Are Plantae Prokaryotic or Eukaryotic? Plant Cell
Plantae, a kingdom of multicellular organisms, are characterized by cells containing membrane-bound organelles, a feature that distinguishes them from prokaryotes such as E. coli. The presence of a nucleus within plant cells, similar to that found in Animalia, definitively categorizes the members of the Plantae kingdom as eukaryotic; therefore, the answer to "are plantae prokaryotic or eukaryotic" is that they are not prokaryotic. Scientists at institutions like the Max Planck Institute have conducted extensive research confirming that plant cells possess complex internal structures indicative of eukaryotic organisms.
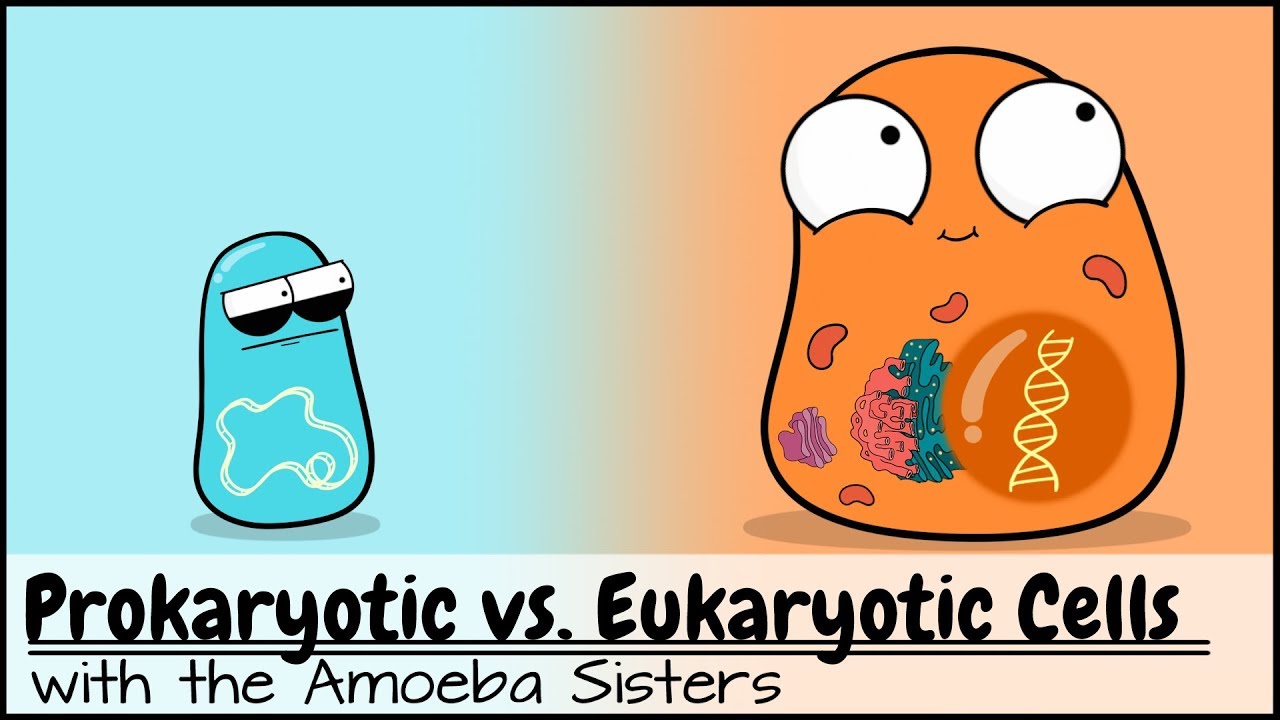
Image taken from the YouTube channel Amoeba Sisters , from the video titled Prokaryotic vs. Eukaryotic Cells (Updated) .
Understanding plant cell biology is fundamental to grasping the complexities of plant life. Plants, as the primary producers in most ecosystems, play a critical role in sustaining life on Earth. Their unique cellular structures and processes are essential for photosynthesis, growth, and adaptation to diverse environments.
