PP Glass Transition Temperature: A Practical Guide
Polypropylene, a versatile polymer, exhibits a critical thermal property known as the PP glass transition temperature, influencing its behavior in various applications. Understanding this property is essential for engineers at organizations such as the Society of Plastics Engineers (SPE), who design products requiring specific performance characteristics. Differential Scanning Calorimetry (DSC), a common thermal analysis tool, allows for precise determination of the PP glass transition temperature. The values obtained through DSC can further guide the work of materials scientists such as Paul Flory, whose research on polymer properties has been seminal to our understanding of this thermal behavior. Therefore, accurate measurements and appropriate application of PP glass transition temperature values are vital for predicting the performance of polypropylene materials across diverse environmental conditions.
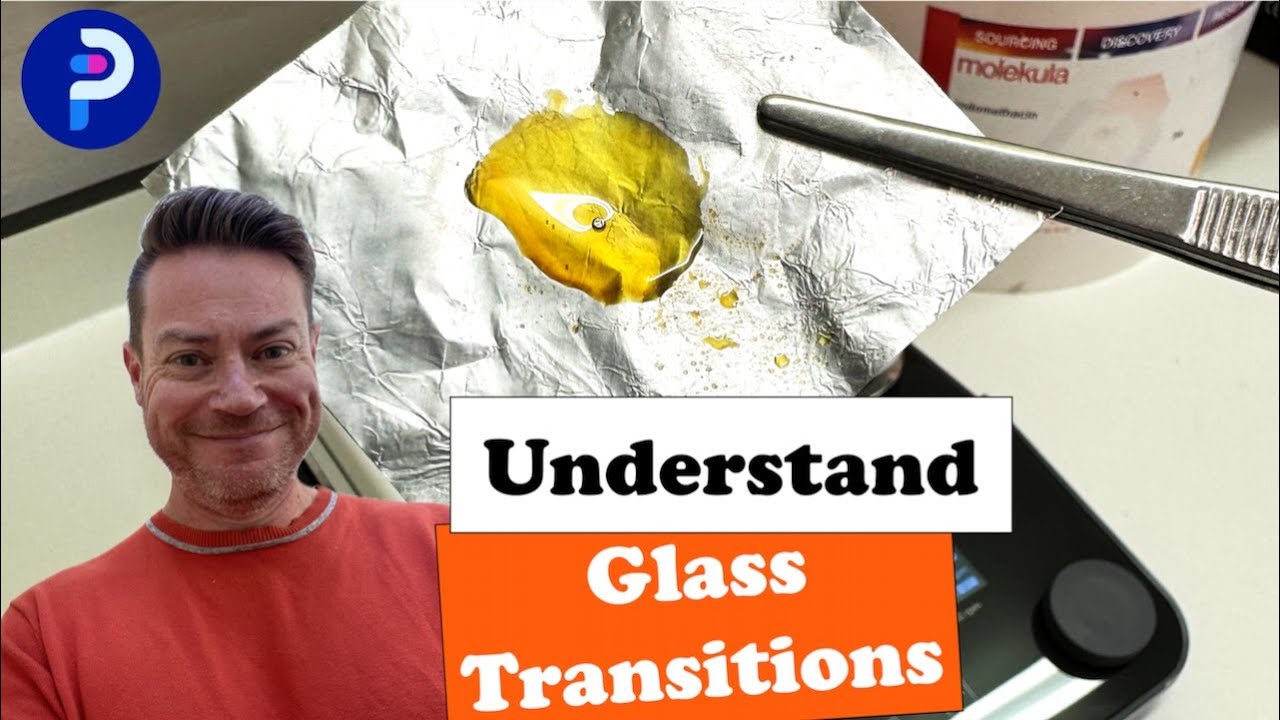
Image taken from the YouTube channel Pharma Drama , from the video titled Unlocking the Mystery of Glass Transition Temperatures .
Understanding the Glass Transition Temperature (Tg) of Polypropylene (PP)
The glass transition temperature (Tg) is a critical property of amorphous and semi-crystalline materials, significantly impacting their behavior and application. It marks the reversible transition from a rigid, glassy state to a more flexible, rubbery state as temperature increases.
This transition is not a phase change in the traditional sense, but rather a change in the material's physical properties due to increased molecular mobility.
