What is a Pyrimidine Dimer? Causes & Prevention
Pyrimidine dimers, a type of DNA damage, represent a significant area of study in molecular biology due to their implications for genetic mutations and cellular health. Ultraviolet (UV) radiation serves as the primary cause of pyrimidine dimer formation by inducing covalent bonds between adjacent pyrimidine bases, specifically thymine or cytosine, along a DNA strand. Understanding the molecular mechanisms involved in repairing these lesions, such as nucleotide excision repair (NER), is crucial for developing strategies to mitigate the harmful effects of pyrimidine dimers. The exploration of what is a pyrimidine dimer is further enhanced by the work of researchers at institutions like the National Institutes of Health (NIH), who investigate the cellular responses to DNA damage and develop potential therapeutic interventions.
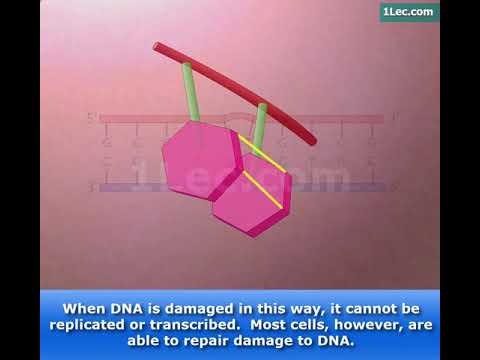
Image taken from the YouTube channel Maxanim Gentaur Ltd. , from the video titled Thymine Dimers .
Unveiling Pyrimidine Dimers: UV Radiation's Mark on DNA
The integrity of our genetic code, DNA, is constantly challenged by various environmental factors. Among these, ultraviolet (UV) radiation stands out as a potent disruptor, primarily through the formation of pyrimidine dimers, a common type of DNA lesion. These dimers represent a significant threat to cellular health, initiating a cascade of events that can lead to mutations and, potentially, cancer.
Pyrimidine Dimers: A UV-Induced Anomaly
Pyrimidine dimers arise when adjacent pyrimidine bases – specifically cytosine (C) and thymine (T) – within the DNA strand become covalently bonded. This aberrant bonding is triggered by the energy absorbed from UV radiation, particularly UV-B.
The process results in the formation of cyclobutane pyrimidine dimers (CPDs) or pyrimidine (6-4) pyrimidone photoproducts (6-4 PPs), distorting the normal structure of the DNA double helix. This distortion is not merely a cosmetic change; it has profound implications for essential cellular processes.
Impairment of DNA Replication and Transcription
The presence of pyrimidine dimers presents a formidable obstacle to DNA replication and transcription.
During replication, the distorted DNA template can stall the progress of DNA polymerase, the enzyme responsible for synthesizing new DNA strands. This stalling can lead to replication errors, including the insertion of incorrect bases or the skipping of segments of the DNA sequence.
Similarly, during transcription, the distortion caused by the dimer can impede the movement of RNA polymerase, the enzyme responsible for synthesizing RNA molecules. This can result in truncated or altered RNA transcripts, disrupting gene expression.
The Link to Mutation and Carcinogenesis
The consequences of unrepaired pyrimidine dimers extend beyond immediate disruptions in replication and transcription. If these lesions persist, they can lead to permanent mutations in the DNA sequence.
These mutations can alter the function of critical genes, including those involved in cell growth and division.
The accumulation of such mutations is a hallmark of cancer development, and pyrimidine dimers have been strongly implicated in the increased risk of skin cancer, particularly melanoma and non-melanoma skin cancers. The connection between UV exposure, pyrimidine dimer formation, and cancer highlights the importance of understanding these DNA lesions and the mechanisms that repair them.
The Molecular Players: Pyrimidine Bases and DNA Distortion
Following the unveiling of pyrimidine dimers and their genesis through UV radiation, it's crucial to understand the molecular entities involved in this disruptive process and how their interactions lead to DNA distortion. These dimers don't just appear out of nowhere; they arise from the fundamental building blocks of our genetic material.
