Rubidium Electron Configuration: A Simple Guide
Unlocking the secrets of the periodic table can feel like navigating a complex maze, but understanding the electron configuration of elements like rubidium doesn't have to be daunting. Niels Bohr's atomic model laid the groundwork for our understanding of how electrons are arranged around an atom's nucleus, and rubidium, a soft, silvery-white metal, is no exception. The "s", "p", "d", and "f" orbitals, which are essential components in writing the rubidium electron configuration, dictate where electrons reside within the atom. Mastering this concept allows chemists and students alike to predict rubidium's behavior in various chemical reactions, making the study of rubidium electron configuration not only accessible but also fascinating.
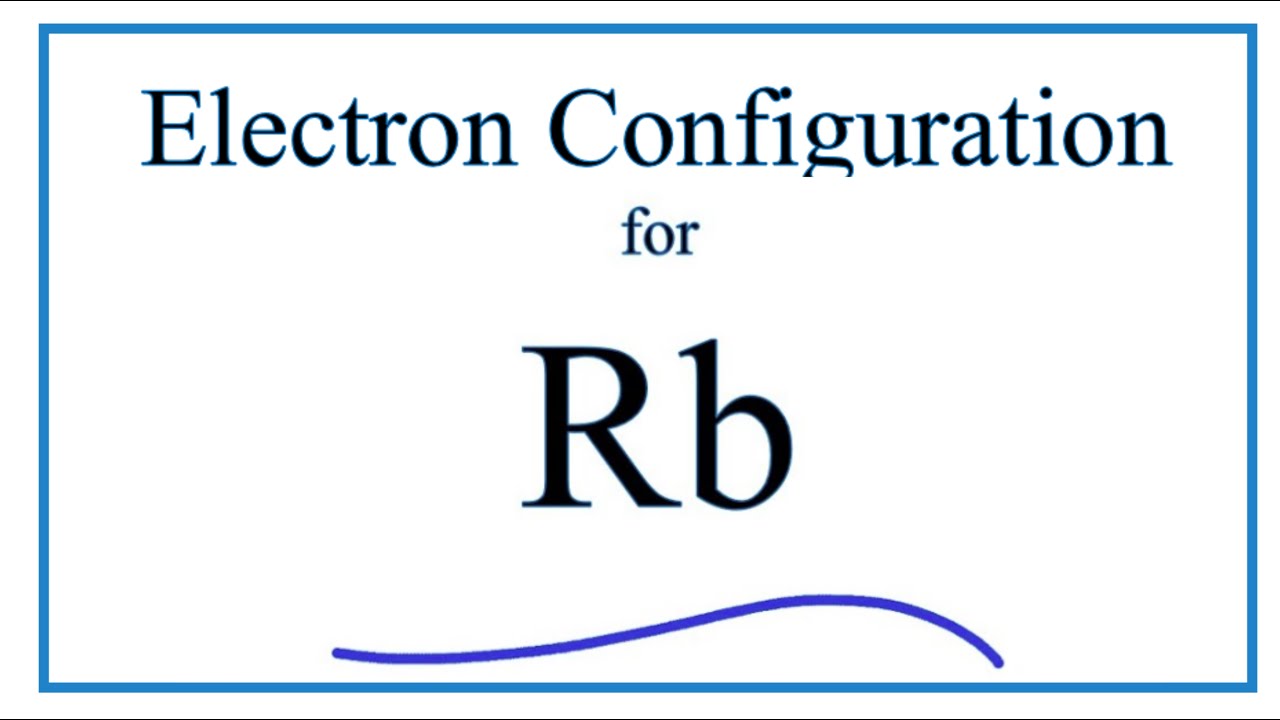
Image taken from the YouTube channel Wayne Breslyn (Dr. B.) , from the video titled A step-by-step description of how to write the electron configuration for Rubidium (Rb). .
Have you ever wondered what dictates the behavior of the elements around us? The answer lies within their electron configuration. Let's start by exploring Rubidium (Rb), a fascinating element that provides a perfect example of this principle.
Rubidium: A Member of the Alkali Metal Family
Rubidium, with the symbol Rb, proudly takes its place as an alkali metal in the periodic table's Group 1. Alkali metals are known for their high reactivity, a characteristic that stems directly from their electron arrangement.