Defining the Glass Transition
In amorphous materials, like certain polymers, the glass transition is a distinct and easily observable phenomenon. Below Tg, the material is hard, brittle, and exhibits limited molecular motion.
Above Tg, the material becomes softer, more pliable, and capable of greater deformation.
Semi-crystalline materials, such as polypropylene (PP), present a more complex scenario. They consist of both amorphous and crystalline regions. The crystalline regions remain rigid and maintain structural integrity, while the amorphous regions undergo the glass transition.
The presence of crystallinity influences the overall behavior of the material, affecting the sharpness and detectability of the Tg.
Significance of Tg
The glass transition temperature (Tg) is crucial for several reasons:
-
Material Selection: Tg dictates the temperature range within which a material can be effectively used.
-
Processing: It influences processing parameters such as molding and extrusion temperatures.
-
Performance: It affects the mechanical properties, impact resistance, and long-term durability of the material.
-
Design: An understanding of Tg is crucial in product design to ensure structural integrity and desired performance under varying temperature conditions.
Polypropylene: A Versatile Polymer
Polypropylene (PP) is a widely used thermoplastic polymer known for its versatility, chemical resistance, and cost-effectiveness. Its applications span numerous industries, including:
- Packaging.
- Automotive components.
- Textiles.
- Medical devices.
PP's properties can be tailored through various modifications, such as copolymerization and blending, to meet specific application requirements.
Scope and Objectives
This guide aims to provide a comprehensive understanding of the glass transition temperature of polypropylene. We will explore:
- The fundamental principles behind Tg.
- The factors influencing Tg in PP.
- The techniques used to measure Tg.
- The practical implications of Tg in PP applications.
By the end of this guide, readers will gain insights into controlling Tg to optimize PP performance in diverse applications.
Fundamentals of Glass Transition: From Glassy to Rubbery
Understanding the Glass Transition Temperature (Tg) of Polypropylene (PP) requires a solid grasp of the underlying scientific principles. This section delves into the heart of the glass transition phenomenon. It explores the molecular transformations that dictate the shift from a rigid, glassy state to a more pliable, rubbery state in polymers.
Understanding the Glass Transition Phenomenon
The glass transition is a defining characteristic of amorphous and semi-crystalline polymers. It marks a reversible shift in the material's physical properties. Below the Tg, the polymer exists in a glassy state. It is characterized by hardness, brittleness, and limited molecular mobility. The polymer chains are essentially frozen in place.
As the temperature increases and surpasses the Tg, the polymer enters a rubbery state. In this state, the polymer becomes more flexible and elastic. The polymer chains gain the ability to move more freely. This increased molecular mobility allows for greater deformation under stress.
Molecular Mobility, Chain Conformation, and the Glass Transition
The glass transition is fundamentally linked to the molecular mobility of the polymer chains. In the glassy state, molecular motion is restricted to vibrations and short-range rotations. As temperature rises, the polymer chains acquire sufficient energy to overcome intermolecular forces. This enables larger-scale conformational changes.
These changes lead to increased flexibility and a transition to the rubbery state. The chain conformation also plays a crucial role. Polymers with flexible backbones and fewer bulky side groups generally exhibit lower Tg values.
Key Factors Influencing Tg
Several factors influence the glass transition temperature of a polymer. These include molecular weight, polymer chain flexibility, and free volume. Understanding these factors is crucial for predicting and controlling the thermal behavior of polypropylene and other polymers.
Molecular Weight
Molecular weight plays a significant role in determining the Tg of a polymer. Generally, as the molecular weight increases, the Tg also increases. This is because longer polymer chains exhibit greater entanglement. This entanglement restricts molecular mobility and requires more energy to induce the transition to the rubbery state.
However, this relationship plateaus at very high molecular weights. The effect diminishes as the chains become long enough that further increases do not significantly impact the overall entanglement density.
Polymer Chain Flexibility
The flexibility of the polymer chain backbone also greatly influences Tg. Polymers with inherently flexible backbones, due to the presence of flexible linkages like ether or ester groups, tend to have lower Tg values. This is because these flexible linkages allow for easier rotation and conformational changes, requiring less thermal energy to initiate the glass transition.
Conversely, polymers with stiff backbones, often due to the presence of bulky side groups or rigid cyclic structures, exhibit higher Tg values. These bulky groups hinder chain movement and increase the energy needed for the transition.
