Differential Reproductive Success: Evolution
Differential reproductive success, a cornerstone concept in evolutionary biology, illuminates how natural selection drives species adaptation. Charles Darwin's observations on finches in the Galapagos Islands, for instance, provided early evidence of how variations in traits lead to unequal contributions to future generations. Natural selection acts as the mechanism through which certain traits, better suited to environmental demands, increase an organism's chances of survival and reproduction, thus directly influencing differential reproductive success. Moreover, the field of population genetics offers the mathematical framework to quantify changes in allele frequencies, demonstrating that differential reproductive success affects the genetic makeup of populations over time.
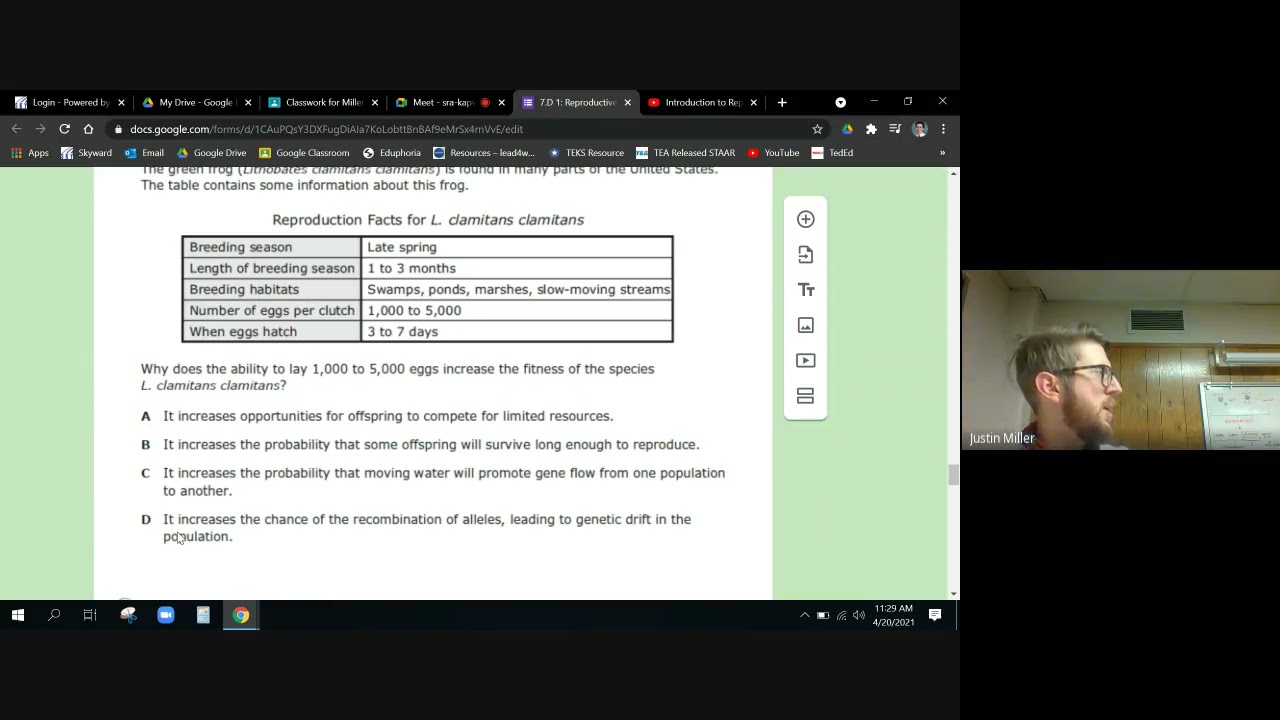
Image taken from the YouTube channel Dr. Professor Miller , from the video titled 7.D 1: Reproductive Success .
The Engine of Evolution: Differential Reproductive Success
Differential reproductive success (DRS) stands as the cornerstone of evolutionary biology, the very engine driving the magnificent diversity of life on Earth. It's a concept both elegant in its simplicity and profound in its implications.
At its heart, DRS refers to the variation in reproductive output among individuals within a population. Some organisms, by virtue of their traits, behaviors, or circumstances, leave behind more offspring than others.
This seemingly straightforward difference is far from inconsequential.
The Consequence: Shifting Allele Frequencies
The primary consequence of differential reproductive success is the alteration of allele frequencies across generations. Alleles, the different forms of a gene, are the raw material of heredity. When individuals carrying certain alleles consistently produce more offspring, those alleles become more prevalent in the gene pool.
Conversely, alleles associated with lower reproductive rates become less common.