The Pyrimidine Quartet: Cytosine, Thymine, and Uracil
At the heart of DNA and RNA lie the nitrogenous bases, and among them, the pyrimidines—cytosine (C), thymine (T), and uracil (U)—play a starring role in the story of pyrimidine dimer formation. Cytosine and thymine are essential components of DNA, while uracil takes thymine's place in RNA.
These bases are structurally similar, all built around a single-ring molecule. This shared structural foundation, however, doesn't negate their individual significance in the fidelity and functionality of nucleic acids.
Their Roles in Nucleic Acid Architecture
In DNA, cytosine forms a stable base pair with guanine (G), while thymine pairs with adenine (A), creating the rungs of the DNA double helix. This specific pairing, dictated by hydrogen bonding, ensures the accurate transmission of genetic information during replication.
RNA, on the other hand, utilizes uracil to pair with adenine, reflecting its distinct role in protein synthesis and gene regulation. The subtle chemical difference between thymine and uracil—a methyl group—dictates their respective roles in maintaining the stability of DNA versus the flexibility of RNA.
Pyrimidine Dimers and DNA's Structural Integrity
The formation of pyrimidine dimers directly impacts the structural integrity of DNA. When adjacent pyrimidines on the same DNA strand become covalently linked due to UV radiation, the smooth, predictable structure of the DNA double helix is disrupted.
This disruption manifests as a kink or bend in the DNA, altering its overall conformation. The consequences of this distortion are far-reaching, affecting processes essential for cell survival and function.
Impeding Replication and Transcription
The distorted DNA structure caused by pyrimidine dimers poses a significant obstacle to DNA replication and transcription. During replication, the DNA polymerase—the enzyme responsible for synthesizing new DNA strands—struggles to accurately copy the region containing the dimer.
This can lead to replication stalling, errors in the newly synthesized DNA, and potentially, the introduction of mutations. Similarly, during transcription, RNA polymerase encounters difficulty traversing the distorted DNA template.
This can result in premature termination of transcription, reduced gene expression, and ultimately, a compromised cellular function. The cell's ability to accurately replicate and transcribe its genetic material is paramount, and pyrimidine dimers directly undermine this fundamental process. Therefore, understanding the molecular players and their impact is crucial for appreciating the importance of DNA repair mechanisms and protective strategies against UV radiation.
DNA's Defense: Repair Mechanisms Against Pyrimidine Dimers
Following the unveiling of pyrimidine dimers and their genesis through UV radiation, it's crucial to understand the molecular entities involved in this disruptive process and how their interactions lead to DNA distortion. These dimers don't just appear out of nowhere; they arise from the fusion of adjacent pyrimidine bases, causing significant kinks and bends in the DNA's structure. However, all is not lost. DNA, remarkably, possesses a sophisticated arsenal of repair mechanisms designed to combat these UV-induced lesions.
These mechanisms represent the cell's intrinsic ability to maintain the integrity of its genetic code, and understanding them is critical to appreciating the interplay between environmental stressors and cellular resilience.
The Importance of DNA Repair
The constant bombardment of environmental mutagens, including UV radiation, necessitates robust repair systems. Without these mechanisms, the accumulation of DNA damage would lead to catastrophic consequences, including uncontrolled cell growth and the propagation of deleterious mutations. The existence of multiple repair pathways underscores the evolutionary pressure to safeguard the genome.
Nucleotide Excision Repair (NER): A Primary Defense
Nucleotide Excision Repair (NER) stands as a central player in the removal of bulky DNA lesions, with pyrimidine dimers being a prime target. NER operates via a multi-step process, involving the coordinated action of numerous proteins:
- Damage Recognition: NER proteins initially scan the DNA for distortions, identifying the site of the pyrimidine dimer.
- Unwinding: Once identified, the DNA around the lesion is unwound, creating a bubble-like structure.
- Incision: Specialized endonucleases then make incisions on both sides of the damaged region, excising a short stretch of DNA containing the dimer.
- DNA Synthesis and Ligation: Finally, DNA polymerase fills the gap using the undamaged strand as a template, and DNA ligase seals the nick, restoring the DNA's original integrity.
