Rod Shaped Bacteria: Types, Identification, Guide
Rod-shaped bacteria, also known as bacilli, represent a significant group within the diverse world of microbiology and are commonly examined using staining techniques pioneered by scientists like Hans Christian Gram. Bacillus anthracis, a notable species of rod shaped bacteria, exemplifies the pathogenic potential of this bacterial morphology. Accurate identification of rod shaped bacteria often involves utilizing laboratory tools such as microscopes to observe cellular structures and characteristics. The Centers for Disease Control and Prevention (CDC) provides guidelines and resources for identifying and managing infections caused by various types of rod shaped bacteria, underscoring the importance of understanding their characteristics and behaviors.
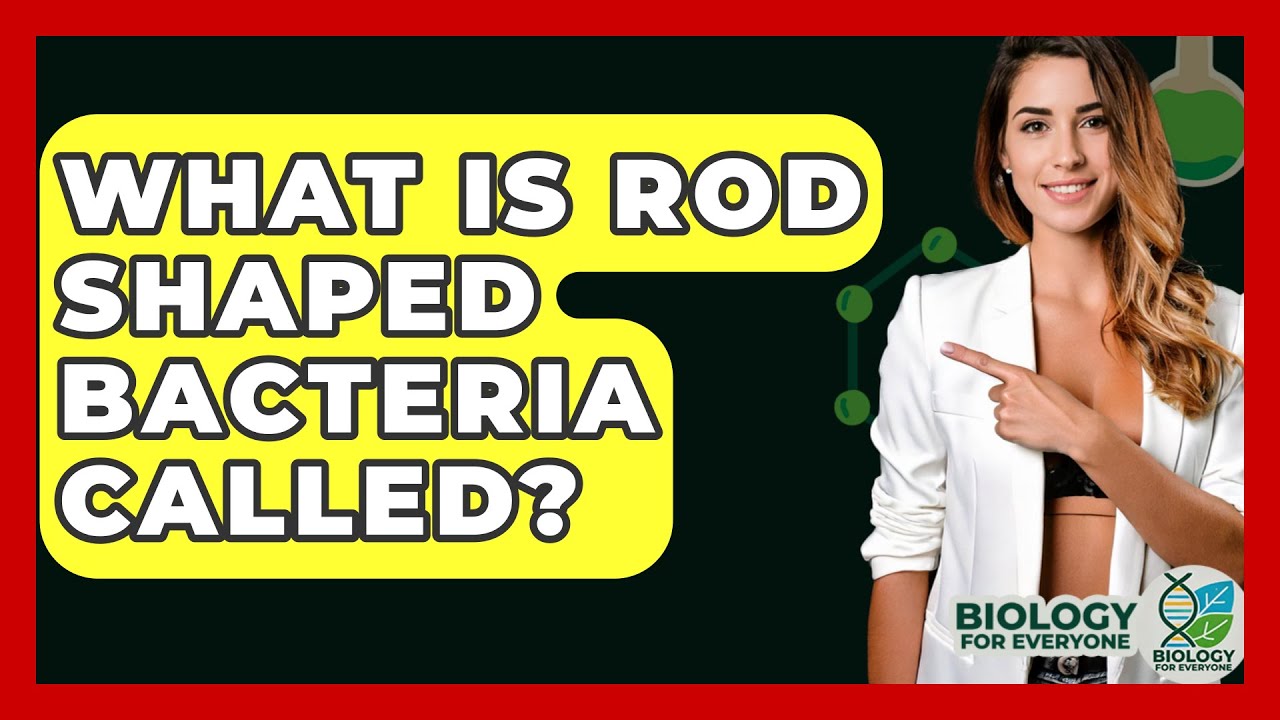
Image taken from the YouTube channel Biology for Everyone , from the video titled What Is Rod Shaped Bacteria Called? - Biology For Everyone .
Unveiling the World of Rod-Shaped Bacteria (Bacilli)
Rod-shaped bacteria, known as bacilli, represent a ubiquitous and remarkably diverse group of microorganisms. Their simple yet effective morphology has allowed them to colonize virtually every environment on Earth.
From the depths of the ocean to the surface of our skin, bacilli play critical roles in a multitude of processes. Understanding their nature and impact is essential for comprehending the intricate web of life and addressing challenges in medicine, industry, and environmental science.
What are Bacilli?
Bacilli are characterized by their elongated, rod-like shape, a defining feature that distinguishes them from other bacterial forms, such as cocci (spherical) or spirilla (spiral). This seemingly simple morphology belies a vast diversity in their genetic makeup, metabolic capabilities, and ecological roles.
Their widespread distribution is a testament to their adaptability. They thrive in diverse conditions, including soil, water, air, and even the extreme environments of hot springs and glaciers. This resilience is often attributed to their ability to form endospores, highly resistant structures that allow them to survive harsh conditions.
Significance Across Domains
The significance of bacilli extends across multiple domains, impacting human health, industrial processes, and ecological balance.
Their roles can be broadly categorized as:
-
Medical Significance: Certain bacilli are notorious pathogens, responsible for diseases like anthrax (caused by Bacillus anthracis) and tetanus (caused by Clostridium tetani). Conversely, some bacilli are beneficial, serving as probiotics that promote gut health and aid in digestion.
