Sodium Electrons: What Are They & Why They Matter?
Sodium, a vital element, exhibits unique chemical properties due to its electron configuration. Ionic bonding, a concept central to understanding chemical reactions, is highly influenced by the behavior of sodium electrons. Understanding sodium chloride's formation, commonly known as table salt, exemplifies the importance of electron transfer involving sodium electrons. Further exploration into the periodic table reveals how the single valence electron in sodium dictates its reactivity.
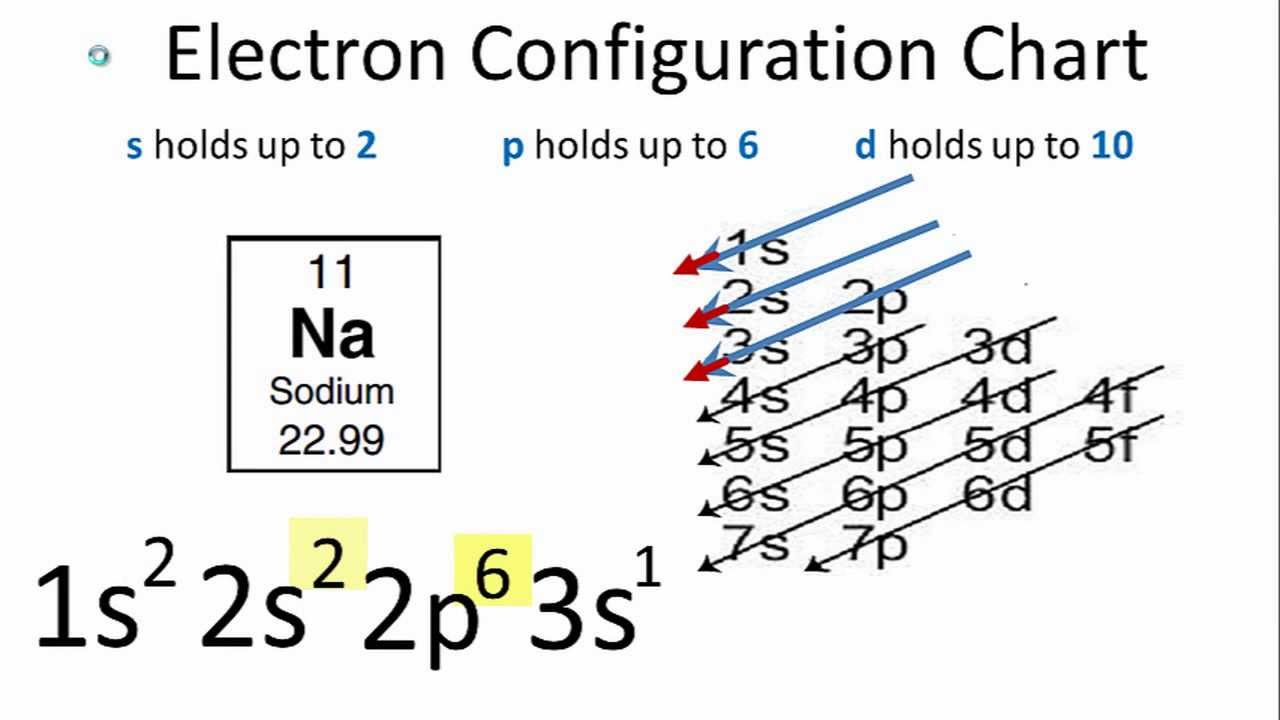
Image taken from the YouTube channel Wayne Breslyn (Dr. B.) , from the video titled Sodium Electron Configuration .
Sodium (Na), an element familiar to many, extends far beyond its simple appearance. From the table salt that flavors our food to its critical functions within our bodies, sodium is ubiquitous and essential.
But what dictates the properties that make sodium so significant? The answer lies within the realm of its electrons, the tiny, negatively charged particles that govern its behavior.
The Ubiquitous Sodium Atom
Sodium's presence is felt across diverse fields. In biology, it plays a vital role in nerve impulse transmission. In industry, it is a key component in the creation of a myriad of useful compounds.
It's an element that deserves a closer look, beyond the common perception of it.
Electrons: The Architects of Sodium's Behavior
The properties that make sodium a cornerstone of chemistry stem from the arrangement of its electrons. These subatomic particles dictate how sodium interacts with other elements.