This shift in allele frequencies, driven by differential reproductive success, is the very essence of evolution.
Understanding Differential Reproductive Success
This exploration aims to provide a comprehensive understanding of differential reproductive success. We must go beyond a simple definition. Understanding DRS necessitates a thorough analysis of its underlying mechanisms, far-reaching implications, and the broader context within which it operates.
An Overview of Our Approach
Our journey into the heart of differential reproductive success will begin by examining the core concepts that underpin evolutionary advantage. We will explore ideas such as natural selection, adaptation, and fitness.
We will then acknowledge the work of pioneering scientists who have shaped our understanding of this fundamental process. Finally, we will delve into the applications and implications of DRS, demonstrating its relevance across various biological disciplines.
Core Concepts: Building Blocks of Evolutionary Advantage
Differential reproductive success, while a powerful engine in its own right, doesn't operate in a vacuum. Several core concepts act as its essential components, shaping the direction and pace of evolutionary change. Understanding these building blocks is crucial to grasping the full picture of how life adapts and diversifies.
Natural Selection: The Sculptor of Life
Natural selection is arguably the most well-known concept in evolutionary biology. It dictates that traits which confer a higher chance of survival and reproduction become more prevalent in a population over time.
This process hinges on three key components: variation in traits, inheritance of those traits, and, of course, differential reproductive success itself. Individuals with advantageous traits leave behind more offspring, gradually shifting the population's characteristics.
Classic examples abound, such as the rise of antibiotic-resistant bacteria, where only those bacteria survive exposure to the antibiotic and can reproduce. Camouflage adaptation, where organisms evolve to better blend into their environments, is another.
Adaptation: The Result of Selective Pressures
An adaptation is a trait that enhances an organism's ability to survive and reproduce in its environment.
These adaptations can manifest in various forms, including structural features (e.g., the long neck of a giraffe), physiological processes (e.g., the ability of camels to conserve water), and behavioral patterns (e.g., bird migration). Adaptations are not random occurrences; rather, they are the result of natural selection acting on existing variation.
Adaptations have a direct link to differential reproductive success. Organisms with better-suited adaptations are more likely to survive, mate, and produce viable offspring, thus propagating their beneficial traits to future generations.
Fitness: Measuring Reproductive Success
Fitness, in an evolutionary context, refers to an individual's reproductive success relative to others in the population. It's not simply about survival; it's about passing on genes to the next generation.
Fitness encompasses several components: survival (living long enough to reproduce), mating success (acquiring mates), and fecundity (producing a large number of offspring).
The concept of inclusive fitness expands on this idea by considering the reproductive success of an individual's relatives. This explains the evolution of altruistic behaviors, where individuals may sacrifice their own reproductive potential to help close relatives, thus indirectly promoting the survival of their shared genes.
Sexual Selection: The Power of Attraction and Competition
Sexual selection is a specialized form of natural selection where mate choice by one sex or competition within the same sex drives the evolution of certain characteristics.
This can occur through two primary mechanisms: mate choice, where individuals (typically females) prefer mates with specific traits, and intrasexual competition, where individuals (typically males) compete with each other for access to mates.
Traits favored by sexual selection, such as bright plumage in birds or elaborate courtship displays, may not necessarily enhance survival. They do, however, increase mating opportunities, ultimately contributing to differential reproductive success.
Heritability: The Foundation of Inheritance
Heritability quantifies the proportion of phenotypic variation (observable differences) in a population that is attributable to genetic variation.
This concept is critical because selection can only act effectively on traits that are heritable. If a trait is primarily determined by environmental factors, rather than genes, then selection will have little impact on its evolution.
High heritability means offspring will tend to resemble their parents, and that those with traits linked to improved reproduction will pass those traits to their offspring.
Genetic Variation: The Source of Novelty
Genetic variation refers to the differences in genes that exist among individuals within a population.
This variation is the raw material upon which natural selection acts. Without genetic variation, there would be no differences in traits, and therefore no basis for differential reproductive success.
The primary sources of genetic variation include mutation (random changes in DNA), recombination (shuffling of genes during sexual reproduction), and gene flow (migration of genes between populations).
Pioneers of Progress: Shaping the Landscape of Evolutionary Thought
Differential reproductive success, while a powerful engine in its own right, doesn't operate in a vacuum. Several core concepts act as its essential components, shaping the direction and pace of evolutionary change. Understanding these building blocks is crucial to grasping the full picture of how evolution unfolds.
The journey to understanding differential reproductive success is paved with the insights of brilliant minds. From the groundbreaking work of Darwin and Wallace to the modern synthesis and beyond, these individuals have fundamentally shaped our understanding of how life evolves. Their contributions provide the foundation upon which our current knowledge rests.