-
Industrial Significance: Bacilli are indispensable in various industrial applications. They are used in the fermentation of foods (e.g., Bacillus subtilis in natto production), the production of enzymes (e.g., proteases and amylases used in detergents and food processing), and the synthesis of pharmaceuticals.
-
Ecological Significance: In ecological systems, bacilli are vital for nutrient cycling, decomposition of organic matter, and nitrogen fixation. They play a crucial role in maintaining soil fertility and supporting plant growth.
Bacilli participate in the decomposition of organic matter, breaking down complex compounds into simpler substances that can be recycled by other organisms.
They also contribute to the nitrogen cycle, converting atmospheric nitrogen into forms that plants can use. This is a crucial process for maintaining soil fertility and supporting plant growth.
In conclusion, the world of rod-shaped bacteria is one of immense complexity and importance. Their pervasive nature and diverse activities make them essential players in shaping our planet and influencing our lives.
Morphology and Structure: Deconstructing the Bacillus Form
The success of bacilli hinges on their structural features, which enable them to thrive in diverse environments and execute various functions. From the rigid cell wall to the resilient endospores, each component plays a crucial role in their survival and, in some cases, their pathogenicity.
Understanding these structural elements is key to comprehending the biology of these ubiquitous microorganisms.
Bacillus Morphology and Arrangements
The term "bacillus" inherently refers to a rod-like shape, but within this broad category exists some variation. While most bacilli exhibit a clear rod shape, some may appear as coccobacilli, which are short, oval rods that blur the line between cocci (spherical) and bacilli.
Beyond the individual cell shape, the arrangement of bacilli is another distinguishing characteristic. These arrangements arise from how cells separate after division:
- Single: Individual, unattached rods.
- Pairs (Diplobacilli): Two rods connected end-to-end.
- Chains (Streptobacilli): A linear series of rods connected end-to-end.
- Palisades: Parallel arrangement of rods, often side-by-side, resembling a picket fence.
These morphological differences and arrangements can be valuable clues in the initial identification of a particular bacillus species.
The Bacterial Cell Wall: A Fortress of Peptidoglycan
The cell wall is an essential structure in bacteria, providing rigidity and protecting the cell from osmotic lysis. In bacilli, the cell wall's composition and architecture are critical determinants of their Gram staining properties and their susceptibility to various antimicrobial agents.
Peptidoglycan: The Backbone of the Cell Wall
Peptidoglycan is a unique polymer found only in bacterial cell walls, and it forms the primary structural component. It's a mesh-like structure composed of repeating N-acetylglucosamine (NAG) and N-acetylmuramic acid (NAM) subunits, cross-linked by peptide chains.
This cross-linking provides strength and rigidity, creating a robust barrier that protects the cell from internal pressure and external stressors.
Gram Staining: A Dichotomy of Cell Wall Structures
Gram staining is a fundamental technique in microbiology that differentiates bacteria based on their cell wall structure. The procedure involves staining cells with crystal violet, followed by iodine, decolorization with alcohol, and counterstaining with safranin.
The Gram stain results in two primary classifications: Gram-positive and Gram-negative bacteria.
Gram-Positive Bacteria: Thick Peptidoglycan and Teichoic Acids
Gram-positive bacteria, such as Bacillus and Clostridium species, possess a thick layer of peptidoglycan in their cell wall. This thick layer retains the crystal violet dye during the decolorization step, resulting in a characteristic purple or blue stain.
In addition to peptidoglycan, Gram-positive bacteria often contain teichoic acids, which are negatively charged polymers embedded within the peptidoglycan layer. Teichoic acids contribute to cell wall rigidity, cell division, and binding of cations.
Gram-Negative Bacteria: Thin Peptidoglycan and the Outer Membrane
Gram-negative bacteria, such as Escherichia coli and Salmonella species, have a more complex cell wall structure. They possess a thin layer of peptidoglycan, located between the inner cytoplasmic membrane and an outer membrane.
During Gram staining, the thin peptidoglycan layer is unable to retain the crystal violet dye during decolorization. Consequently, these bacteria are counterstained by safranin, resulting in a pink or red appearance.
The outer membrane is a defining feature of Gram-negative bacteria. It is composed of phospholipids, proteins, and lipopolysaccharide (LPS). LPS, also known as endotoxin, is a potent immunostimulatory molecule that can trigger a strong inflammatory response in the host.
The presence of LPS makes Gram-negative bacteria particularly concerning in clinical settings, as bloodstream infections can lead to septic shock.
Capsule: A Shield Against Phagocytosis
Many bacilli produce a capsule, a layer of polysaccharide or protein that surrounds the cell wall. The capsule serves as a protective barrier, shielding the bacterium from various environmental stressors and host defenses.
One of the primary roles of the capsule is to inhibit phagocytosis, the process by which immune cells engulf and destroy bacteria. By masking surface antigens, the capsule prevents recognition and ingestion by phagocytes, enhancing the bacterium's ability to cause infection.