It dictates how sodium forms chemical bonds, and ultimately, how it contributes to larger molecular structures. Understanding sodium's electrons is the key to unlocking its chemical personality.
Decoding Sodium: An Exploration of Electronic Structure
This article aims to provide a comprehensive explanation of sodium electrons and their significance. We will dissect the electronic structure of sodium.
We will explore its reactivity, and delve into the far-reaching implications of this element in chemistry and beyond. By understanding the fundamentals of sodium's electrons, we gain insight into the fundamental principles governing chemical behavior.
This knowledge allows us to appreciate the role of sodium in our world. We also gain insights into the broader world of chemistry.
Delving into Sodium's Atomic Architecture
We've established that sodium's electrons are the key to its behavior. But to truly understand their influence, we need to first explore the fundamental building blocks of the sodium atom itself.
This requires a journey into the core of matter, understanding how protons, neutrons, and, of course, electrons are arranged within this element.
The Atom: A Foundation of Matter
At its most basic, an atom consists of three primary subatomic particles: protons, neutrons, and electrons.
The protons and neutrons reside in the nucleus, the atom's central core. Protons carry a positive (+) charge, while neutrons have no charge, making them neutral.
Orbiting around the nucleus are the electrons, which carry a negative (-) charge. These electrons are not simply scattered randomly; they are organized into specific energy levels or shells.
Sodium's Unique Electronic Blueprint
Sodium (Na) has an atomic number of 11, meaning it has 11 protons within its nucleus. In a neutral sodium atom, the number of electrons also equals 11 to balance the positive charge of the protons.
These 11 electrons are not all held with the same amount of energy. They reside in specific energy levels or electron shells around the nucleus.
The first shell, closest to the nucleus, can hold a maximum of two electrons. The second shell can hold up to eight electrons. The third shell can hold up to 18 electrons, though sodium only utilizes it partially.
This distribution directly dictates sodium’s chemical behavior.
Unpacking the Electron Configuration
The electron configuration of sodium is expressed as 1s2 2s2 2p6 3s1. This notation might seem complex, but it's a concise way to describe the arrangement of electrons.
-
The numbers (1, 2, 3) represent the energy levels or shells.
-
The letters (s, p) denote the sublevels within each shell, which have distinct shapes and energy.
-
The superscripts (2, 6, 1) indicate the number of electrons occupying each sublevel.
Breaking it down: 1s2 signifies that the first energy level (shell) has two electrons in the s sublevel. 2s2 2p6 indicates that the second energy level has two electrons in the s sublevel and six electrons in the p sublevel. Finally, 3s1 indicates that the third energy level has one electron in the s sublevel.
Valence Electrons: The Key Players
Of all the electrons in an atom, the ones farthest from the nucleus, in the outermost shell, are called valence electrons. These are the electrons primarily involved in chemical bonding.
Sodium possesses one valence electron, residing in its outermost 3s sublevel. This single valence electron is what makes sodium such a reactive element.
It readily participates in chemical reactions to achieve a more stable electron configuration, a principle we'll explore further.
Delving into the atomic structure gives us a static snapshot of sodium. However, the true magic lies in how sodium interacts with other elements. This interaction is governed by its outermost electrons, and understanding their role is key to unlocking the secrets of sodium's behavior.
The Power of Valence Electrons: Shaping Sodium's Behavior
Atoms don't exist in isolation; they form molecules and compounds through chemical bonds. These bonds are the result of interactions between atoms, with valence electrons playing the starring role. These outermost electrons are the key players in determining how an atom interacts with its neighbors, and thus, its chemical properties.
Valence Electrons: The Architects of Chemical Bonds
Valence electrons are not just any electrons; they are the electrons in the outermost shell of an atom. They are the ones that participate in chemical bonding. Sodium, with its electron configuration of 1s2 2s2 2p6 3s1, has just one valence electron in its outermost (3s) shell.
This single electron is loosely held compared to the electrons in the inner shells closer to the positively charged nucleus. This makes it relatively easy to remove, and this ease dictates much of sodium's chemical reactivity.
The interactions between valence electrons determine the type of chemical bond that forms. Atoms can share electrons (covalent bonds) or transfer electrons (ionic bonds). With its lone valence electron, sodium is a prime candidate for forming ionic bonds.
