing the volume and radiotoxicity of nuclear waste.
In conclusion, Sodium-Cooled Fast Reactors represent a promising avenue for nuclear energy production, offering potential advantages in terms of fuel efficiency, waste management, and sustainability. While challenges remain in handling liquid sodium, ongoing research and development efforts are focused on addressing these challenges and further enhancing the performance and safety of SFR technology, while contributing to efficient energy production.
Having established the role of sodium as a critical component in reactor cooling, especially in fast reactor designs where neutron economy is paramount, it is important to appreciate how neutrons themselves can be used to probe the properties of sodium-containing materials. This is achieved through neutron scattering, a suite of techniques that exploit the wave-particle duality of neutrons to reveal information about the structure and dynamics of matter.
Probing the Atomic World: Neutron Scattering Techniques
Neutron scattering is a powerful tool for investigating the microscopic properties of materials. It provides insights into the arrangement of atoms and their motions.
Fundamentals of Neutron Scattering
At its core, neutron scattering involves directing a beam of neutrons at a sample and analyzing the resulting scattering pattern. The manner in which neutrons interact with the sample's atoms depends on several factors, including the neutron's energy, the type of nuclei in the sample, and the arrangement of these nuclei.
Elastic Scattering
Elastic scattering occurs when neutrons collide with atoms and are deflected without any loss of energy. This type of scattering provides information about the static structure of the material. It reveals the positions of atoms and the overall arrangement of the crystal lattice, if present.
Inelastic Scattering
In contrast, inelastic scattering involves neutrons exchanging energy with the sample. This exchange can either excite the material (neutron loses energy) or de-excite it (neutron gains energy). By measuring the energy and momentum changes of the neutrons, scientists can probe the dynamics of the material, such as atomic vibrations (phonons), magnetic excitations (magnons), and molecular motions.
Studying Sodium-Containing Materials
Neutron scattering is particularly useful for studying materials containing light elements like sodium, where X-ray diffraction techniques may be less sensitive. Several types of sodium-containing materials can be effectively investigated:
- Sodium Oxides: Neutron diffraction can elucidate the crystal structure of different sodium oxides (e.g., Na₂O, Na₂O₂), which are important in various chemical and industrial processes.
- Sodium Alloys: Neutron scattering can probe the atomic arrangement and dynamics in sodium alloys, helping understand their mechanical and thermal properties.
- Sodium Intercalated Compounds: Many materials can incorporate sodium ions within their structure (e.g., battery materials). Neutron scattering is used to track the location and mobility of these ions.
Applications and Insights
The information obtained from neutron scattering experiments on sodium-containing materials has broad applications across various scientific and technological fields:
- Material Structure Determination: Precisely determining the atomic positions and crystal structure of novel sodium compounds.
- Dynamics and Excitations: Characterizing the vibrational and magnetic properties of materials, providing insights into their thermal and electronic behavior.
- Phase Transitions: Studying how materials change their structure and properties as a function of temperature or pressure, such as the melting of sodium metal or structural transitions in sodium oxides.
- Battery Research: Understanding the diffusion mechanisms of sodium ions in battery electrode materials, leading to the development of more efficient energy storage devices.
- Geological Sciences: Investigating the structure and composition of sodium-bearing minerals under extreme conditions relevant to the Earth's mantle.
By exploiting the unique properties of neutrons, scattering techniques provide detailed insights into the structure and dynamics of sodium-containing materials that are often inaccessible by other methods. This capability is vital for advancing our understanding of fundamental materials science and developing new technologies.
Having explored how neutron scattering unveils the structural and dynamic properties of sodium-containing materials, we turn our attention to another powerful technique that leverages neutron interactions: Neutron Activation Analysis (NAA). This method provides a unique means of identifying and quantifying trace elements within a sodium matrix, a capability of significant importance for quality control and materials characterization.
Unlocking Elemental Secrets: Neutron Activation Analysis with Sodium
Neutron Activation Analysis (NAA) stands as a highly sensitive nuclear technique employed to determine the elemental composition of materials. Its application is particularly valuable in scenarios where trace element quantification is critical, such as in the analysis of high-purity sodium used in nuclear reactors.
The Principles of Neutron Activation Analysis
At its essence, NAA relies on the principle of inducing radioactivity in a sample through neutron bombardment. When a sample is exposed to a flux of neutrons, the nuclei of its constituent elements can capture these neutrons, transforming them into radioactive isotopes.
These newly formed radioactive isotopes are unstable and will subsequently decay, emitting characteristic gamma rays in the process. The energy of these gamma rays is unique to each element, acting as a fingerprint for its identification.