The composition and structure of the capsule can vary among different bacillus species, influencing its effectiveness as a virulence factor.
Endospores: Dormant Survivalists
A remarkable characteristic of some bacilli, particularly those belonging to the genera Bacillus and Clostridium, is their ability to form endospores. Endospores are highly resistant, dormant structures that allow bacteria to survive extreme environmental conditions.
Formation and Resistance
Endospore formation, or sporulation, is triggered by nutrient deprivation or other stressful conditions. The process involves the replication of the bacterial chromosome, followed by its encapsulation within a tough, multilayered structure.
Endospores exhibit remarkable resistance to:
- Heat: Endospores can withstand boiling temperatures for extended periods.
- Radiation: They are highly resistant to UV and ionizing radiation.
- Desiccation: Endospores can survive in a dehydrated state for years.
- Chemical Disinfectants: They are less susceptible to the effects of many disinfectants.
This resistance is attributed to several factors, including a low water content, a thick cortex composed of peptidoglycan, and the presence of dipicolinic acid (DPA) complexed with calcium ions.
Significance in Survival and Disease
The ability to form endospores allows bacilli to persist in the environment for extended periods, even under conditions that would be lethal to vegetative cells. When favorable conditions return, the endospore can germinate, giving rise to a new, metabolically active bacterial cell.
Endospores also play a critical role in the transmission of certain diseases. For example, Bacillus anthracis endospores can survive in soil for decades and can cause anthrax when inhaled, ingested, or introduced through a skin abrasion. Similarly, Clostridium tetani endospores can enter the body through wounds and cause tetanus.
Motility: Navigating the Environment
Many bacilli are motile, using flagella to move through their environment. Flagella are long, whip-like appendages that rotate to propel the bacterium forward.
Different bacilli exhibit different flagellar arrangements:
- Peritrichous: Flagella are distributed over the entire cell surface.
- Polar: A single flagellum is located at one or both poles of the cell.
Motility allows bacilli to seek out nutrients, escape unfavorable conditions, and colonize new environments. In some pathogenic species, motility may contribute to their ability to invade host tissues.
Metabolism and Growth: Fueling the Bacillus Lifestyle
The survival and proliferation of bacilli depend fundamentally on their metabolic capabilities and growth strategies. These microorganisms exhibit a remarkable diversity in how they acquire energy and reproduce, enabling them to colonize a wide range of environments.
Understanding these metabolic processes and the factors that influence their growth is crucial for comprehending their ecological roles and their impact on human health.
Oxygen Requirements and Metabolic Diversity
Bacilli can be broadly classified based on their oxygen requirements, which dictates their metabolic strategies and the environments they can inhabit.
Aerobes are bacteria that require oxygen for growth, utilizing it as the terminal electron acceptor in aerobic respiration.
Anaerobes, on the other hand, cannot grow in the presence of oxygen; some are even killed by it (obligate anaerobes).
Facultative anaerobes are versatile organisms that can grow with or without oxygen. In the presence of oxygen, they utilize aerobic respiration; in its absence, they switch to fermentation or anaerobic respiration. This adaptability allows them to thrive in diverse niches.
The oxygen requirement of a particular bacillus species has profound implications for its ecological niche. Aerobic bacilli are typically found in oxygen-rich environments, such as the surface layers of soil or in well-aerated water.
Anaerobic bacilli, such as many Clostridium species, are often found in oxygen-depleted environments, such as deep soil layers, sediments, or the gastrointestinal tract.
Energy Production: Respiration
Respiration is a metabolic process that extracts energy from organic compounds by oxidizing them. Aerobic respiration requires oxygen as the final electron acceptor in the electron transport chain, generating a large amount of ATP (adenosine triphosphate), the primary energy currency of the cell.
This process involves the complete oxidation of organic molecules, such as glucose, to carbon dioxide and water. The high energy yield of aerobic respiration allows bacilli to grow rapidly and efficiently in oxygen-rich environments.
Energy Production: Fermentation
In the absence of oxygen, some bacilli utilize fermentation to generate energy.
Fermentation is an anaerobic process that involves the partial oxidation of organic compounds, resulting in the production of various end-products, such as lactic acid, ethanol, acetic acid, and gases.
Fermentation produces much less ATP than aerobic respiration. As such, bacilli that rely on fermentation for energy production typically grow more slowly. However, fermentation allows them to survive and grow in anaerobic environments where respiration is not possible.
The fermentation products generated by bacilli have numerous industrial applications. For example, Bacillus subtilis is used in the fermentation of soybeans to produce natto, a traditional Japanese food.
Other bacilli are used in the production of various organic acids, enzymes, and other valuable compounds.
Reproduction: Binary Fission
Bacilli reproduce primarily through binary fission, a form of asexual reproduction in which a single cell divides into two identical daughter cells.
The process begins with the replication of the bacterial chromosome, followed by cell elongation and the formation of a septum (a dividing wall) in the middle of the cell.
The septum eventually divides the cell into two separate compartments, each containing a complete copy of the chromosome.
Binary fission is a rapid and efficient process, allowing bacterial populations to double in a short period of time under optimal conditions.