NER is especially crucial in higher organisms, as it can handle a diverse range of DNA adducts, not just pyrimidine dimers.
Photoreactivation: Direct Reversal with Light
Photoreactivation offers a more direct approach to repairing pyrimidine dimers, relying on the enzyme photolyase. This enzyme binds to the dimer and, upon exposure to visible light, utilizes the light energy to break the covalent bonds between the pyrimidine bases, reversing the damage and restoring the DNA to its original state.
Photolyase is prevalent in bacteria, plants, and some animals, but is notably absent in placental mammals, including humans. Its mechanism highlights the power of enzymatic catalysis in reversing potentially harmful DNA modifications.
The Consequences of Unrepaired Dimers
Despite the existence of these repair pathways, some pyrimidine dimers may escape detection and repair. This leads to significant consequences during DNA replication:
- Replication Fork Stalling: Pyrimidine dimers obstruct the progression of the replication fork, as DNA polymerase struggles to accurately copy the damaged template.
- Translesion Synthesis (TLS): To overcome this blockage, specialized DNA polymerases involved in translesion synthesis (TLS) are recruited. These polymerases can bypass the lesion, but they are often error-prone.
- Mutation Introduction: The use of TLS polymerases increases the likelihood of incorporating incorrect nucleotides opposite the dimer, leading to mutations.
These mutations can have far-reaching effects, contributing to genomic instability and increasing the risk of cancer. The delicate balance between DNA damage, repair, and replication fidelity ultimately determines the fate of a cell.
Health Repercussions: Pyrimidine Dimers and the Risk of Skin Cancer
[DNA's Defense: Repair Mechanisms Against Pyrimidine Dimers Following the unveiling of pyrimidine dimers and their genesis through UV radiation, it's crucial to understand the molecular entities involved in this disruptive process and how their interactions lead to DNA distortion. These dimers don't just appear out of nowhere; they arise from the fu...] Now, we must delve into the significant health consequences of these DNA lesions. Specifically, we will explore the undeniable connection between pyrimidine dimers and the development of skin cancer. Understanding this relationship is paramount for effective prevention and early detection strategies.
The Direct Link Between Pyrimidine Dimers and Carcinogenesis
Pyrimidine dimers are notorious instigators of skin cancer.
Their formation, primarily induced by ultraviolet (UV) radiation, directly contributes to the initiation and progression of various forms of skin cancer.
These lesions disrupt the normal DNA replication process, leading to mutations.
If these mutations occur in critical genes involved in cell growth and regulation, the result can be uncontrolled cellular proliferation.
This proliferation forms the basis for cancerous tumors.
Chronic Sun Exposure: A Recipe for Accumulation
Chronic, unprotected exposure to sunlight is the primary driver of pyrimidine dimer accumulation within skin cells.
Each instance of UV radiation exposure inflicts damage upon the DNA.
While our cells possess repair mechanisms, prolonged and excessive exposure can overwhelm these defenses.
This leads to a buildup of unrepaired dimers.
This accumulation significantly elevates the risk of mutations and subsequent carcinogenic transformation.
Individuals who spend long hours outdoors without adequate sun protection face a dramatically heightened risk.
UV-B Radiation: The Primary Culprit
The UV spectrum is broad, but UV-B radiation is particularly implicated in the formation of pyrimidine dimers.
UV-B wavelengths possess sufficient energy to directly damage DNA.
They induce the covalent bonding between adjacent pyrimidine bases.
While UV-A radiation can also contribute to skin damage, its mechanism is typically indirect.
UV-B represents the more potent and immediate threat in terms of dimer formation and associated cancer risk.
Melanoma: A Deadly Consequence
Melanoma, the most aggressive and deadliest form of skin cancer, is strongly linked to pyrimidine dimer formation.
While basal cell carcinoma and squamous cell carcinoma are more common, melanoma's metastatic potential makes it particularly dangerous.
Mutations arising from unrepaired pyrimidine dimers are frequently observed in melanoma cells.
This underscores the crucial role of UV exposure and DNA damage in the pathogenesis of this devastating disease.