This section lays the groundwork for exploring the fascinating world of plant cells. We will define key terms and concepts, setting the stage for a detailed examination of their unique characteristics. Understanding these basics is crucial for appreciating the intricate mechanisms that drive plant life.
Defining Cell Theory
The Cell Theory is a cornerstone of modern biology. It provides the foundation for understanding the organization and function of all living organisms.
The Cell Theory's core tenets can be summarized as follows:
- All living organisms are composed of one or more cells.
- The cell is the basic unit of structure and function in living organisms.
- All cells arise from pre-existing cells through cell division.
This theory underscores the importance of cells as the fundamental building blocks of life and highlights their role in inheritance and development.
Understanding Cell Structure
Cell structure refers to the arrangement of various components within a cell. These components, known as organelles, each perform specific functions that contribute to the overall activity and survival of the cell.
The organization of these components dictates how the cell functions. Understanding cell structure is crucial for deciphering the mechanisms that govern cellular processes.
Eukaryotic Cells Explained
Eukaryotic cells are characterized by their complex internal organization.
The presence of membrane-bound organelles, such as the nucleus, mitochondria, and chloroplasts (in plant cells), distinguishes them from prokaryotic cells. The nucleus, houses the cell's genetic material.
This compartmentalization allows for greater efficiency and specialization of cellular functions. Plant and animal cells are prime examples of eukaryotic cells.
Contrasting with Prokaryotic Cells
Prokaryotic cells represent a simpler form of cellular organization. They lack a defined nucleus and other membrane-bound organelles.
Bacteria and archaea are examples of prokaryotic organisms. Their genetic material is typically located in a region called the nucleoid.
Understanding the differences between eukaryotic and prokaryotic cells is essential for appreciating the unique features of plant cells, which belong to the eukaryotic domain. These differences highlight the evolutionary pathways that have led to the diversity of life on Earth.
Eukaryotic vs. Prokaryotic Cells: A Comparative Overview
Understanding plant cell biology is fundamental to grasping the complexities of plant life. Plants, as the primary producers in most ecosystems, play a critical role in sustaining life on Earth. Their unique cellular structures and processes are essential for photosynthesis, growth, and adaptation to diverse environments. This section lays the groundwork by comparing and contrasting the two fundamental cell types: eukaryotic and prokaryotic.
Eukaryotic Cells: Complexity and Organization
Eukaryotic cells, characterized by their intricate internal organization, represent a significant step in the evolution of cellular life. The defining feature of a eukaryotic cell is the presence of membrane-bound organelles, most notably the nucleus, which houses the cell's genetic material.
This compartmentalization allows for specialized functions to occur within the cell, increasing efficiency and complexity. Eukaryotic cells are significantly larger and more complex than their prokaryotic counterparts.
Examples of Eukaryotic Cells: Plant and Animal Cells
Both plant and animal cells are prime examples of eukaryotic cells, though they possess distinct characteristics. Plant cells, for instance, contain chloroplasts for photosynthesis and a rigid cell wall for structural support.
Animal cells, conversely, lack these features but may contain structures like centrioles involved in cell division. These differences highlight the adaptability and specialization within the eukaryotic domain.
The Nucleus: The Eukaryotic Control Center
The nucleus is arguably the most important organelle within a eukaryotic cell. It serves as the control center, housing the cell's DNA in the form of chromosomes.
The nuclear envelope, a double membrane structure, regulates the passage of molecules between the nucleus and the cytoplasm, ensuring the integrity and controlled expression of genetic information. The nucleus dictates cell function and heredity.
Prokaryotic Cells: Simplicity and Efficiency
Prokaryotic cells, representing the earlier form of cellular life, are characterized by their simpler structure and organization. Unlike eukaryotic cells, prokaryotic cells lack a membrane-bound nucleus.
Instead, their genetic material resides in the cytoplasm in a region called the nucleoid. Despite their simplicity, prokaryotic cells are incredibly adaptable and diverse, thriving in a wide range of environments.
Bacteria and Archaea: Domains of Prokaryotic Life
The two primary domains of prokaryotic life are Bacteria and Archaea. While both share the fundamental characteristics of prokaryotic cells, they differ in their biochemical and genetic makeup.