Environmental Factors Influencing Growth
The growth and survival of bacilli are significantly influenced by various environmental factors, including:
-
Temperature: Bacilli have optimal temperature ranges for growth, with some species being psychrophiles (cold-loving), mesophiles (moderate-temperature-loving), or thermophiles (heat-loving).
-
pH: Bacilli typically grow best at neutral pH values, but some species can tolerate acidic or alkaline conditions.
-
Nutrient Availability: Bacilli require essential nutrients, such as carbon, nitrogen, phosphorus, and various micronutrients, for growth. The availability of these nutrients can limit their growth in certain environments.
-
Water Activity: Water activity, a measure of the amount of unbound water available for microbial growth, is another critical factor. Bacilli generally require high water activity for growth, but some species can tolerate dry conditions.
Understanding how these environmental factors affect the growth and survival of bacilli is essential for predicting their distribution in nature and for controlling their growth in industrial and clinical settings.
Identification Techniques: Distinguishing One Bacillus from Another
The accurate identification of rod-shaped bacteria, or bacilli, is paramount in various fields, ranging from clinical diagnostics to environmental monitoring and industrial microbiology. Differentiating between various species and strains of bacilli requires a multifaceted approach, employing a range of techniques that leverage their morphological, biochemical, and genetic characteristics. These methods, while varying in complexity and resolution, provide a comprehensive toolkit for bacterial identification.
Microscopic Examination: A First Look
Microscopy forms the initial step in bacterial identification, offering a direct visual assessment of cellular morphology and arrangement. The basic distinctions it offers can guide the next steps.
Light Microscopy
Light microscopy allows for the fundamental observation of bacterial cell shape, size, and arrangement. Staining techniques, such as the Gram stain, can be coupled to further classify bacteria based on cell wall structure. This procedure differentiates bacteria into Gram-positive (retaining the crystal violet stain) and Gram-negative (staining with the counterstain, safranin) groups, due to differences in their cell wall composition. Light microscopy can also reveal the presence of structures like endospores.
Electron Microscopy
Electron microscopy, including Transmission Electron Microscopy (TEM) and Scanning Electron Microscopy (SEM), provides significantly higher magnification and resolution than light microscopy.
This allows for detailed visualization of bacterial ultrastructure, such as cell wall layers, flagellar arrangements, and internal components. While valuable for research purposes and specific diagnostic applications, electron microscopy is less commonly used for routine bacterial identification due to its complexity and cost.
Culture Media: Nurturing Growth
Culture media are essential for isolating and propagating bacteria, allowing for the observation of colony morphology and growth characteristics. The choice of culture medium can significantly influence the outcome of bacterial identification.
Agar Plates and Broth Cultures
Agar plates provide a solid surface for bacterial growth, facilitating the isolation of pure colonies. These colonies can be characterized by their shape, size, color, texture, and edge morphology. Broth cultures, on the other hand, are liquid media used for propagating bacteria in bulk. They are often used for preparing bacterial suspensions for biochemical tests and molecular analyses.
Selective Media
Selective media are designed to inhibit the growth of certain bacteria while allowing others to thrive. This is achieved by incorporating specific inhibitors, such as antibiotics, dyes, or chemicals, into the medium. MacConkey agar, for example, selects for Gram-negative bacteria while inhibiting the growth of Gram-positive organisms.
Differential Media
Differential media contain indicators that allow for the differentiation of bacteria based on their metabolic activities. These indicators often change color in response to pH changes or the production of specific enzymes. Blood agar, for instance, differentiates bacteria based on their ability to lyse red blood cells (hemolysis).
Biochemical Tests: Unveiling Metabolic Fingerprints
Biochemical tests are a cornerstone of bacterial identification, revealing the metabolic capabilities of bacteria by assessing their enzymatic activities and substrate utilization patterns. These tests provide a phenotypic "fingerprint" that can be used to distinguish between different species and strains.
Catalase and Oxidase Tests
The catalase test detects the presence of the catalase enzyme, which catalyzes the decomposition of hydrogen peroxide into water and oxygen. This test is commonly used to differentiate Staphylococcus (catalase-positive) from Streptococcus (catalase-negative). The oxidase test detects the presence of cytochrome c oxidase, an enzyme involved in the electron transport chain. This test is useful for identifying bacteria such as Pseudomonas, which are oxidase-positive.
Fermentation and Urease Tests
Fermentation tests assess the ability of bacteria to ferment specific sugars, such as glucose, lactose, and sucrose. These tests often involve the use of Durham tubes to detect gas production during fermentation. The urease test detects the presence of the urease enzyme, which catalyzes the hydrolysis of urea into ammonia and carbon dioxide. This test is useful for identifying bacteria such as Proteus, which are urease-positive and can cause urinary tract infections.
IMViC Tests
IMViC tests (Indole, Methyl Red, Voges-Proskauer, Citrate) are a suite of biochemical tests commonly used to differentiate Enterobacteriaceae. These tests assess the ability of bacteria to produce indole from tryptophan (Indole test), produce stable acids from glucose fermentation (Methyl Red test), produce acetoin from glucose fermentation (Voges-Proskauer test), and utilize citrate as a sole carbon source (Citrate test).