Early detection and prevention are paramount in mitigating the risks associated with melanoma and other UV-induced skin cancers.
Genetic Predisposition: Xeroderma Pigmentosum (XP) and DNA Repair Deficiency
Following the unveiling of pyrimidine dimers and their genesis through UV radiation, it's crucial to understand the molecular entities involved in this disruptive process and how their interactions lead to DNA distortion. However, the efficacy of these repair mechanisms isn't uniform across the population. Genetic variations can significantly impair DNA repair pathways, predisposing certain individuals to heightened risks from UV-induced DNA damage.
One stark example of such a genetic predisposition is Xeroderma Pigmentosum (XP), a rare autosomal recessive disorder characterized by a profound deficiency in DNA repair, primarily within the nucleotide excision repair (NER) pathway. This deficiency manifests as an extreme sensitivity to ultraviolet (UV) radiation and a dramatically increased susceptibility to skin cancers.
Understanding Xeroderma Pigmentosum
XP arises from mutations in genes responsible for the NER pathway. NER is the primary mechanism for removing bulky DNA adducts, including pyrimidine dimers. These mutations compromise the cell's ability to efficiently excise and repair UV-induced DNA damage.
The genetic basis of XP is complex, with mutations in at least eight different genes (XPA, XPB, XPC, XPD, XPE, XPF, XPG, and XPV) each potentially leading to the XP phenotype. Each gene encodes a protein involved in a specific step of the NER pathway. Therefore, the severity and specific symptoms of XP can vary depending on which gene is affected and the nature of the mutation.
The Defect in Nucleotide Excision Repair (NER)
The hallmark of XP is a dysfunctional NER pathway. The NER pathway normally functions to recognize distortions in the DNA helix caused by damage, such as pyrimidine dimers. Subsequently, it recruits a complex of proteins to excise the damaged segment, synthesize a new DNA strand using the undamaged strand as a template, and ligate the new strand to restore the integrity of the DNA.
In XP patients, one or more of these steps are impaired. This impairment results in the accumulation of unrepaired DNA damage. This damage leads to mutations and genomic instability, drastically increasing the risk of cancer development. The inability to efficiently repair pyrimidine dimers is a key factor in the development of XP-related pathologies.
Clinical Manifestations and Cancer Risk
Individuals with XP exhibit a range of clinical symptoms, primarily affecting the skin and eyes, due to their heightened sensitivity to UV radiation. Early symptoms often appear in infancy or early childhood, including:
- Severe sunburn after minimal sun exposure.
- Excessive freckling at a young age.
- Dry, scaly skin (xeroderma).
- Pigmentary changes (pigmentosum).
The most alarming consequence of XP is the remarkably elevated risk of developing skin cancers, including basal cell carcinoma, squamous cell carcinoma, and melanoma. These cancers typically appear at a much younger age in XP patients compared to the general population.
Furthermore, XP is associated with an increased risk of internal cancers and neurological abnormalities in some cases, highlighting the systemic impact of impaired DNA repair. Neurological complications can include progressive cognitive impairment, hearing loss, and spasticity.
Management and Prevention Strategies
While there is no cure for XP, meticulous management strategies can significantly improve the quality of life and reduce the risk of cancer development. These strategies primarily focus on rigorous sun protection and regular dermatological surveillance.
-
Strict sun avoidance: Minimizing exposure to sunlight is paramount. This involves staying indoors during peak sunlight hours, wearing protective clothing (long sleeves, hats, sunglasses), and using high-SPF, broad-spectrum sunscreens.
-
Regular dermatological exams: Frequent skin examinations by a dermatologist are crucial for early detection and treatment of any precancerous or cancerous lesions.
-
Surgical intervention: Surgical removal of skin cancers is often necessary, and early intervention is key to improving outcomes.
-
Genetic counseling: Genetic counseling is recommended for families with a history of XP to assess the risk of inheritance and provide information about genetic testing options.
XP serves as a powerful reminder of the critical role of DNA repair in maintaining genomic stability and preventing cancer. The study of XP continues to provide valuable insights into the mechanisms of DNA repair and the consequences of its failure, informing both preventive and therapeutic strategies for cancer and other age-related diseases.