Bacteria are ubiquitous, playing critical roles in nutrient cycling and decomposition. Archaea, often found in extreme environments, exhibit unique metabolic pathways.
Absence of a Defined Nucleus: A Defining Characteristic
The absence of a nucleus is the defining feature of prokaryotic cells. The genetic material, typically a single circular chromosome, is located in the nucleoid region, freely interacting with the cytoplasm.
This direct interaction allows for rapid protein synthesis and adaptation to changing environmental conditions. This is a key difference from eukaryotes.
In conclusion, understanding the fundamental differences between eukaryotic and prokaryotic cells is essential for appreciating the diversity and complexity of life. The more complex eukaryotes, including plant cells, build on these foundations to enable the evolution of specialized structures and functions.
[Eukaryotic vs. Prokaryotic Cells: A Comparative Overview Understanding plant cell biology is fundamental to grasping the complexities of plant life. Plants, as the primary producers in most ecosystems, play a critical role in sustaining life on Earth. Their unique cellular structures and processes are essential for photosynthesis, growth, and adapt...]
Having established the foundational concepts of cell biology and differentiated between eukaryotic and prokaryotic cells, it is now prudent to focus specifically on plant cells. These cells, the fundamental units of all plants, exhibit unique characteristics that enable them to perform essential functions, setting them apart from other cell types, notably animal cells.
The Kingdom Plantae: A Taxonomic Overview
Plants belong to the Kingdom Plantae, one of the largest and most diverse kingdoms within the domain Eukaryota. This kingdom encompasses a vast array of organisms, from microscopic algae to towering trees, all characterized by their ability to perform photosynthesis. Understanding the taxonomic classification of plants provides a framework for studying their evolutionary relationships and ecological roles.
The defining feature of plants is their ability to synthesize their own food through photosynthesis, a process that converts light energy into chemical energy.
This autotrophic mode of nutrition distinguishes them from animals, which are heterotrophic and must consume other organisms for sustenance. The Kingdom Plantae's diverse members share the common trait of complex, multicellular eukaryotic organization, adapted for terrestrial or aquatic environments.
Defining the Plant Cell: The Basic Unit of Plant Life
A plant cell is the fundamental structural and functional unit of all plants.
Like all eukaryotic cells, it possesses a complex internal organization, with various organelles performing specialized tasks. However, plant cells possess unique features that distinguish them from animal cells, enabling them to carry out functions specific to plant life.
These distinctive characteristics are essential for processes like photosynthesis, structural support, and nutrient storage.
Unique Features of Plant Cells
Plant cells exhibit several unique features not found in animal cells, each playing a crucial role in plant function.
Cell Wall: Providing Structure and Support
One of the most prominent distinctions is the presence of a cell wall, a rigid outer layer that provides structural support and protection to the cell. This wall, primarily composed of cellulose, gives plant cells their characteristic shape and prevents them from bursting due to osmotic pressure.
The cell wall also plays a role in regulating cell growth and differentiation, influencing the overall architecture of the plant.
Chloroplasts: The Site of Photosynthesis
Another defining feature of plant cells is the presence of chloroplasts, organelles responsible for carrying out photosynthesis.
These organelles contain chlorophyll, the green pigment that captures light energy from the sun, enabling plants to convert carbon dioxide and water into glucose and oxygen.
Photosynthesis is the cornerstone of plant life, providing the energy and nutrients necessary for growth and survival.
Other Distinguishing Features
In addition to the cell wall and chloroplasts, plant cells often contain a large central vacuole, which stores water, nutrients, and waste products. This vacuole also helps maintain turgor pressure, contributing to the rigidity of the plant.
Plant cells also differ from animal cells in their lack of centrioles, structures involved in cell division in animal cells.
Plant Cell vs. Animal Cell: A Comparative Overview
A comparison of plant and animal cells reveals fundamental differences in structure and function. Plant cells possess a cell wall and chloroplasts, while animal cells lack these features. Animal cells have centrioles, which plant cells do not. Additionally, plant cells have a large central vacuole, whereas animal cells have smaller vacuoles, if any.