Molecular Methods: Decoding the Genome
Molecular methods have revolutionized bacterial identification, providing rapid, accurate, and high-resolution tools for differentiating between even closely related species and strains. These methods target specific DNA sequences within the bacterial genome.
PCR and DNA Sequencing
PCR (Polymerase Chain Reaction) amplifies specific DNA sequences, allowing for the detection of even small amounts of bacterial DNA in a sample. PCR can be coupled with other techniques, such as gel electrophoresis or DNA sequencing, for further characterization. DNA sequencing determines the precise nucleotide sequence of a bacterial gene or genome. This information can be used to identify bacteria by comparing their DNA sequences to known sequences in databases.
16S rRNA Sequencing
16S rRNA sequencing has become a gold standard for bacterial identification and phylogenetic analysis. The 16S rRNA gene is a highly conserved gene found in all bacteria, but it also contains variable regions that can be used to differentiate between different species. By sequencing the 16S rRNA gene and comparing it to known sequences, bacteria can be identified with high accuracy.
MALDI-TOF Mass Spectrometry: A Proteomic Approach
MALDI-TOF (Matrix-Assisted Laser Desorption/Ionization Time-of-Flight) mass spectrometry is a rapid and cost-effective method for bacterial identification based on protein profiles. This technique involves ionizing bacterial proteins and measuring their mass-to-charge ratio. The resulting mass spectrum serves as a unique "fingerprint" for each bacterial species, allowing for rapid identification by comparing it to a database of known spectra.
Staining Techniques: Visualizing Key Structures
Staining techniques, beyond the Gram stain, are used to visualize specific bacterial structures, aiding in identification.
Gram Stain and Endospore Stain
The Gram stain kit, as previously mentioned, differentiates bacteria based on cell wall composition. The endospore stain kit, such as the Schaeffer-Fulton stain, is used to identify endospores, which are highly resistant structures produced by certain bacteria, such as Bacillus and Clostridium. This stain differentiates endospores (typically staining green) from vegetative cells (typically staining red or pink), facilitating the identification of endospore-forming bacteria.
Pathogenic Rod-Shaped Bacteria: Enemies Within
Rod-shaped bacteria, while vital in numerous ecological processes and industries, also harbor some notorious pathogens capable of causing severe diseases in humans. Categorizing these bacilli by Gram stain reaction and morphology provides a structured framework for understanding their distinct pathogenic mechanisms and clinical presentations. This section will delve into the characteristics of significant pathogenic rod-shaped bacteria, emphasizing their role as infectious agents.
Gram-Positive Rods: Intracellular and Toxin-Mediated Pathogenesis
Gram-positive rods exhibit a diverse array of pathogenic strategies, often relying on toxin production or intracellular invasion to establish infection. Their thick peptidoglycan layer contributes to their resilience and interaction with the host immune system.
Bacillus: Anthrax and Food Poisoning
The genus Bacillus includes both saprophytic and pathogenic species. Bacillus anthracis, the causative agent of anthrax, exemplifies the severity of bacterial infections. Anthrax manifests in cutaneous, inhalation, and gastrointestinal forms, each with distinct routes of transmission and clinical outcomes. The bacterium's virulence is attributed to its capsule and potent toxins.
Bacillus cereus, another notable species, is a common cause of food poisoning. It produces toxins that lead to emetic or diarrheal syndromes, typically associated with the consumption of contaminated food.
Clostridium: Potent Toxins and Anaerobic Niches
Clostridium species are obligate anaerobes, thriving in oxygen-deprived environments. Several Clostridium species are potent toxin producers, leading to severe and often life-threatening diseases.
Clostridium botulinum produces botulinum toxin, one of the most potent neurotoxins known. It causes botulism, characterized by flaccid paralysis resulting from the toxin's blockage of acetylcholine release at neuromuscular junctions.
Clostridium tetani causes tetanus through the production of tetanospasmin, a neurotoxin that inhibits inhibitory neurotransmitters in the spinal cord. This leads to rigid paralysis, often presenting as lockjaw.
Clostridium perfringens is responsible for gas gangrene, a severe tissue infection characterized by necrosis and gas production. It also causes food poisoning through enterotoxin production.
Clostridium difficile, now Clostridioides difficile, is a major cause of antibiotic-associated diarrhea and pseudomembranous colitis. Disruption of the gut microbiota by antibiotics allows C. difficile to proliferate and produce toxins that damage the colonic mucosa.
Corynebacterium: Diphtheria and Toxin-Mediated Damage
Corynebacterium diphtheriae causes diphtheria, a serious infection primarily affecting the respiratory tract. The bacterium produces diphtheria toxin, which inhibits protein synthesis in eukaryotic cells, leading to tissue damage and the formation of a pseudomembrane in the throat.
Listeria: Intracellular Pathogen and Foodborne Infections
Listeria monocytogenes is a facultative intracellular pathogen, meaning it can survive and multiply within host cells. It causes listeriosis, a severe infection particularly dangerous for pregnant women, newborns, and immunocompromised individuals. Listeria is often transmitted through contaminated food, such as unpasteurized dairy products and processed meats.