Think of the periodic table as a meticulously organized neighborhood, where elements with similar properties reside near each other. Rubidium's neighbors, like Sodium and Potassium, share this high reactivity due to their similar electronic structures.
The Key: Electron Configuration and Chemical Behavior
So, why is electron configuration so important? It's because the arrangement of electrons determines how an atom interacts with other atoms. These interactions are what drive chemical reactions and define an element's chemical properties.
Understanding electron configuration is like having a roadmap to an element's behavior. It tells us how likely an atom is to form bonds, what types of compounds it can create, and even how it will react with water or air.
Atomic Number 37: The Electron Count
Every element has a unique atomic number, which defines its identity. For Rubidium, this number is 37. This seemingly simple number holds crucial information: it tells us the number of protons within Rubidium's nucleus, and, in a neutral atom, it also tells us the number of electrons orbiting that nucleus.
Therefore, a neutral Rubidium atom contains 37 electrons. It is these 37 electrons that we will be arranging to understand the element's electronic structure. Stay tuned to learn how these electrons are arranged! This arrangement is what dictates Rubidium's unique properties and behavior.
Have you ever wondered what dictates the behavior of the elements around us? The answer lies within their electron configuration. Let's start by exploring Rubidium (Rb), a fascinating element that provides a perfect example of this principle.
The Foundation: Electrons and Atomic Number
Before we dive into the specifics of Rubidium's electron configuration, it's crucial to establish a solid foundation. This section will cover the fundamental concepts of electrons and atomic numbers. By reinforcing these basics, we'll be well-prepared to tackle the complexities ahead.
What is an Electron?
At the heart of every atom lies the nucleus, containing protons and neutrons. Whizzing around the nucleus are tiny, negatively charged particles called electrons.
These aren't just any particles; they are the key players in chemical bonding.
Electrons are responsible for how atoms interact with each other, forming molecules and compounds. Think of them as the glue that holds everything together.
Electrons and Chemical Bonding
When atoms come together to form a chemical bond, it's the electrons that are doing all the work. Atoms share or transfer electrons to achieve a more stable electron configuration.
This sharing or transferring of electrons dictates the type of bond formed, whether it's a strong covalent bond or an ionic bond. Understanding this role of electrons is essential to understanding chemical reactions.
Electron Configuration: Why Does It Matter?
Electron configuration is simply the arrangement of electrons within an atom. It's not just a random arrangement, but a specific pattern governed by the laws of quantum mechanics.
The electron configuration determines an element's chemical properties. It dictates how an atom will interact with other atoms.
Knowing the electron configuration of an element is like having a secret code to its chemical behavior.
Revisiting Rubidium's Atomic Number: 37
As we discussed earlier, Rubidium's atomic number is 37. This number is incredibly important because it tells us two things: the number of protons in Rubidium's nucleus and, in a neutral atom, the number of electrons orbiting that nucleus.
Therefore, a neutral Rubidium atom has 37 electrons. These 37 electrons need to be arranged in a specific way to determine Rubidium's electron configuration. The arrangement governs Rubidium’s behavior.
Understanding that the atomic number directly corresponds to the number of electrons is the cornerstone of understanding electron configurations. With this foundational knowledge, we can proceed to explore the rules and principles that govern electron arrangement.
Have you ever wondered what dictates the behavior of the elements around us? The answer lies within their electron configuration. Let's start by exploring Rubidium (Rb), a fascinating element that provides a perfect example of this principle.
Unlocking the Rules: Orbitals, Quantum Numbers, and the Aufbau Principle
Now that we have a solid understanding of electrons and atomic numbers, let's delve into the rules that govern how electrons arrange themselves around the nucleus. These rules are based on the principles of quantum mechanics and are essential for understanding the electron configuration of any element, including Rubidium.
Atomic Orbitals: Defining Electron Space
Electrons don't orbit the nucleus in neat, planetary-like paths. Instead, they exist in specific regions of space called atomic orbitals. Think of these orbitals as probability maps; they show where an electron is most likely to be found at any given time.
There are four main types of atomic orbitals, designated as s, p, d, and f. Each type has a distinct shape:
- s orbitals: These are spherical, with the nucleus at the center. Each energy level has one s orbital.
- p orbitals: These have a dumbbell shape and exist in sets of three, oriented along the x, y, and z axes.