These structural differences reflect the distinct roles of plant and animal cells in their respective organisms. Plant cells are specialized for photosynthesis, structural support, and nutrient storage, while animal cells are adapted for movement, nerve impulse transmission, and other functions specific to animal life.
Understanding these differences is crucial for appreciating the diversity and complexity of life at the cellular level.
Plant Cell Structure: A Detailed Look at the Components
Understanding plant cell biology is fundamental to grasping the complexities of plant life. To truly appreciate the intricacies of plant cells, we must delve into a detailed examination of their individual components and how these components work together.
This section provides a comprehensive overview of the structures within a plant cell. It will cover the various organelles and their specific roles, emphasizing how they interact to maintain cellular function.
Unveiling the Cellular Architecture
Plant cells, like all eukaryotic cells, are highly organized structures with a division of labor among their components. These components, known as organelles, perform specific functions essential for the cell's survival and operation. The coordinated action of these organelles dictates the overall health and function of the plant.
Each organelle plays a crucial role in various aspects of plant cell life, from energy production and waste management to protein synthesis and structural support. Examining these organelles in detail provides insight into the inner workings of plant life.
Core Organelles and Their Interactions
At the heart of cellular activity are key organelles like the nucleus, chloroplasts, mitochondria, endoplasmic reticulum, Golgi apparatus, and vacuoles. The nucleus houses the cell's genetic material (DNA), dictating the production of proteins and enzymes that drive cellular processes.
Chloroplasts are responsible for photosynthesis, the process by which plants convert light energy into chemical energy. Mitochondria are the powerhouses of the cell, generating energy through cellular respiration.
The endoplasmic reticulum (ER) and Golgi apparatus work in tandem to synthesize and process proteins and lipids. They prepare molecules for transportation to their designated locations within the cell or secretion outside the cell. Vacuoles serve as storage facilities, holding water, nutrients, and waste products.
These organelles do not operate in isolation; they are interconnected in a complex network of interactions and dependencies. The ER, for example, interacts closely with the Golgi apparatus to modify and package proteins synthesized by ribosomes.
Mitochondria rely on proteins and lipids produced by the ER and Golgi apparatus to maintain their structure and function. This intricate dance of interaction is essential for the cell's coordinated activities.
The Gatekeeper: Cell Membrane (Plasma Membrane)
The cell membrane, or plasma membrane, acts as the interface between the cell and its environment. The structure regulates the movement of substances in and out of the cell. Its selective permeability is crucial for maintaining the optimal internal environment required for cellular function.
Composed of a phospholipid bilayer with embedded proteins, the plasma membrane allows certain molecules to pass through while restricting others. This selective barrier is essential for regulating nutrient uptake, waste removal, and the maintenance of proper ion concentrations.
Furthermore, the plasma membrane plays a vital role in cell signaling, receiving and transmitting external signals that influence cellular behavior. This makes it a key player in coordinating cellular responses to the environment.
Plant Cell vs. Animal Cell: A Comparative Analysis
While both plant and animal cells are eukaryotic, they exhibit key structural differences that reflect their distinct functions. Plant cells, for example, possess a rigid cell wall composed primarily of cellulose. This is a feature absent in animal cells. The cell wall provides structural support, protection, and shape to the plant cell.
Plant cells also contain chloroplasts, the site of photosynthesis, which are not found in animal cells. Conversely, animal cells possess centrioles, which are involved in cell division and are typically absent in plant cells.
Furthermore, plant cells often have a large central vacuole that occupies a significant portion of the cell volume. This vacuole stores water, nutrients, and waste products and plays a role in maintaining cell turgor pressure. Animal cells, in contrast, have smaller vacuoles, and animal cells lack cell walls.
These structural differences underscore the distinct roles and adaptations of plant and animal cells, reflecting their divergent evolutionary pathways and functions within their respective organisms.
Key Plant Cell Organelles and Their Functions
Understanding plant cell biology is fundamental to grasping the complexities of plant life. To truly appreciate the intricacies of plant cells, we must delve into a detailed examination of their individual components and how these components work together.