Free Volume
Free volume refers to the unoccupied space between polymer chains. This space allows for molecular motion. Polymers with a higher free volume generally exhibit lower Tg values. This is because less energy is required for chains to move and transition to the rubbery state.
Factors that increase free volume, such as the introduction of small molecules or the presence of defects in the polymer structure, can lower the Tg. Conversely, factors that reduce free volume, such as strong intermolecular forces, can increase the Tg.
Amorphous vs. Crystalline Polymers
The presence and degree of crystallinity significantly influence the glass transition behavior of a polymer.
Impact of Crystallinity on Tg
Crystalline polymers possess ordered regions where the polymer chains are tightly packed in a regular lattice. These crystalline regions do not undergo a glass transition. Instead, they exhibit a melting transition (Tm) at a higher temperature.
However, semi-crystalline polymers also contain amorphous regions. These regions do exhibit a glass transition. The Tg of a semi-crystalline polymer is primarily determined by the properties of its amorphous phase. The presence of crystalline regions can constrain the mobility of the amorphous chains, potentially influencing the observed Tg value.
iPP vs. aPP: A Comparison
Isotactic polypropylene (iPP) and atactic polypropylene (aPP) differ significantly in their crystallinity and, consequently, their glass transition behavior. Isotactic PP has a highly ordered structure, allowing for a high degree of crystallinity. Therefore, iPP has a well-defined melting point, and its glass transition, while present in the amorphous regions, is less pronounced.
Atactic PP, on the other hand, has a random arrangement of methyl groups along the polymer chain. This prevents efficient packing and results in a predominantly amorphous structure. As such, atactic PP exhibits a more distinct and easily measurable Tg. It lacks a clear melting point due to the absence of significant crystalline regions. The Tg of aPP is generally lower than that of iPP. This is due to the increased chain mobility in the amorphous structure.
Polypropylene (PP): Characteristics and Glass Transition Behavior
Having explored the foundational concepts of glass transition, we now turn our attention to polypropylene (PP), a polymer whose versatility hinges on understanding its unique thermal properties. This section examines PP's structure, morphology, and how these factors interplay to define its glass transition behavior. The impact of copolymers and blends on PP's Tg will also be considered, offering insights into tailoring PP for specific applications.
PP Homopolymers and Copolymers: A Structural Overview
Polypropylene, in its simplest form, is a homopolymer composed solely of propylene monomers. The arrangement of these monomers, specifically the methyl groups along the polymer chain, dictates the polymer's tacticity and, consequently, its properties. Isotactic PP (iPP), where all methyl groups are on the same side of the chain, exhibits high crystallinity and a relatively high glass transition temperature. In contrast, atactic PP (aPP), with randomly arranged methyl groups, is amorphous and possesses a significantly lower Tg, often below room temperature.
PP copolymers, on the other hand, incorporate other monomers alongside propylene. Common comonomers include ethylene, resulting in random copolymers (PP-R) or block copolymers (PP-B). The introduction of comonomers disrupts the regularity of the PP chain, reducing crystallinity and affecting the glass transition behavior.
Influence of Polymer Morphology on Tg
The morphology of PP, referring to the arrangement of crystalline and amorphous regions within the material, profoundly influences its Tg. Highly crystalline PP exhibits a less pronounced glass transition because the rigid crystalline regions constrain the mobility of the amorphous chains.
The degree of crystallinity, the size and distribution of crystallites, and the presence of any mesophases all contribute to the observed Tg. Factors such as cooling rate during processing can dramatically affect PP's morphology and, therefore, its Tg.
Polypropylene Copolymers and Blends: Tailoring Tg for Specific Needs
The creation of PP copolymers and blends offers a powerful strategy for tailoring the glass transition temperature and overall properties of the material.
The Effect of Comonomers
The inclusion of comonomers in PP chains disrupts the polymer's regularity, leading to a reduction in crystallinity. This, in turn, lowers the glass transition temperature, making the material more flexible at lower temperatures. For instance, incorporating ethylene into PP to form a random copolymer results in a material with improved impact resistance, especially at low temperatures, due to the reduced Tg.