Gram-Negative Rods: Endotoxin and Opportunistic Infections
Gram-negative rods possess a thin peptidoglycan layer and an outer membrane containing lipopolysaccharide (LPS), also known as endotoxin. LPS is a potent immunostimulant that can trigger severe systemic inflammation.
Escherichia coli (E. coli): Diverse Pathotypes and Infections
Escherichia coli is a diverse species with both commensal and pathogenic strains. Pathogenic strains of E. coli are categorized into pathotypes based on their virulence factors and mechanisms of pathogenesis. Enterohemorrhagic E. coli (EHEC), such as E. coli O157:H7, produces Shiga toxins that cause bloody diarrhea and hemolytic uremic syndrome (HUS). Enterotoxigenic E. coli (ETEC) produces toxins that lead to watery diarrhea, a common cause of traveler's diarrhea. Enteropathogenic E. coli (EPEC) causes diarrhea primarily in infants by attaching to and effacing intestinal cells.
Salmonella: Gastroenteritis and Systemic Infections
Salmonella species are a common cause of gastroenteritis (salmonellosis), typically acquired through contaminated food or water. Salmonella enterica encompasses various serotypes, some of which cause localized infections in the gut, while others can disseminate systemically. Salmonella typhi is the causative agent of typhoid fever, a severe systemic infection characterized by high fever, abdominal pain, and rose spots.
Pseudomonas: Opportunistic Infections and Biofilm Formation
Pseudomonas aeruginosa is an opportunistic pathogen that primarily infects individuals with compromised immune systems or underlying conditions. It causes a wide range of infections, including pneumonia, bloodstream infections, and wound infections. P. aeruginosa is also known for its ability to form biofilms, which are highly resistant to antibiotics and host defenses.
Curved Rods: Gastric and Intestinal Pathogens
Curved rod-shaped bacteria are a distinctive group that includes important gastrointestinal pathogens. Their unique morphology and motility contribute to their ability to colonize and infect specific niches within the digestive tract.
Helicobacter pylori: Gastritis, Ulcers, and Gastric Cancer
Helicobacter pylori is a spiral-shaped bacterium that colonizes the human stomach. It is a major cause of gastritis, peptic ulcers, and gastric cancer. H. pylori survives in the acidic environment of the stomach by producing urease, an enzyme that neutralizes stomach acid.
Vibrio cholerae: Cholera and Severe Diarrhea
Vibrio cholerae is the causative agent of cholera, a severe diarrheal disease characterized by profuse watery stools. The bacterium produces cholera toxin, which disrupts ion transport in the intestinal cells, leading to massive fluid loss.
Campylobacter jejuni: Campylobacteriosis and Gastroenteritis
Campylobacter jejuni is a common cause of bacterial gastroenteritis (campylobacteriosis) worldwide. It is typically transmitted through contaminated food, such as poultry. Campylobacteriosis is characterized by diarrhea, abdominal pain, and fever.
Antibiotic Susceptibility and Resistance: Battling Bacillus Infections
The effective treatment of infections caused by rod-shaped bacteria hinges critically on understanding their susceptibility and resistance to antimicrobial agents. Antibiotic susceptibility testing provides essential data that guides clinicians in selecting the most appropriate and effective antibiotics, minimizing treatment failures, and mitigating the spread of antibiotic-resistant strains. The increasing prevalence of antibiotic resistance among bacilli necessitates a robust and vigilant approach to antimicrobial stewardship.
The Imperative of Antibiotic Susceptibility Testing
Antibiotic resistance represents a significant threat to global public health. Indiscriminate antibiotic use has driven the evolution of resistant strains, rendering previously effective drugs obsolete.
Determining antibiotic susceptibility patterns for specific bacterial isolates is paramount to ensuring optimal patient outcomes. These tests provide clinicians with crucial information about which antibiotics are likely to be effective against a particular infection, thereby reducing the risk of treatment failure and the emergence of further resistance.
Disk Diffusion Method: A Qualitative Assessment of Susceptibility
The Kirby-Bauer disk diffusion method is a widely used and relatively simple technique for assessing antibiotic susceptibility. This method involves inoculating a standardized bacterial suspension onto an agar plate, placing antibiotic-impregnated disks on the surface, and incubating the plate under controlled conditions.
Following incubation, the zones of inhibition around each disk, representing areas where bacterial growth has been inhibited by the antibiotic, are measured. The size of the zone of inhibition is then compared to established interpretative standards (e.g., CLSI guidelines) to classify the organism as susceptible, intermediate, or resistant to the tested antibiotic.
Advantages and Limitations
The disk diffusion method offers several advantages, including its ease of use, low cost, and ability to test multiple antibiotics simultaneously. However, it is a qualitative test that provides only a general indication of susceptibility.
It does not provide precise quantitative data on the concentration of antibiotic required to inhibit bacterial growth. Furthermore, the accuracy of the disk diffusion method can be influenced by factors such as inoculum density, agar depth, and incubation conditions.