Shielding Against the Sun: Prevention and Protective Measures
Following the unveiling of pyrimidine dimers and their genesis through UV radiation, it's crucial to understand the molecular entities involved in this disruptive process and how their interactions lead to DNA distortion. However, the efficacy of these repair mechanisms is not absolute, nor is it instantaneous. Thus, proactive measures to minimize UV exposure are paramount in preventing the initial formation of these damaging lesions.
Minimizing UV Exposure: A Multifaceted Approach
The cornerstone of pyrimidine dimer prevention lies in diligently reducing the skin's exposure to ultraviolet radiation. This involves a combination of behavioral adjustments and the consistent use of protective barriers. Sun avoidance, particularly during peak hours (typically 10 AM to 4 PM), is a critical first step.
Seeking Shade: A Simple Yet Effective Strategy
When outdoor activities are unavoidable, seeking refuge in shaded areas significantly reduces UV exposure. Trees, umbrellas, and built structures offer substantial protection. Remember that UV radiation can still reach you indirectly, through reflection from surfaces like water and sand, so shade alone is not always sufficient.
Sunscreen: A Chemical Shield Against UV-B
Sunscreen is an indispensable tool in the fight against UV-induced DNA damage. The Sun Protection Factor (SPF) indicates the level of protection against UV-B radiation, the primary culprit in pyrimidine dimer formation.
Understanding SPF and Proper Application
It is vital to choose a sunscreen with an SPF of 30 or higher, offering broad-spectrum protection against both UV-A and UV-B rays. Application should be generous and thorough, covering all exposed skin areas.
Reapplication: Maintaining Optimal Protection
Sunscreen's effectiveness diminishes over time, especially with sweating or swimming. Reapplication every two hours, or immediately after water exposure, is crucial for maintaining optimal protection.
Protective Clothing: A Physical Barrier
Clothing provides a physical barrier against UV radiation. Tightly woven fabrics offer the best protection. Long sleeves, long pants, and wide-brimmed hats can shield significant portions of the skin.
Specialized UV-Protective Clothing
Consider investing in clothing specifically designed with UV-protective properties. These garments are often labeled with an Ultraviolet Protection Factor (UPF), indicating the level of UV radiation they block.
By integrating these preventive measures into daily life, individuals can significantly reduce their risk of pyrimidine dimer formation and, consequently, lower their chances of developing skin cancer and other UV-related health issues. Sun safety is not just a seasonal concern; it is a year-round commitment to preserving the integrity of our DNA.
Pioneers and Progress: Research and Study in the Field of Pyrimidine Dimers
Following the unveiling of pyrimidine dimers and their genesis through UV radiation, it's crucial to understand the molecular entities involved in this disruptive process and how their interactions lead to DNA distortion. However, the efficacy of these repair mechanisms is not absolute, rendering the need for us to reflect on the work of scientists whose years of research have expanded our scientific and medical understanding of pyrimidine dimers, DNA repair mechanisms, and the consequential development of skin cancer. This section highlights pivotal figures and diverse fields contributing to our current understanding.
The Giants Upon Whose Shoulders We Stand
The field of DNA repair is built upon the foundational work of numerous scientists. To name all would be a task too ambitious for this segment. However, it would be impossible to properly chronicle the history of DNA repair and UV radiation’s influence without mentioning the monumental contributions of figures like Richard B. Setlow.
Setlow's research illuminated the mechanisms of DNA repair, particularly focusing on the role of pyrimidine dimers. His work provided critical insights into how cells respond to and repair DNA damage caused by UV radiation. His experimental methods were revolutionary and have helped expand the ways that future generations of researchers study DNA.
His methodologies and findings have paved the way for subsequent advancements in understanding the genetic and molecular basis of diseases linked to DNA damage. Setlow’s insights helped clarify the link between unrepaired dimers and diseases like Xeroderma Pigmentosum.