The Impact of Blending
Blending PP with other polymers can also modify its Tg. The miscibility of the blend components plays a crucial role. If the polymers are miscible (mix at the molecular level), the resulting blend will exhibit a single, composition-dependent Tg.
However, if the polymers are immiscible, the blend will display two distinct glass transition temperatures, corresponding to the individual components. A common example is blending PP with elastomers like EPDM (ethylene propylene diene monomer rubber). This blend creates impact-modified PP, where the dispersed elastomeric phase lowers the overall Tg and improves low-temperature toughness.
Material Properties and Applications
The manipulation of PP's Tg through copolymerization and blending directly impacts its material properties and expands its application range. Lowering the Tg enhances flexibility, impact resistance, and low-temperature performance, making it suitable for applications such as automotive parts, flexible packaging, and consumer goods.
Conversely, maintaining a higher Tg preserves stiffness and heat resistance, crucial for applications like rigid containers, fibers, and high-temperature components. By carefully selecting comonomers and blend components, engineers can fine-tune PP's Tg to meet the specific demands of a wide array of applications.
Measuring Tg in Polypropylene: Techniques and Interpretation
Having explored the foundational concepts of glass transition, we now turn our attention to polypropylene (PP), a polymer whose versatility hinges on understanding its unique thermal properties. This section examines the experimental techniques employed to measure the glass transition temperature of polypropylene, focusing on the principles, procedures, and interpretation of results derived from Differential Scanning Calorimetry (DSC) and Dynamic Mechanical Analysis (DMA). We will also briefly touch upon other relevant techniques.
Differential Scanning Calorimetry (DSC)
Differential Scanning Calorimetry (DSC) is a powerful thermal analysis technique used to determine the glass transition temperature (Tg) of polypropylene and other polymeric materials. DSC measures the heat flow difference between a sample and a reference material as a function of temperature.
Principles of DSC
DSC operates by heating both a sample and a reference material under controlled conditions. The instrument maintains both the sample and reference at essentially the same temperature throughout the experiment. If the sample undergoes a thermal transition (like the glass transition), it will absorb or release heat.
The DSC measures the differential heat flow required to maintain the sample and reference at the same temperature. This heat flow difference is directly related to the thermal events occurring in the sample.
Procedure for Determining Tg in PP using DSC
To determine Tg in PP using DSC, a small sample of the material (typically a few milligrams) is placed in a DSC pan. The sample and a reference pan (usually empty) are then heated or cooled at a controlled rate.
A typical DSC experiment involves a heating-cooling-heating cycle. This removes the thermal history of the sample. During the second heating cycle, the glass transition is observed as a change in heat capacity.
Interpreting DSC Thermograms
The glass transition is typically observed as a step-like change in the DSC curve (thermogram). The Tg is often taken as the midpoint of this transition. The sharpness and magnitude of the step can provide information about the amorphous content and homogeneity of the PP sample.
Factors such as heating rate can influence the measured Tg. Therefore, it's crucial to report the experimental conditions along with the Tg value.
Dynamic Mechanical Analysis (DMA)
Dynamic Mechanical Analysis (DMA) provides complementary information to DSC, measuring the viscoelastic properties of polypropylene as a function of temperature or frequency. DMA is particularly sensitive to molecular motions and relaxation processes associated with the glass transition.
Principles of DMA
DMA applies an oscillating force or displacement to a sample and measures the material's response. This response is characterized by two parameters: the storage modulus (E' or G') and the loss modulus (E'' or G'').
The storage modulus represents the elastic component of the material's behavior, while the loss modulus represents the viscous component. The ratio of the loss modulus to the storage modulus is known as the loss tangent (tan δ).
Using DMA to Measure Tg and Viscoelasticity in PP
In a DMA experiment to determine Tg, a PP sample is subjected to an oscillating force at a fixed frequency. The temperature is scanned, and the storage modulus, loss modulus, and tan δ are measured as functions of temperature.
The glass transition is typically identified as a drop in the storage modulus and a peak in the loss modulus and tan δ.
Frequency Dependence of Tg in DMA Measurements
The Tg measured by DMA is frequency-dependent. This is because the molecular motions associated with the glass transition require a certain amount of time to occur.
At higher frequencies, the molecules have less time to respond to the applied force, and the glass transition appears to shift to higher temperatures. This frequency dependence provides valuable information about the relaxation behavior of the polymer.