- d orbitals: These have more complex shapes and exist in sets of five.
- f orbitals: These are even more complex and exist in sets of seven.
The further you move away from the nucleus, the more complex the orbital shapes become.
Quantum Numbers: The Electron's Address
To fully describe an electron in an atom, we use a set of four numbers called quantum numbers. Think of these as the electron's unique address within the atom.
Principal Quantum Number (n)
The principal quantum number, denoted by n, indicates the energy level of the electron. It can be any positive integer (1, 2, 3, and so on). Higher values of n correspond to higher energy levels and greater distances from the nucleus. For example, n=1 would indicate it is the closest to the nucleus.
Angular Momentum or Azimuthal Quantum Number (l)
The angular momentum quantum number, denoted by l, describes the shape of the electron's orbital and has values ranging from 0 to n-1. Each value of l corresponds to a specific type of orbital:
- l = 0 corresponds to an s orbital.
- l = 1 corresponds to a p orbital.
- l = 2 corresponds to a d orbital.
- l = 3 corresponds to an f orbital.
Magnetic Quantum Number (ml)
The magnetic quantum number, denoted by ml, describes the orientation of the orbital in space. It can take on integer values from -l to +l, including 0. For example, if l = 1 (a p orbital), ml can be -1, 0, or +1, representing the three different p orbitals oriented along the x, y, and z axes.
Spin Quantum Number (ms)
The spin quantum number, denoted by ms, describes the intrinsic angular momentum of the electron, which is also quantized. This is often referred to as the "spin" of the electron. It can have two possible values: +1/2 or -1/2, representing the two possible spin states (spin up and spin down).
The Aufbau Principle: Building Electron Configurations
The Aufbau principle (from the German word "Aufbauen" meaning "to build up") provides a systematic way to determine the electron configuration of an atom. It states that electrons first occupy the lowest energy orbitals available before filling higher energy orbitals.
This principle dictates the order in which orbitals are filled: 1s, 2s, 2p, 3s, 3p, 4s, 3d, 4p, 5s, and so on. It's not always a straightforward progression, as some orbitals at higher energy levels (like 4s) can be lower in energy than orbitals at lower energy levels (like 3d). Remember that the orbitals will fill in order of increasing energy!
Understanding the Aufbau principle is critical. The principle is important for predicting the electron configuration of an element and, therefore, its chemical behavior. It's the foundation upon which we build our understanding of how electrons arrange themselves in atoms.
Step-by-Step: Building Rubidium's Electron Configuration
Now that we have a strong grasp of the rules, let's put our knowledge to the test and construct the electron configuration for Rubidium (Rb), step by painstaking step! Grab your metaphorical hard hats; it's time to build.
Laying the Foundation: The Aufbau Principle in Action
Our journey begins with the Aufbau principle, our guiding star in this endeavor. Remember, this principle tells us that electrons will always occupy the lowest energy orbitals first. Think of it like filling a container from the bottom up; you wouldn't start pouring water into the top if the bottom was empty!
To visualize this, keep the filling order in mind: 1s, 2s, 2p, 3s, 3p, 4s, 3d, 4p, 5s… This sequence dictates which orbitals get filled, and in what order.
Filling the Orbitals: A Detailed Walkthrough
Rubidium has an atomic number of 37, meaning a neutral Rubidium atom has 37 electrons. Our task is to distribute these electrons into the appropriate orbitals according to the Aufbau principle.
Stage 1: The Innermost Shells
We begin with the 1s orbital. The 1s orbital can hold a maximum of 2 electrons. So, we fill it completely: 1s².
Next, we move to the second energy level (n=2). The 2s orbital can hold 2 electrons: 2s².
Following that, the 2p orbital comes into play. The 2p orbital can accommodate up to 6 electrons: 2p⁶.
Stage 2: Progressing Outwards
Now, we proceed to the third energy level (n=3). The 3s orbital fills with 2 electrons: 3s².
The 3p orbital follows, housing up to 6 electrons: 3p⁶.
Stage 3: Addressing the 4s and 3d Orbitals
Here's where things get a little interesting! According to the Aufbau principle, the 4s orbital is slightly lower in energy than the 3d orbital. Therefore, we fill the 4s orbital first with 2 electrons: 4s².