MIC (Minimum Inhibitory Concentration) Testing: A Quantitative Approach
The Minimum Inhibitory Concentration (MIC) is defined as the lowest concentration of an antibiotic that inhibits the visible growth of a bacterium in vitro. Determining the MIC provides a quantitative measure of antibiotic susceptibility, allowing for more precise treatment decisions.
Broth Microdilution: A Common MIC Testing Method
Broth microdilution is a widely used method for determining MICs. This technique involves preparing serial dilutions of an antibiotic in a liquid growth medium and inoculating each dilution with a standardized bacterial suspension.
The microdilution trays are then incubated, and the MIC is determined by visually inspecting the wells for turbidity, which indicates bacterial growth. The lowest concentration of antibiotic that prevents visible growth is recorded as the MIC.
Interpretation and Clinical Significance
The MIC value is compared to established breakpoints, which are specific to each antibiotic and bacterial species. Breakpoints are determined based on pharmacokinetic/pharmacodynamic data and clinical outcomes.
Organisms with MIC values below the susceptible breakpoint are considered susceptible to the antibiotic, while those with MIC values above the resistant breakpoint are considered resistant. The MIC value provides clinicians with valuable information for optimizing antibiotic dosing and selecting the most effective treatment regimen.
By diligently employing antibiotic susceptibility testing methods and interpreting the results within the context of clinical guidelines, healthcare professionals can make informed decisions that optimize patient care and combat the growing threat of antibiotic resistance in rod-shaped bacteria.
Environmental Significance: Bacillus Beyond the Lab
Rod-shaped bacteria, or bacilli, are not confined to laboratory settings or clinical environments. They are ubiquitous, playing critical roles in diverse ecosystems across the globe. From the soil beneath our feet to the depths of aquatic environments and the complex microbial communities within the human and animal gut, bacilli exert a profound influence on nutrient cycling, bioremediation, and a myriad of other ecological processes. Their versatility and adaptability make them indispensable contributors to the balance and functioning of our planet.
Bacilli in the Soil Ecosystem
The soil is a complex and dynamic environment teeming with microbial life. Within this intricate web of interactions, bacilli stand out as key players in several critical processes. They act as efficient decomposers, breaking down complex organic matter into simpler compounds that can be utilized by plants and other organisms.
Many bacilli species also contribute to nitrogen fixation, converting atmospheric nitrogen into ammonia, a form of nitrogen that plants can readily absorb. This process is essential for plant growth and overall soil fertility.
Furthermore, certain bacilli species exhibit plant growth-promoting properties. They can produce hormones and other compounds that stimulate root development, enhance nutrient uptake, and protect plants from pathogens. The use of these bacilli as biofertilizers and biocontrol agents is gaining increasing attention in sustainable agriculture.
Aquatic Environments: Nutrient Cycling and Pollution Degradation
Bacilli are also abundant in aquatic environments, including rivers, lakes, and oceans. In these ecosystems, they play a vital role in nutrient cycling, breaking down organic matter and releasing essential nutrients back into the water column.
Certain bacilli species are capable of degrading pollutants, such as petroleum hydrocarbons, pesticides, and heavy metals. These bacteria can be harnessed for bioremediation, a process that uses microorganisms to clean up contaminated environments.
For example, some bacilli can break down complex hydrocarbons found in oil spills, converting them into less harmful substances. This makes them valuable allies in mitigating the environmental impact of pollution.
The Human and Animal Gut Microbiome
The human and animal gut is home to a vast and diverse community of microorganisms, collectively known as the gut microbiome. Among these microbial inhabitants, bacilli are present and contribute to various aspects of gut health.
Some bacilli species produce enzymes that aid in the digestion of complex carbohydrates and other nutrients. They can also synthesize vitamins, such as vitamin K and certain B vitamins, which are essential for human health.
Furthermore, bacilli can interact with the host's immune system, helping to modulate immune responses and protect against pathogens. Certain bacilli strains are being investigated for their potential as probiotics, which are live microorganisms that confer health benefits when consumed.
Bacilli in Food: Spoilage, Fermentation, and Production
Bacilli have a significant impact on the food industry, playing both detrimental and beneficial roles. Some bacilli species are responsible for food spoilage, causing undesirable changes in taste, texture, and appearance.
However, other bacilli species are essential for food fermentation, a process that transforms raw ingredients into flavorful and nutritious products. Bacillus subtilis, for example, is used in the fermentation of natto, a traditional Japanese food made from soybeans.
Bacilli are also used in the production of various enzymes, such as amylases and proteases, which are used in food processing, detergent manufacturing, and other industrial applications. Their versatility makes them invaluable in biotechnology.
In summary, bacilli are far more than just microscopic organisms; they are vital components of the world around us. Their diverse metabolic capabilities and adaptability enable them to thrive in a wide range of environments, contributing to nutrient cycling, bioremediation, and the health of ecosystems and organisms alike. Understanding the environmental significance of bacilli is crucial for harnessing their potential for sustainable development and addressing environmental challenges.
Impact on Human Health: The Double-Edged Sword of Bacilli
Rod-shaped bacteria, or bacilli, present a complex and often paradoxical relationship with human health. Their influence is not simply confined to causing disease; bacilli also contribute to beneficial processes within the human body. Understanding this duality is crucial for developing effective strategies to combat pathogenic infections and harness the potential of beneficial bacilli for therapeutic purposes.