By carefully measuring the energies and intensities of the emitted gamma rays, scientists can qualitatively identify which elements are present in the sample and quantitatively determine their concentrations.
NAA in Sodium Analysis: Unveiling Impurities
The application of NAA is particularly relevant in the context of sodium analysis due to the stringent purity requirements for sodium used in nuclear reactor cooling systems. Even trace amounts of impurities can significantly impact the performance and safety of these reactors.
NAA provides a powerful tool for identifying and quantifying these impurities, ensuring that the sodium meets the required specifications.
Identifying Contaminants
The high sensitivity of NAA allows for the detection of a wide range of elements that might be present in sodium, including metallic impurities (e.g., iron, chromium, nickel) and non-metallic contaminants (e.g., chlorine, sulfur).
By analyzing the gamma-ray spectrum emitted from an irradiated sodium sample, researchers can pinpoint the presence of these elements, even at extremely low concentrations (parts per billion levels).
Quantifying Impurity Levels
Beyond mere identification, NAA enables the precise quantification of impurity concentrations. The intensity of the gamma ray emitted by a particular element is directly proportional to its concentration in the sample.
By comparing the measured gamma-ray intensity to that of known standards, the concentration of the element can be determined with high accuracy. This quantitative information is crucial for assessing the suitability of sodium for its intended application in nuclear reactors.
Applications in Quality Control and Materials Characterization
NAA plays a pivotal role in ensuring the quality of sodium used in nuclear reactors. It is used to monitor the purity of sodium during production, storage, and operation.
By regularly analyzing sodium samples using NAA, operators can detect any potential contamination issues early on and take corrective actions to prevent adverse effects on reactor performance.
Moreover, NAA is valuable in materials characterization studies, providing insights into the elemental composition of sodium alloys and other sodium-containing materials. This information is essential for understanding the behavior of these materials under reactor operating conditions.
Having explored how neutron scattering unveils the structural and dynamic properties of sodium-containing materials, we turn our attention to another powerful technique that leverages neutron interactions: Neutron Activation Analysis (NAA). This method provides a unique means of identifying and quantifying trace elements within a sodium matrix, a capability of significant importance for quality control and materials characterization.
Isotopes and Cross-Sections: Quantifying Interactions
The interaction between neutrons and sodium isn't a uniform process. It's heavily dependent on the specific isotope of sodium involved. Each isotope possesses a unique nuclear structure. This leads to varying probabilities of interaction with neutrons, a concept quantified by neutron cross-sections.
The Isotopic Landscape of Sodium
Sodium, in its naturally occurring state, is predominantly composed of the isotope Sodium-23 (²³Na). It constitutes nearly 100% of naturally found sodium. This makes ²³Na the primary player in most sodium-neutron interactions.
However, other isotopes can be formed through nuclear reactions. A notable example is Sodium-24 (²⁴Na), a radioactive isotope. It is produced through neutron capture by ²³Na.
²⁴Na is significant because of its radioactive decay. It has a half-life of approximately 15 hours. This makes it useful in certain applications. These applications are such as tracing fluid flow or as an indicator in NAA, as previously discussed.
The existence and abundance of these isotopes, particularly ²³Na and ²⁴Na, directly impact the overall behavior of sodium in neutron environments.
Understanding Neutron Cross-Sections
Neutron cross-section is a measure of the probability of a specific nuclear reaction occurring between a neutron and a nucleus. It is measured in units of barns (1 barn = 10⁻²⁸ m²).
Different isotopes exhibit different cross-sections for various neutron interactions.
The key types of neutron cross-sections include:
-
Absorption Cross-Section (σₐ): This represents the probability that a neutron will be absorbed by the nucleus, leading to the formation of a heavier isotope or a nuclear reaction.
-
Scattering Cross-Section (σₛ): This represents the probability that a neutron will be scattered by the nucleus, changing its direction and energy. Scattering can be further divided into elastic and inelastic scattering, depending on whether kinetic energy is conserved.
-
Fission Cross-Section (σ𝒻): Although not typically significant for sodium itself, this represents the probability that a neutron will induce nuclear fission in the nucleus. It is relevant in the context of surrounding fissile materials in a reactor core.
Isotopic Variations in Cross-Sections
The neutron cross-sections for ²³Na and ²⁴Na differ significantly. ²³Na has a relatively low absorption cross-section for thermal neutrons. This is one of the key reasons it's used as a coolant in fast reactors.
It allows neutrons to propagate through the coolant with minimal absorption. ²⁴Na, being a radioactive isotope, has its own set of cross-sections. They govern its formation and subsequent decay.