Now, we move to the 3d orbital, which can hold a maximum of 10 electrons: 3d¹⁰.
Stage 4: Continuing the Fill
After the 3d orbital, we go to the 4p orbital. This can hold a total of 6 electrons: 4p⁶.
Stage 5: The Final Electron
We've filled quite a few orbitals! Let's tally our electrons: 2 + 2 + 6 + 2 + 6 + 2 + 10 + 6 = 36 electrons. We have one electron left to place.
According to the Aufbau principle, the next orbital in line is the 5s orbital. So, our final electron goes into the 5s orbital: 5s¹.
The Grand Finale: Rubidium's Electron Configuration Revealed
By following these steps meticulously, we've successfully built Rubidium's electron configuration! The result is:
1s²2s²2p⁶3s²3p⁶4s²3d¹⁰4p⁶5s¹
Congratulations! You've just deciphered the electronic structure of Rubidium. Remember, practice makes perfect. So, try applying this step-by-step approach to other elements and watch your confidence soar!
Shorthand: The Noble Gas Configuration for Rubidium
Building upon our understanding of full electron configurations, we can explore a streamlined approach using Noble Gas Notation. This shorthand not only saves time and space, but also highlights the valence electrons, which are crucial for predicting chemical behavior.
What is Noble Gas Notation?
Noble Gas Notation is a simplified way of writing electron configurations. It leverages the stable, filled electron shells of noble gases (Helium, Neon, Argon, Krypton, Xenon, and Radon) to represent the core electrons.
Instead of writing out the entire electron configuration, we use the symbol of the noble gas that precedes the element in question, enclosed in brackets, to represent all the electrons up to that point. We then only write out the remaining electrons in the outermost shells.
Think of it as a shortcut: we are acknowledging that we understand the underlying configuration of the noble gas core electrons and are choosing not to write it all out.
Identifying the Right Noble Gas: Krypton (Kr)
To apply Noble Gas Notation to Rubidium, we need to identify the noble gas that comes before it in the periodic table. Looking at the periodic table, we see that Krypton (Kr), with an atomic number of 36, precedes Rubidium (atomic number 37).
This means that Krypton's electron configuration (1s²2s²2p⁶3s²3p⁶4s²3d¹⁰4p⁶) represents the electron configuration of the first 36 electrons of Rubidium.
Writing Rubidium's Shorthand Configuration: [Kr] 5s¹
Now comes the easy part! We replace the long string of Krypton's electron configuration with its symbol in brackets: [Kr].
Rubidium has one more electron than Krypton. So, after Krypton's configuration is filled, the next electron goes into the 5s orbital. That single electron is represented as 5s¹.
Therefore, the Noble Gas Notation for Rubidium's electron configuration is simply: [Kr] 5s¹. This notation immediately tells us that Rubidium has a Krypton core and one valence electron in the 5s orbital.
By using Noble Gas Notation, we can quickly and efficiently represent electron configurations, focusing our attention on the valence electrons that dictate an element's chemical properties.
Valence Electrons and Chemical Behavior
Now that we've mapped out Rubidium's electron configuration, both in its full and shorthand forms, it's time to understand what it all means. The true power of understanding electron configuration lies in its ability to predict an element's chemical behavior. This is where the concept of valence electrons comes into play.
What are Valence Electrons?
Valence electrons are the electrons in the outermost shell of an atom. These are the electrons that are most involved in chemical bonding and determine how an atom interacts with other atoms.
They are the key players in forming chemical bonds, whether through sharing (covalent bonds) or transferring (ionic bonds) electrons.
Rubidium's Lone Wolf: The 5s¹ Electron
Looking back at Rubidium's Noble Gas Notation, [Kr] 5s¹, we can easily identify its valence electron. It's the single electron residing in the 5s orbital: 5s¹.
This single electron in its outermost shell makes Rubidium an element eager to form chemical bonds. It is characteristic of alkali metals like Rubidium to have only one valence electron.
The Drive to React: Understanding Rubidium's Reactivity
The presence of a single valence electron has profound implications for Rubidium's reactivity. Atoms "prefer" to have a full outermost shell, which confers stability. Rubidium, with its lone 5s¹ electron, is much more likely to lose that electron to achieve a stable electron configuration, resembling the nearest noble gas (Krypton).