Other Techniques
While DSC and DMA are the most commonly used techniques for measuring Tg, other methods can also provide useful information.
Dilatometry
Dilatometry measures the change in volume of a material as a function of temperature. At the glass transition, the thermal expansion coefficient typically changes, allowing for the determination of Tg. This method is based on measuring specific volume changes and relating them to the glass transition.
Dielectric Analysis (DEA)
Dielectric Analysis (DEA) probes the electrical properties of a material as a function of temperature or frequency. DEA assesses relaxation processes by measuring the dielectric constant and loss factor. The glass transition can be detected as a change in these properties due to increased molecular mobility.
Factors Affecting the Glass Transition Temperature of Polypropylene
Having explored the techniques used to measure the glass transition temperature (Tg) of polypropylene (PP), we now delve into the factors that can significantly influence this crucial property. The Tg of PP is not a fixed value; it varies based on compositional elements, the rigors of processing, and the surrounding environment. Understanding these factors is paramount for tailoring PP's performance to specific applications.
Compositional Effects on Tg
The addition of fillers, additives, and plasticizers can drastically alter the glass transition temperature of polypropylene. These compositional modifications impact the polymer's chain mobility and intermolecular forces, resulting in shifts in Tg.
Influence of Fillers and Additives
The inclusion of fillers, such as talc, calcium carbonate, or glass fibers, generally increases the Tg of polypropylene. This is primarily due to the restriction of polymer chain mobility imposed by the rigid filler particles. Fillers reduce the free volume available for polymer chain movement, thereby requiring more energy (higher temperature) for the transition from the glassy to the rubbery state.
Additives, on the other hand, can have varying effects depending on their nature. Nucleating agents, for instance, may increase crystallinity, which indirectly influences Tg by affecting the amorphous phase content. Stabilizers and antioxidants usually have a minimal direct impact on Tg, as they are primarily designed to prevent degradation rather than alter the polymer's physical state.
Plasticization and the Reduction of Tg
Plasticizers are substances added to polymers to increase their flexibility and processability. They achieve this by lowering the glass transition temperature. Plasticizers work by inserting themselves between polymer chains, increasing the intermolecular spacing and reducing the van der Waals forces that hold the chains together.
This increased free volume allows the polymer chains to move more freely at lower temperatures, thus reducing the Tg. The effectiveness of a plasticizer depends on its compatibility with the polymer and its ability to disrupt intermolecular interactions.
Common plasticizers for polypropylene include low molecular weight polyolefins or esters. The specific choice of plasticizer and its concentration must be carefully considered to achieve the desired balance of properties without compromising the polymer's strength or thermal stability.
Processing Conditions and Thermal History
The conditions under which polypropylene is processed can also significantly affect its glass transition temperature. Factors such as cooling rate, molding temperature, and annealing processes can alter the polymer's morphology and, consequently, its Tg.
Impact of Thermal History
The thermal history of polypropylene refers to the sequence of heating and cooling cycles it experiences during processing. Rapid cooling from the melt typically results in a lower degree of crystallinity and a lower Tg. This is because the polymer chains have less time to arrange themselves into ordered crystalline structures.
Conversely, slow cooling or annealing (heating the polymer to a temperature below its melting point and holding it there for a period of time) promotes the formation of larger and more perfect crystals, leading to a higher degree of crystallinity and potentially a higher Tg. The specific effects of thermal history are also influenced by the polymer's molecular weight distribution and the presence of nucleating agents.
Environmental Factors and Chemical Exposure
The surrounding environment can also influence the glass transition temperature of polypropylene. Moisture and chemical exposure can alter the polymer's structure and properties, leading to changes in Tg.
Effects of Moisture and Chemical Exposure
Polypropylene is generally considered hydrophobic, meaning it does not readily absorb water. However, prolonged exposure to high humidity or direct contact with water can lead to minimal moisture absorption, which may slightly plasticize the polymer and lower the Tg. The effect is typically less pronounced than with more hydrophilic polymers like polyamides.
Exposure to certain chemicals, particularly solvents, can have a more significant impact. Solvents can swell the polymer matrix, increasing the free volume and reducing the Tg. The extent of the effect depends on the chemical's solubility parameter and its compatibility with polypropylene. Strong oxidizing agents or UV radiation can also degrade the polymer chains, leading to changes in molecular weight and, consequently, the Tg.