This section explores the diverse ways in which bacilli impact human health, examining both the detrimental effects of pathogenic species and the positive contributions of those that promote well-being.
The Pathogenic Potential of Bacilli
Numerous rod-shaped bacteria are significant human pathogens, responsible for a wide range of infectious diseases. These infections can range from localized and self-limiting to systemic and life-threatening, depending on the specific pathogen, the host's immune status, and the availability of effective treatment.
Respiratory Infections
Bacterial pneumonia is a prime example of a severe disease caused by several bacilli species. Klebsiella pneumoniae, for instance, is a Gram-negative rod known for causing a particularly aggressive form of pneumonia, often characterized by lung tissue destruction and the formation of abscesses. Similarly, Pseudomonas aeruginosa, another Gram-negative rod, can cause pneumonia, especially in individuals with compromised immune systems or those with chronic lung conditions like cystic fibrosis.
Systemic Infections
Septicemia, or bloodstream infection, represents another critical threat posed by pathogenic bacilli. Escherichia coli and Pseudomonas aeruginosa are frequent culprits in septicemia cases, often originating from infections in other parts of the body, such as the urinary tract or lungs. The presence of these bacteria in the bloodstream can trigger a systemic inflammatory response, leading to sepsis, septic shock, and potentially, multiple organ failure.
Other Infections
Beyond pneumonia and septicemia, various other infections can be attributed to rod-shaped bacteria. These include, but are not limited to, diseases detailed in the "Pathogenic Rod-Shaped Bacteria" section, which covers a spectrum of illnesses caused by Gram-positive and Gram-negative rods, as well as curved rods like Helicobacter pylori and Vibrio cholerae. These infections affect diverse organ systems and present a range of clinical manifestations, underscoring the versatility and adaptability of pathogenic bacilli.
Beneficial Roles: The Probiotic Promise
While some bacilli are notorious pathogens, others play beneficial roles in maintaining human health, particularly within the gut microbiome. Certain species of Bacillus, for example, are increasingly recognized for their probiotic potential. These beneficial bacilli can contribute to gut health in several ways, including:
-
Enhancing digestion: Some Bacillus species produce enzymes that aid in the breakdown of complex carbohydrates and other nutrients, improving digestive efficiency.
-
Modulating the immune system: Beneficial bacilli can interact with the host's immune system, promoting immune tolerance and protecting against pathogenic bacteria.
-
Producing beneficial compounds: Certain Bacillus strains can synthesize vitamins and other beneficial compounds that contribute to overall health.
The use of Bacillus as probiotics is an area of growing interest, with ongoing research exploring their potential in preventing and treating various health conditions, such as diarrhea, inflammatory bowel disease, and even certain allergies. The gut microbiome is a diverse and complex ecosystem, and understanding the specific roles of different Bacillus species is essential for developing targeted probiotic interventions.
In conclusion, bacilli exert a complex and multifaceted influence on human health. While pathogenic species pose significant threats, causing a range of infectious diseases, beneficial bacilli offer promising avenues for promoting gut health and overall well-being. Further research is needed to fully elucidate the mechanisms underlying both the harmful and beneficial effects of rod-shaped bacteria. This knowledge is essential for developing effective strategies to combat infections and harness the therapeutic potential of these ubiquitous microorganisms.
Video: Rod Shaped Bacteria: Types, Identification, Guide
Frequently Asked Questions About Rod Shaped Bacteria
What are the main characteristics used to identify different types of rod shaped bacteria?
Identification of rod shaped bacteria relies on several key characteristics including Gram staining (positive or negative), motility (whether they can move), spore formation (presence or absence), and their metabolic capabilities. These features help differentiate between the many species of rod shaped bacteria.
What are some common diseases caused by rod shaped bacteria?
Rod shaped bacteria are responsible for a wide range of illnesses. Examples include E. coli infections causing food poisoning and urinary tract infections, Bacillus anthracis causing anthrax, and Clostridium tetani leading to tetanus. The specific disease depends on the type of rod shaped bacteria involved.
Why is Gram staining important in identifying rod shaped bacteria?
Gram staining is a crucial first step. It differentiates bacteria based on their cell wall structure, resulting in either Gram-positive (purple) or Gram-negative (pink) staining. This simple test quickly narrows down the possibilities when identifying different types of rod shaped bacteria.
What role do spores play in the survival of certain rod shaped bacteria?
Some rod shaped bacteria, like those in the Bacillus and Clostridium genera, can form spores. Spores are highly resistant structures that allow the bacteria to survive harsh environmental conditions such as heat, radiation, and disinfectants. This survival mechanism is critical for the persistence and spread of these rod shaped bacteria.
So, next time you hear about bacteria, remember those little rod-shaped guys! They're everywhere, playing all sorts of roles, good and bad. Hopefully, this guide has given you a better understanding of the world of rod shaped bacteria and how scientists identify them. Keep exploring, keep learning, and maybe you'll even spot some under a microscope one day!