These cross-sections are energy-dependent. This means the probability of interaction varies with the neutron's kinetic energy. This energy dependence is crucial for reactor design and safety calculations.
Implications for Nuclear Applications
The isotopic composition of sodium and their respective neutron cross-sections have profound implications for nuclear applications:
-
Reactor Design: Accurate knowledge of these cross-sections is crucial for modeling neutron transport within reactors, predicting reaction rates, and ensuring criticality control.
-
Safety Analysis: Understanding how neutrons interact with sodium isotopes is essential for assessing the consequences of potential accidents. This includes scenarios involving coolant voiding or the formation of radioactive isotopes.
-
Waste Management: The production of radioactive isotopes like ²⁴Na contributes to the overall radioactivity of reactor components. Proper management and disposal strategies are necessary.
In conclusion, the interplay between sodium isotopes and neutron cross-sections forms a critical aspect of nuclear science and technology. Precise knowledge and careful consideration of these factors are paramount. They are paramount for the safe and efficient operation of nuclear reactors and other applications involving neutron interactions with sodium.
Having established the critical role of sodium isotopes and neutron cross-sections in dictating reaction probabilities, it's logical to ask: where does this crucial research actually take place? Which facilities are at the forefront of unraveling the complexities of sodium-neutron interactions? Understanding the landscape of these research hubs provides valuable context for appreciating the advancements in this field.
Frontiers of Research: Facilities Studying Sodium-Neutron Interactions
The study of sodium and neutron interactions isn't confined to theoretical models and computational simulations. Across the globe, specialized research facilities are actively engaged in experimental investigations. These facilities, equipped with advanced instrumentation and resources, are crucial for validating theoretical predictions and pushing the boundaries of our knowledge.
Research Reactors: A Foundation for Neutron Studies
Research reactors are a cornerstone of neutron-based research. They provide a controlled environment for generating and utilizing neutron beams. These beams are essential for studying various materials, including sodium and sodium-containing compounds.
Examples of research reactors include:
-
The High Flux Isotope Reactor (HFIR) at Oak Ridge National Laboratory (ORNL) in the United States.
-
The Institut Laue-Langevin (ILL) in Grenoble, France.
These reactors serve as versatile platforms for conducting neutron scattering experiments. They enable scientists to probe the structural and dynamic properties of sodium under different conditions. They can also study the effects of neutron irradiation on reactor materials.
Spallation Neutron Sources: High-Intensity Neutron Beams
Spallation neutron sources represent another vital class of facilities. These sources generate neutrons by bombarding a heavy metal target (typically tungsten or mercury) with high-energy protons. This process, known as spallation, produces a copious amount of neutrons.
Notable examples of spallation sources include:
-
The Spallation Neutron Source (SNS) at Oak Ridge National Laboratory.
-
The Japan Proton Accelerator Research Complex (J-PARC) in Tokai, Japan.
These facilities are particularly well-suited for studying dynamic processes in materials. They provide intense neutron beams that are essential for time-resolved experiments. These experiments help capture the real-time behavior of sodium under extreme conditions.
Specialized Facilities for Reactor Research
Beyond general-purpose research reactors and spallation sources, some facilities are specifically designed for studying reactor-related phenomena. These facilities often incorporate specialized equipment for handling radioactive materials and simulating reactor environments.
An example is:
- The Experimental Breeder Reactor-II (EBR-II), though now decommissioned, was a pioneering facility for studying sodium-cooled fast reactors.
While EBR-II is no longer operational, its legacy lives on. It has contributed significantly to our understanding of sodium coolant behavior. Also, the effects of irradiation on reactor components are better understood as well. Future facilities build upon the knowledge gained from EBR-II.
Research Focus: Understanding Sodium Behavior
The research conducted at these facilities spans a wide range of topics related to sodium and neutron interactions.
-
Neutron Scattering Studies: Investigating the atomic structure and dynamics of sodium-containing materials using neutron diffraction and spectroscopy.
-
Irradiation Effects: Examining the effects of neutron irradiation on the properties of sodium and reactor materials.
-
Reactor Safety: Studying the behavior of sodium coolant under accident conditions to enhance reactor safety.
-
Advanced Reactor Design: Developing innovative reactor designs that utilize sodium coolant for improved efficiency and safety.
International Collaboration: A Global Endeavor
Research on sodium and neutron interactions is a collaborative endeavor, with scientists from around the world working together to address complex challenges. International collaborations facilitate the sharing of knowledge, resources, and expertise. This accelerates progress in this important field.