Practical Implications: How Tg Impacts Polypropylene Applications
Having explored the techniques used to measure the glass transition temperature (Tg) of polypropylene (PP), we now delve into the factors that can significantly influence this crucial property. The Tg of PP is not a fixed value; it varies based on compositional elements, the rigors of processing, and even ambient environmental conditions. This section unpacks the multifaceted effects of Tg on PP's real-world performance, from processing to end-use applications and even its recyclability.
Processing Temperatures and Melt Flow
The glass transition temperature of PP plays a vital role in determining optimal processing conditions. During processes like injection molding or extrusion, PP must be heated to a molten state to facilitate flow and mold filling.
If the processing temperature is too low, the PP will not melt sufficiently, leading to incomplete filling, poor surface finish, and compromised mechanical properties.
Conversely, excessively high temperatures can cause polymer degradation, resulting in discoloration, reduced molecular weight, and inferior performance.
Therefore, understanding and controlling the Tg is crucial to maintain desired processing parameters. Tailoring the Tg through copolymerization or blending can fine-tune the melt flow characteristics. It ensures efficient processing without sacrificing material integrity.
Service Temperatures and Material Integrity
The service temperature, or the range of temperatures at which a product is expected to perform reliably, is directly influenced by the Tg of the PP material.
When PP is used in applications where it is exposed to varying temperatures, it's critical that the operating temperature remains within the material's capabilities.
At temperatures significantly below the Tg, PP becomes brittle and prone to fracture under stress. Above Tg, its stiffness and strength decrease. This can lead to deformation or failure under load.
For example, PP containers used for hot-filled products must be engineered to withstand elevated temperatures without losing structural integrity.
Product Design and Material Selection
Product designers must consider the Tg of PP when selecting it for specific applications. The intended use-case dictates the ideal Tg range.
For rigid applications, a PP with a higher Tg may be preferred, while flexible applications may benefit from a lower Tg.
Factors such as anticipated loads, exposure to chemicals, and long-term environmental conditions need to be evaluated in relation to the material's Tg.
Copolymers or PP blends can be formulated to achieve a balance of properties tailored to the specific demands of the product, ensuring it meets performance and safety requirements.
Durability, Performance, and Longevity
The long-term durability and performance of PP products are intimately linked to their glass transition temperature. The Tg affects the material's resistance to creep, impact, and fatigue.
Materials operating close to their Tg may exhibit increased creep or reduced impact strength over time.
Environmental factors like UV exposure, humidity, and chemical contact can also influence the long-term behavior of PP near its Tg, potentially leading to premature degradation or failure.
Understanding these interdependencies is critical for designing products with a predictable lifespan.
Recycling and Reprocessing Considerations
Recycling PP presents unique challenges related to its thermal properties, particularly the Tg. During reprocessing, recycled PP is often subjected to multiple melt cycles.
These cycles can alter its molecular weight, crystallinity, and, consequently, its Tg.
Changes in Tg can affect the processability and end-use performance of recycled PP. It may require the addition of virgin material or compatibilizers to restore its desired properties.
A thorough understanding of how recycling impacts Tg is crucial for optimizing reprocessing parameters and maintaining the quality of recycled PP products.
Standards and Regulations for Polypropylene Testing
Having explored the techniques used to measure the glass transition temperature (Tg) of polypropylene (PP), we now turn to the standards and regulations that govern how PP is tested and characterized. Adherence to these standards ensures consistency, reliability, and comparability of results across different laboratories and applications. This section provides an overview of the prominent international standards from ASTM and ISO, focusing on those relevant to determining PP's thermal properties.
Overview of ASTM Standards for Polypropylene
ASTM International, a globally recognized standards organization, offers a wide range of test methods applicable to polypropylene. These standards are crucial for ensuring the quality and performance of PP materials in various applications. Several ASTM standards directly relate to the thermal analysis of PP, including the determination of its glass transition temperature.
Key ASTM Standards for Thermal Analysis of PP
ASTM D3418 stands out as a key standard, encompassing the determination of transition temperatures of polymers using Differential Scanning Calorimetry (DSC). This test method provides a standardized procedure for measuring the Tg of PP, ensuring consistent and comparable results. It specifies the necessary equipment, calibration procedures, and data analysis methods for accurately determining the glass transition temperature.