Darwin and Wallace: The Architects of Natural Selection
Charles Darwin and Alfred Russel Wallace stand as the originators of the theory of evolution by natural selection.
Darwin's meticulous observations and extensive research, culminating in "On the Origin of Species," laid out the core principles: heritable variation exists within populations, and those individuals with traits that confer an advantage are more likely to survive and reproduce.
This differential reproductive success, as we now call it, drives evolutionary change.
Wallace, independently arriving at similar conclusions, spurred Darwin to publish his work and jointly presented the theory to the Linnean Society in 1858.
Their combined insights revolutionized biology, providing a framework for understanding the diversity and adaptation of life on Earth.
The Architects of Modern Synthesis: Fisher, Haldane and Wright
The early 20th century saw the rise of population genetics, reconciling Mendelian inheritance with Darwinian selection. Three figures stand out as key architects of this "modern synthesis": Ronald Fisher, J.B.S. Haldane, and Sewall Wright.
Ronald Fisher, a brilliant statistician, provided the mathematical framework for understanding how natural selection could operate on continuous variation.
His work demonstrated that even small selective advantages could lead to significant evolutionary change over time.
J.B.S. Haldane further developed the mathematical theory of natural selection, exploring its implications for various evolutionary phenomena.
His work helped to clarify the relationship between gene frequencies and selection pressures.
Sewall Wright emphasized the role of genetic drift and population structure in evolution. He introduced the concept of adaptive landscapes, visualizing the relationship between genotype and fitness.
These three scientists laid the groundwork for a comprehensive understanding of how evolution occurs at the population level.
The Modern Synthesis Expanded: Dobzhansky and Mayr
The Modern Synthesis integrated genetics, systematics, and paleontology into a cohesive evolutionary framework. Two prominent figures who significantly shaped this synthesis were Theodosius Dobzhansky and Ernst Mayr.
Dobzhansky's work on genetic diversity in natural populations demonstrated that genetic variation is abundant and provides the raw material for adaptation.
His famous quote, "Nothing in biology makes sense except in the light of evolution," encapsulates the central role of evolution in understanding all aspects of life.
Mayr focused on the process of speciation, emphasizing the importance of reproductive isolation in the formation of new species.
He argued that biological species are defined by their ability to interbreed, and that reproductive barriers are essential for maintaining species boundaries.
The Gene-Centric View: Williams, Hamilton, Trivers, and Dawkins
The latter half of the 20th century saw a shift towards a more gene-centric view of evolution, emphasizing the role of genes as the fundamental units of selection. Key figures in this movement include George C. Williams, William Hamilton, Robert Trivers, and Richard Dawkins.
Williams challenged group selectionist arguments, advocating for the view that adaptation should be explained at the level of the individual or the gene.
Hamilton's theory of kin selection revolutionized our understanding of altruism, showing that individuals can increase their inclusive fitness by helping relatives who share their genes.
Trivers explored the evolution of reciprocal altruism and sexual selection. He made important contributions to our understanding of parent-offspring conflict and the evolution of social behaviors.
Dawkins popularized the gene-centric view in his influential book, "The Selfish Gene."
He argued that genes are the fundamental units of selection and that organisms are simply vehicles for their replication.
Contemporary Voices: Olivia Judson
More recently, evolutionary biologists like Olivia Judson have continued to advance our understanding of differential reproductive success through engaging science communication and research.
Judson's work, including her book "Dr. Tatiana's Sex Advice to All Creation," explores the diverse and often bizarre world of reproductive strategies, highlighting the creative power of natural selection.
Her ability to communicate complex scientific concepts to a broad audience has made a significant impact on public understanding of evolution.
Legacy of Innovation
These pioneering figures, each with their unique contributions, have collectively transformed our understanding of differential reproductive success and the evolutionary process.
Their insights continue to guide research and inspire new discoveries in the ever-evolving field of evolutionary biology. Their work underscores the power of scientific inquiry and the importance of building upon the foundations laid by those who came before.
Applications and Implications: From Genes to Ecosystems
Differential reproductive success, while a powerful engine in its own right, doesn't operate in a vacuum. Several core concepts act as its essential components, shaping the direction and pace of evolutionary change. Understanding these building blocks is crucial to grasping the full implications of DRS across the biological spectrum. This section delves into the practical applications and broader implications of differential reproductive success, spanning population genetics, life history theory, and parental investment theory. Each field provides a unique lens through which we can understand how this fundamental principle shapes the diversity of life.