The facilities mentioned above represent just a fraction of the global infrastructure dedicated to studying sodium and neutron interactions. These research hubs play a vital role in advancing our understanding of this complex relationship. They contribute to the development of safer, more efficient, and sustainable energy technologies.
Having established the critical role of sodium isotopes and neutron cross-sections in dictating reaction probabilities, it's logical to ask: where does this crucial research actually take place? Which facilities are at the forefront of unraveling the complexities of sodium-neutron interactions? Understanding the landscape of these research hubs provides valuable context for appreciating the advancements in this field.
From Lab to Power Grid: Real-World Applications and Energy Production
The intricate dance between sodium and neutrons, meticulously studied in laboratories worldwide, extends far beyond academic curiosity. These interactions are the bedrock of several critical applications, most notably in energy production and various technological advancements. The principles uncovered through fundamental research directly translate into tangible benefits for society, powering homes and industries alike.
Sodium and Energy: A Powerful Partnership
The primary real-world application of sodium-neutron interactions lies in the realm of nuclear energy, particularly in the design and operation of Sodium-Cooled Fast Reactors (SFRs). These reactors leverage the unique properties of sodium to achieve efficient and sustainable energy generation.
SFRs: A Cornerstone of Nuclear Energy
SFRs represent an advanced type of nuclear reactor with several advantages over traditional light water reactors. Sodium's exceptional thermal conductivity allows for efficient heat removal from the reactor core, enabling higher power densities and improved fuel utilization.
Furthermore, sodium's low neutron absorption cross-section contributes to a harder neutron spectrum within the reactor core. This harder spectrum facilitates the breeding of plutonium, a fissile material, from uranium-238. This breeding process enhances the reactor's fuel efficiency and reduces the overall demand for natural uranium resources.
Electricity Generation: How SFRs Contribute
The heat generated within an SFR core, through controlled nuclear fission reactions, is transferred to a secondary sodium loop. This secondary sodium then heats water to produce steam, which drives turbines connected to electricity generators. The process is similar to conventional power plants, but the heat source is derived from nuclear fission mediated by sodium and neutron interactions.
SFRs contribute to electricity production in several countries, offering a low-carbon alternative to fossil fuels. While SFR technology is more complex and requires careful engineering and safety considerations, the potential benefits in terms of fuel efficiency, waste reduction, and energy security are significant.
Beyond Reactors: Other Applications
While energy production is the most prominent application, sodium-neutron interactions play a role in other technological domains. Neutron activation analysis (NAA), as detailed earlier, relies on neutron bombardment to identify and quantify elements within a sample. This technique is employed in various industries, including:
- Environmental monitoring
- Materials science
- Forensic science
The creation of radioisotopes through neutron irradiation, involving sodium or other target materials, also contributes to medical imaging and cancer therapy. These applications, while not directly related to energy production, demonstrate the versatility of neutron-based technologies stemming from the fundamental understanding of neutron interactions with various elements, including sodium.
Video: Sodium Neutrons: Unveiling the Secrets! 60/60
Sodium Neutrons: Unveiling the Secrets! 60/60 - FAQs
Here are some frequently asked questions to further clarify the properties and implications of Sodium Neutrons.
What does "Sodium Neutrons: 60/60" actually mean?
The term "60/60" refers to a hypothetical isotope of sodium where the nucleus contains 60 protons and 60 neutrons. This is highly unusual because naturally occurring sodium isotopes have significantly fewer neutrons than protons.
Is Sodium-120 (Sodium Neutrons 60/60) stable?
No, Sodium-120 (60 protons, 60 neutrons) would be extremely unstable. The large imbalance between protons and neutrons in the nucleus would cause it to decay rapidly through processes like beta decay or spontaneous fission. Stable isotopes require a specific neutron-to-proton ratio.
How are unusual isotopes like Sodium Neutrons (60/60) created?
Creating such unusual isotopes requires high-energy nuclear reactions, often within particle accelerators or nuclear reactors. Even then, they exist for only fractions of a second before decaying. Scientists study these short-lived isotopes to understand the limits of nuclear stability and the fundamental forces that hold nuclei together.
Why is it important to study isotopes like Sodium Neutrons 60/60, even though they are unstable?
Studying exotic isotopes like hypothetical sodium neutrons helps us test and refine our models of nuclear structure. By understanding how nuclei behave at extreme neutron-to-proton ratios, we gain insights into the strong nuclear force, which is crucial for understanding the origin of elements in the universe.
So, hopefully, that gives you a solid grasp of sodium neutrons! Keep exploring, and who knows what other fascinating discoveries await us in the world of nuclear physics?