A Multidisciplinary Approach: Fields of Inquiry
Understanding the intricacies of pyrimidine dimers and their implications demands a comprehensive approach. Several scientific disciplines converge to unravel the complexities of DNA damage, repair, and the resulting health consequences.
Molecular Biology: Deciphering the Mechanisms
Molecular biology plays a central role in elucidating the molecular mechanisms underlying pyrimidine dimer formation and repair. Researchers in this field delve into the enzymatic processes involved in DNA replication, transcription, and repair pathways.
This discipline helps us understand how enzymes like photolyase and components of the Nucleotide Excision Repair (NER) pathway function at a molecular level. Molecular biology also seeks to reveal the intricate interactions between proteins and DNA during damage recognition and repair.
Genetics: Unraveling the Hereditary Impact
Genetics provides insights into the hereditary aspects of DNA repair deficiencies. Genetic studies have been instrumental in identifying genes associated with DNA repair pathways and understanding how mutations in these genes can lead to increased susceptibility to DNA damage and cancer.
Disorders like Xeroderma Pigmentosum (XP), characterized by a profound sensitivity to UV radiation, are prime examples of how genetic defects in DNA repair mechanisms can have severe health consequences.
Photobiology: Illuminating the Effects of Light
Photobiology focuses on the interaction between light and living organisms. In the context of pyrimidine dimers, photobiologists study how UV radiation induces DNA damage.
This field investigates the mechanisms of photoreactivation, a DNA repair process activated by light, particularly in organisms possessing photolyase enzymes.
Dermatology: Bridging the Gap to Clinical Application
Dermatology plays a crucial role in translating basic research findings into clinical applications for preventing and treating skin cancer. Dermatologists are at the forefront of educating the public about the risks of UV exposure and promoting sun-protective behaviors.
Dermatological research focuses on understanding the pathogenesis of skin cancer at a molecular level and developing targeted therapies to combat the disease. Dermatologists can use their understanding of pyrimidine dimers to better treat skin cancers.
The Symbiotic Relationship
Each of these fields contributes unique perspectives and tools to the study of pyrimidine dimers. Molecular biology provides the foundational knowledge of the molecular players, genetics identifies the hereditary factors, photobiology elucidates the role of light, and dermatology translates these findings into clinical practice. This convergence of disciplines drives progress in understanding, preventing, and treating diseases associated with DNA damage.
Only through the combined efforts of scientists across these fields can we hope to fully understand the consequences of UV damage and develop effective strategies to protect human health.
Video: What is a Pyrimidine Dimer? Causes & Prevention
FAQs: Pyrimidine Dimers
What exactly is a pyrimidine dimer?
A pyrimidine dimer is a type of DNA damage. It occurs when two adjacent pyrimidine bases (thymine or cytosine) on the same DNA strand become covalently bonded together. This distorts the DNA structure, interfering with replication and transcription. In short, what is a pyrimidine dimer is a distortion of the DNA.
How do pyrimidine dimers form?
The primary cause of pyrimidine dimer formation is exposure to ultraviolet (UV) radiation, most commonly from sunlight. UV light causes the pyrimidine bases to react abnormally and fuse together. Other factors can also cause what is a pyrimidine dimer, but these are rare compared to UV exposure.
What are the potential consequences of pyrimidine dimer formation?
If left unrepaired, pyrimidine dimers can lead to mutations in DNA. These mutations can disrupt normal cell function, potentially leading to cell death, genetic disorders, or even cancer, particularly skin cancer. The impact is directly related to what is a pyrimidine dimer's effect of blocking normal DNA replication.
How can pyrimidine dimer formation be prevented?
The most effective way to prevent pyrimidine dimer formation is to limit exposure to UV radiation. This includes wearing protective clothing, seeking shade during peak sunlight hours, and using sunscreen with a high SPF. This prevention can significantly reduce the formation of what is a pyrimidine dimer.
So, next time you're soaking up some sun, remember those pesky pyrimidine dimers! Knowing what a pyrimidine dimer is and how they form can help you make smart choices to protect your skin. A little sunscreen and some shade can go a long way in keeping your DNA happy and healthy!