ASTM E1356 outlines test methods for determining glass transition temperatures by thermomechanical analysis.
ASTM E1640 is used to determine the glass transition temperature, as well as degree of cure, of thermosetting polymers.
Significance of Adhering to ASTM Standards
Adhering to ASTM standards is vital for several reasons. Primarily, it ensures that testing procedures are uniform and well-defined. This uniformity allows for the direct comparison of data obtained from different sources. It also enhances the reliability of results, as these standards are developed and refined by experts in the field. Furthermore, compliance with ASTM standards often forms a basis for contractual agreements and regulatory compliance, adding a layer of assurance and accountability.
Overview of ISO Standards for Polypropylene
The International Organization for Standardization (ISO) provides another set of globally recognized standards for polymer characterization. These standards address various aspects of PP testing, including methods for determining thermal properties such as the glass transition temperature. Compliance with ISO standards demonstrates a commitment to internationally recognized best practices and ensures the quality and consistency of PP materials.
Key ISO Standards for Thermal Analysis of PP
ISO 11357 is a multi-part standard focusing on the DSC testing of polymers. Parts 1, 2, and 3 are particularly relevant, covering general principles, determination of glass transition temperatures, and determination of melting temperatures, respectively. This standard provides detailed guidelines for performing DSC measurements on PP, ensuring accuracy and reproducibility.
ISO 6721 outlines the test methods for determining the dynamic mechanical properties of plastics, including measurement of the glass transition temperature via DMA.
Importance of ISO Compliance
ISO standards provide a framework for ensuring that PP materials meet specific performance requirements. Compliance with these standards facilitates international trade by establishing a common language for material properties and testing procedures. It also provides assurance to end-users that the materials have been tested according to rigorous, internationally recognized methods. This adherence contributes to product safety, reliability, and overall customer satisfaction.
Harmonization Between ASTM and ISO Standards
While ASTM and ISO are distinct organizations, efforts have been made to harmonize standards where possible. This harmonization aims to reduce redundancy and facilitate global commerce by minimizing discrepancies between testing procedures. In some cases, ASTM and ISO standards may be technically equivalent, meaning they produce comparable results. However, it is essential to verify the specific requirements of each standard to ensure compliance with the relevant regulatory or contractual obligations.
Regulatory Considerations
In addition to ASTM and ISO standards, various regulations may apply to the use of PP in specific applications, such as food packaging or medical devices. These regulations often reference or incorporate ASTM and ISO standards to ensure that materials meet safety and performance requirements. Compliance with these regulations is mandatory and may require additional testing and certification.
Video: PP Glass Transition Temperature: A Practical Guide
FAQs: PP Glass Transition Temperature: A Practical Guide
What exactly is the glass transition temperature (Tg) of polypropylene (PP)?
The glass transition temperature (Tg) of polypropylene (PP) is the temperature range where it transitions from a hard, glassy state to a more rubbery or flexible state. It's not a sharp melting point, but rather a gradual change in properties. Understanding the pp glass transition temperature is key to predicting its behavior in different environments.
Why does PP's glass transition temperature matter in practical applications?
Knowing the pp glass transition temperature helps predict how PP will perform at different temperatures. Below its Tg, PP is brittle and more prone to cracking. Above it, PP becomes more flexible. This is critical for selecting the right PP grade and designing products that won't fail due to temperature changes.
How does the PP glass transition temperature relate to its use in packaging?
The pp glass transition temperature is important for packaging because it affects the material's impact resistance and ability to withstand temperature fluctuations during shipping and storage. For example, cold temperatures below its Tg can make PP packaging more susceptible to damage.
Can the glass transition temperature of polypropylene be modified?
Yes, the pp glass transition temperature can be influenced by factors such as the type of PP (homopolymer vs. copolymer) and the addition of fillers or plasticizers. Copolymers, in particular, can exhibit a lower Tg compared to homopolymers, enhancing flexibility at lower temperatures.
So, there you have it – a crash course on PP glass transition temperature. While it might sound a bit technical, understanding this property can really help you make better decisions when working with polypropylene. Hopefully, this guide gave you a clearer picture of what PP glass transition temperature is all about and how to apply that knowledge in the real world. Good luck with your projects!