Population Genetics: The Dance of Alleles
At its most fundamental, differential reproductive success manifests as a shift in allele frequencies within a population. Individuals with traits that enhance survival and reproduction contribute more offspring to the next generation, causing the genes associated with those traits to become more prevalent. This process is the cornerstone of adaptation, driving evolutionary change at the genetic level.
The interplay between selection and genetic variation is key. While selection favors beneficial alleles, the existing pool of genetic diversity provides the raw material upon which it operates. Several factors influence this genetic variation, including mutation, genetic drift, and gene flow.
Factors Affecting Genetic Variation
-
Mutation is the ultimate source of new genetic variants. Although mutations are often deleterious, some can be beneficial or neutral, providing the raw material for adaptation.
-
Selection acts on this variation, favoring alleles that increase fitness and eliminating those that reduce it. However, different forms of selection, such as directional, stabilizing, or disruptive selection, can lead to different evolutionary outcomes.
-
Genetic drift introduces random changes in allele frequencies, particularly in small populations. This can lead to the loss of beneficial alleles or the fixation of deleterious ones, independent of their effect on fitness.
-
Gene flow, the movement of genes between populations, can introduce new alleles and homogenize allele frequencies, counteracting the effects of selection and drift.
Life History Theory: Strategies for Survival and Reproduction
Life history theory examines how organisms allocate their limited resources among growth, reproduction, and survival. The central premise is that natural selection favors life history strategies that maximize lifetime reproductive success. However, resources allocated to one aspect of life history are unavailable for others, leading to trade-offs.
Trade-Offs in Life History Traits
-
Reproduction vs. Survival: Investing heavily in reproduction may decrease an individual's survival probability, and vice versa. For instance, species with high fecundity often have shorter lifespans.
-
Offspring Quantity vs. Quality: Organisms may produce many offspring with low levels of parental care, or fewer offspring with high levels of investment.
-
Early vs. Late Reproduction: Delaying reproduction allows for increased growth and resource acquisition, but it also increases the risk of mortality before reproduction.
The optimal life history strategy will vary depending on environmental conditions, such as resource availability, predation pressure, and competition. These trade-offs shape the evolution of diverse life history strategies observed across the natural world.
Parental Investment Theory: Asymmetry in Reproductive Strategies
Parental investment theory focuses on the differences in reproductive strategies between males and females. In most species, females invest more heavily in offspring, due to the energetic costs of gestation and lactation. This asymmetry in parental investment leads to differences in reproductive strategies.
Factors Influencing Parental Care and Mating Systems
-
Female Choice: Because females invest more in each offspring, they are often more selective in their choice of mates. They may prefer males with traits that indicate good genes or the ability to provide resources.
-
Male-Male Competition: Males often compete with each other for access to females. This competition can lead to the evolution of elaborate displays, weaponry, and territoriality.
-
Mating Systems: The level of parental care influences the mating system of a species. In species where both parents care for offspring, monogamy is more common. In species where females provide most of the care, polygyny (one male mating with multiple females) may be more prevalent.
Parental investment theory highlights how differences in reproductive investment can drive the evolution of diverse mating systems and social behaviors. Understanding these dynamics is crucial for explaining the complexity of animal behavior and social organization.
Video: Differential Reproductive Success: Evolution
FAQs: Differential Reproductive Success & Evolution
What does "differential reproductive success" mean in the context of evolution?
Differential reproductive success simply means that some individuals in a population leave more offspring than others. This difference in reproductive output, often due to advantageous traits, is a primary driver of evolutionary change.
How is differential reproductive success related to natural selection?
Natural selection acts on the variation within a population. Individuals with traits that make them better adapted to their environment are more likely to survive and reproduce, leading to greater differential reproductive success compared to those lacking those advantageous traits.
Why is differential reproductive success important for evolution?
It's crucial because it shifts the genetic makeup of a population over time. Individuals exhibiting higher differential reproductive success pass on their genes more frequently. If these genes encode beneficial traits, they become more common in subsequent generations, ultimately leading to evolutionary adaptation.
Does differential reproductive success always mean the "strongest" survive?
Not necessarily. While physical strength can be an advantage, differential reproductive success encompasses any trait – from camouflage to disease resistance – that increases an individual's ability to survive and reproduce in their specific environment. It's about what works best for a particular niche, not simply about being the strongest.
So, next time you see a peacock strutting its stuff or hear a bird belting out a complex song, remember it's all about the drive to pass on those genes. Ultimately, evolution boils down to differential reproductive success: some variations are just better at getting the job done, ensuring their traits continue to thrive in the gene pool. Pretty wild, right?