The Octet Rule: Striving for Stability
The octet rule is a guiding principle in understanding chemical bonding. It states that atoms tend to gain, lose, or share electrons to achieve a full outer shell of eight electrons, resembling the electron configuration of noble gases.
Noble gases, such as neon and argon, are exceptionally stable and unreactive due to their full outer shells. Other elements "strive" to achieve this stability through chemical reactions.
Sodium, with its single valence electron, is just one electron away from having a completely empty outer shell (losing its 3s1 electron). Alternatively, it is seven electrons away from achieving a full outer shell of eight electrons.
It is much easier for sodium to lose one electron than to gain seven. This tendency to lose its valence electron defines much of its chemistry.
Sodium: An Alkali Metal Champion
Sodium belongs to a group of elements known as the alkali metals, found in Group 1 of the periodic table. These elements (lithium, sodium, potassium, rubidium, cesium, and francium) share similar chemical properties due to their similar electron configurations—specifically, having one valence electron.
Like other alkali metals, sodium is a soft, silvery-white metal that is highly reactive. They all readily lose their single valence electron to form positive ions with a +1 charge.
This shared characteristic explains why alkali metals react vigorously with water, halogens, and other elements. The ease with which they lose their valence electron makes them potent reducing agents.
High Reactivity: A Consequence of its Electronic Structure
Sodium's high reactivity is a direct consequence of its single valence electron and its position as an alkali metal. It readily gives up its valence electron to form a positive ion, Na+, achieving a stable electron configuration resembling that of neon.
This tendency to lose an electron makes sodium a strong reducing agent, meaning it readily donates electrons to other substances. This electron donation drives many chemical reactions.
For instance, sodium reacts violently with water, producing hydrogen gas and sodium hydroxide. This is because the sodium atom readily gives up its valence electron to form a bond with the hydroxide ion (OH-).
Similarly, sodium reacts vigorously with chlorine gas to form sodium chloride (table salt). The chlorine atom readily accepts sodium's valence electron, resulting in the formation of an ionic bond.
In conclusion, sodium's chemical behavior is profoundly influenced by its valence electrons. The ease with which it loses its single valence electron, driven by the octet rule and its status as an alkali metal, dictates its high reactivity and its tendency to form ionic compounds. Understanding these factors is essential to comprehending the fundamental role of sodium in chemistry and beyond.
Delving into the atomic structure gives us a static snapshot of sodium. However, the true magic lies in how sodium interacts with other elements. This interaction is governed by its outermost electrons, and understanding their role is key to unlocking the secrets of sodium's behavior.
Sodium in Action: Chemical Reactions and Compound Formation
Sodium's electronic configuration isn't just a theoretical concept; it's the driving force behind its real-world behavior. This section explores how sodium's eagerness to shed its valence electron dictates its interactions with other elements, with a particular focus on the creation of common table salt, sodium chloride (NaCl).
The Eager Reaction with Chlorine: A Tale of Two Ions
Sodium's most iconic reaction is undoubtedly its combination with chlorine. This interaction isn't a gentle dance; it's a highly energetic and exothermic event. When sodium metal comes into contact with chlorine gas, a violent reaction occurs, producing intense heat and bright light.
The product of this spectacular display? A white crystalline solid: sodium chloride, better known as table salt.
This seemingly simple compound holds a profound lesson in chemical bonding.
Ionic Bonding: A Transfer of Power
The formation of sodium chloride exemplifies ionic bonding. Unlike covalent bonds where atoms share electrons, ionic bonds involve a complete transfer of electrons from one atom to another.
In the case of sodium and chlorine, sodium willingly donates its single valence electron to chlorine. Chlorine, with seven valence electrons, is just one electron shy of a full octet. This transfer satisfies chlorine's need for a stable electron configuration.
Achieving Stability: The Driving Force
The driving force behind this electron transfer is the octet rule, which dictates that atoms strive to achieve a stable electron configuration with eight valence electrons (like noble gases).
Sodium, by losing its single valence electron, attains the same electron configuration as neon, a noble gas with a full outer shell.
Chlorine, by gaining that electron, achieves the electron configuration of argon, another noble gas.
From Atoms to Ions: The Birth of Na+ and Cl-
This transfer of an electron has a profound impact on the electrical charge of both atoms. Sodium, having lost a negatively charged electron, becomes a positively charged ion (Na+). This positive charge signifies that it now has one more proton than electrons.