This eagerness to lose an electron explains why Rubidium is such a reactive metal. It readily forms positive ions (cations) with a +1 charge.
Ionization Energy: A Measure of Reactivity
The energy required to remove an electron from an atom is called its ionization energy. Elements with low ionization energies readily lose electrons, making them highly reactive.
Rubidium has a relatively low ionization energy. This means that it doesn't take much energy to remove its 5s¹ electron, further explaining its high reactivity.
In essence, Rubidium's eagerness to lose its single valence electron defines its chemical personality: a highly reactive alkali metal that readily forms ionic compounds.
Tools and Resources for Further Exploration
Diving into the world of electron configurations can feel like navigating a complex maze at first. But don't worry! There's a wealth of tools and resources available to guide you and deepen your understanding.
Think of these resources as your trusty compass and map, helping you explore the fascinating landscape of atomic structure. Let's take a look at some of the most valuable assets you can leverage.
The Indispensable Periodic Table
The periodic table is the fundamental resource for any student of chemistry. At a glance, it provides a wealth of information, including:
- Atomic numbers (essential for determining the number of electrons).
- Element symbols.
- Average atomic masses.
- Electron configurations (often abbreviated).
Many periodic tables also display electronegativity values and oxidation states, offering further insights into an element's chemical behavior. Explore interactive periodic tables online, which often include even more detailed data and visualizations.
Unlocking the Power of Online Electron Configuration Calculators
Sometimes, manually determining electron configurations can be tedious, especially for larger atoms. Fortunately, numerous online electron configuration calculators can streamline the process.
These tools allow you to simply enter the element symbol or atomic number and instantly obtain its electron configuration in various formats. These calculators are invaluable for checking your work and for quickly exploring the electron configurations of different elements.
- How to Use Them Effectively: When using these calculators, be sure to double-check the results against the Aufbau principle and Hund's rule (which governs how electrons fill orbitals within a subshell).
- Validating the Results: Comparing the calculator output with your own manual calculations will reinforce your understanding. It also allows for error correction.
Textbooks and Reputable Online Chemistry Resources
Comprehensive chemistry textbooks provide in-depth explanations of electron configurations, quantum mechanics, and atomic structure.
These books typically include detailed diagrams, practice problems, and worked examples. They solidify your understanding. Complement your textbook study with reputable online chemistry resources, such as university websites, Khan Academy, and Chem LibreTexts.
These platforms offer a wealth of articles, videos, and interactive simulations covering electron configurations and related topics.
- Evaluating Credibility: Always evaluate the credibility of online sources by checking the author's credentials and the website's reputation.
- Cross-Referencing Information: Comparing information from multiple sources helps ensure accuracy and provides a more well-rounded perspective.
By leveraging these tools and resources, you can confidently navigate the intricacies of electron configurations and unlock a deeper understanding of the chemical world. Don't hesitate to explore and experiment – the journey of learning is a rewarding one!
Video: Rubidium Electron Configuration: A Simple Guide
FAQs
What is the shorthand notation for rubidium electron configuration, and what does it tell us?
The shorthand notation for rubidium electron configuration is [Kr] 5s1. It means that rubidium has the same electron configuration as krypton (Kr) plus one additional electron in the 5s orbital. This simplifies writing out the full configuration.
Why is understanding rubidium electron configuration important?
Knowing the rubidium electron configuration helps predict its chemical behavior. The single electron in the outermost 5s orbital is easily lost, making rubidium highly reactive and likely to form a +1 ion.
How does rubidium's electron configuration relate to its position on the periodic table?
Rubidium is in Group 1 (alkali metals) and Period 5 of the periodic table. Its [Kr] 5s1 configuration reflects this: Period 5 because the outermost electron is in the 5th energy level, and Group 1 because it has one valence electron.
What's the difference between the full and condensed rubidium electron configuration?
The full rubidium electron configuration (1s2 2s2 2p6 3s2 3p6 4s2 3d10 4p6 5s1) shows every electron. The condensed version, [Kr] 5s1, uses the noble gas krypton (Kr) to represent the inner, filled electron shells, focusing on the valence electron of rubidium.
So, there you have it! Hopefully, this guide has demystified the somewhat intimidating world of electron configurations, especially when it comes to understanding the rubidium electron configuration. Now you can confidently explain how rubidium's electrons are arranged. Happy chemistry-ing!