Conversely, chlorine, having gained an electron, becomes a negatively charged ion (Cl-). The negative charge indicates an excess of one electron compared to its number of protons.
These charged atoms are no longer neutral; they are ions. The electrostatic attraction between the positively charged sodium ion (Na+) and the negatively charged chloride ion (Cl-) is what forms the strong ionic bond that holds the NaCl crystal lattice together.
The Significance of Na+: Sodium's Ionization
Sodium's tendency to readily lose its valence electron and form the Na+ ion is a key characteristic of alkali metals. This ionization process is relatively easy, as sodium's ionization energy (the energy required to remove an electron) is comparatively low.
This ease of ionization is what makes sodium such a reactive element, readily participating in a wide range of chemical reactions beyond just the formation of table salt. The resulting Na+ ion plays crucial roles in various biological and industrial processes.
Beyond the Lab: Applications and Implications of Sodium
The dance of electrons within a sodium atom dictates more than just textbook reactions; it underpins critical processes in biology and fuels a diverse range of industrial applications. Sodium's influence extends far beyond the laboratory, weaving its way into the very fabric of life and modern technology. Let's explore some of these real-world roles that highlight the element's pervasive significance.
Sodium's Vital Role in Biological Systems
Sodium ions (Na+) are indispensable for a multitude of biological functions, particularly in nerve impulse transmission. Nerve cells use the movement of sodium and potassium ions across their membranes to generate electrical signals.
This electrochemical gradient allows neurons to rapidly transmit signals throughout the body, enabling everything from muscle movement to thought processes. Without the precise control of sodium ion concentrations, nerve function would be severely compromised.
Maintaining Fluid Balance
Beyond nerve function, sodium plays a critical role in regulating fluid balance within the body. Sodium ions help control the distribution of water between different compartments, such as inside and outside cells. This balance is essential for maintaining blood pressure, preventing dehydration, and ensuring proper cellular function.
The kidneys carefully regulate sodium levels to maintain this delicate equilibrium, illustrating the body's intricate homeostatic mechanisms. Disruptions in sodium balance, whether due to dehydration, dietary imbalances, or kidney dysfunction, can lead to serious health consequences.
Industrial Applications of Sodium Compounds
Sodium compounds are workhorses in the industrial sector, serving as key ingredients in the manufacturing of a vast array of products. Sodium chloride (NaCl), or common salt, is not only a culinary staple but also a crucial raw material for producing chlorine gas and sodium hydroxide, both of which are essential for making plastics, paper, and other industrial products.
Sodium carbonate (Na2CO3), also known as soda ash, is widely used in the glass industry, as well as in the production of detergents and other chemicals. The versatility of sodium compounds makes them indispensable in numerous industrial processes.
Sodium Metal: Properties and Uses
While sodium compounds are prevalent, sodium metal itself also has notable applications. Sodium's high electrical conductivity makes it a valuable component in some specialized electrical applications.
However, its reactivity with water and air necessitates careful handling and storage. Sodium is also used in the production of certain pharmaceuticals and in specialized chemical reactions.
Video: Sodium Electrons: What Are They & Why They Matter?
Sodium Electrons: FAQs
Hopefully, this FAQ section clarifies some common questions about sodium electrons and their importance.
Where are sodium electrons located within an atom?
Sodium electrons are arranged in specific energy levels or shells around the nucleus of a sodium atom. The outermost shell, also known as the valence shell, holds only one electron. This single valence electron is what makes sodium so reactive.
Why is sodium so reactive because of its electrons?
Sodium is highly reactive due to its single valence electron. It readily loses this electron to achieve a stable electron configuration. This process of losing an electron leads to the formation of a positively charged sodium ion, which then easily bonds with other elements.
What kind of bonds do sodium electrons participate in?
Sodium electrons primarily participate in ionic bonds. When a sodium atom loses its single valence electron to another atom (like chlorine), it forms a positive ion. The other atom gains an electron and becomes a negative ion. The strong electrostatic attraction between these oppositely charged ions forms a stable ionic bond.
Why are sodium electrons important in biology?
Sodium electrons play a crucial role in biological systems, especially in nerve function. Sodium ions, formed when sodium loses its valence electron, are essential for transmitting nerve impulses. The movement of sodium ions across cell membranes creates electrical signals that allow nerves to communicate throughout the body.