This section focuses on the critical organelles found within plant cells, explaining their structures and functions. It will cover the nucleus, chloroplasts, and mitochondria in detail, emphasizing their roles in key cellular processes.
Organelles: Specialized Units of Cellular Function
Within the intricate world of the plant cell, various membrane-bound structures called organelles perform specialized tasks, contributing to the cell's overall function and survival. Each organelle is uniquely designed to carry out its specific job, allowing the cell to operate efficiently.
These structures work in concert to maintain cellular homeostasis and carry out life processes. Understanding the roles of these organelles is key to understanding plant cell biology.
The Nucleus: The Cell's Control Center
The nucleus is often regarded as the control center of the plant cell. This prominent organelle houses the cell’s genetic material, DNA, organized into chromosomes.
The nucleus dictates cellular activities through the transcription of genes and plays a pivotal role in cell division. Its double-membrane structure, called the nuclear envelope, regulates the movement of molecules between the nucleus and the cytoplasm.
Chloroplasts: Harnessing Light Energy
Chloroplasts are perhaps the most distinctive organelles found in plant cells, primarily responsible for photosynthesis. These organelles contain chlorophyll, a pigment that captures light energy.
Through photosynthesis, light energy is converted into chemical energy in the form of glucose. This process not only sustains the plant but also releases oxygen as a byproduct, vital for many life forms.
The intricate internal structure of chloroplasts, including thylakoids and grana, facilitates efficient light capture and energy conversion. Chloroplasts are essential for all plant life.
Other Significant Plant Cell Organelles
Beyond the nucleus and chloroplasts, several other organelles play crucial roles in plant cell function:
-
Vacuoles: These large, fluid-filled sacs are involved in storage, waste disposal, and maintaining turgor pressure. They can occupy a significant portion of the cell volume.
-
Endoplasmic Reticulum (ER): A network of membranes involved in protein and lipid synthesis. The ER can be smooth or rough, depending on the presence of ribosomes.
-
Golgi Apparatus: This organelle processes and packages proteins and lipids for transport to other locations within or outside the cell. It plays a crucial role in cellular secretion.
-
Ribosomes: Responsible for protein synthesis. Ribosomes can be found free in the cytoplasm or attached to the endoplasmic reticulum.
-
Peroxisomes: These organelles are involved in various metabolic processes, including the breakdown of fatty acids and detoxification.
Mitochondria: The Powerhouse of the Cell
Mitochondria are often referred to as the "powerhouses" of the cell. These organelles are responsible for cellular respiration, a process that converts glucose into ATP (adenosine triphosphate), the cell's primary energy currency.
With their double-membrane structure, including the highly folded inner membrane forming cristae, mitochondria maximize the surface area for ATP production. This efficient energy generation is crucial for all cellular activities.
Understanding the structure and function of these key plant cell organelles provides a foundation for comprehending the complex processes that sustain plant life. The coordinated activity of these organelles is essential for the growth, development, and survival of plants.
Unique Plant Cell Structures: The Cell Wall and Cytoplasm
Understanding plant cell biology is fundamental to grasping the complexities of plant life. To truly appreciate the intricacies of plant cells, we must delve into a detailed examination of their individual components and how these components work together.
This section highlights structures that are often unique to plant cells, specifically the cell wall and the cytoplasm. It will explain their composition and their functions in providing support, protection, and a medium for cellular activities.
The Indispensable Cell Wall
The cell wall is a defining feature of plant cells, distinguishing them from animal cells and many other eukaryotic organisms. It is a rigid, complex outer layer that surrounds the plasma membrane, providing structural support and protection against external stresses. Its presence is essential for maintaining cell shape, turgor pressure, and overall plant rigidity.
Composition of the Cell Wall
The primary component of the plant cell wall is cellulose, a polysaccharide composed of long chains of glucose molecules. These cellulose chains are organized into microfibrils, which provide tensile strength and form a network within the cell wall matrix.
Other components of the cell wall include hemicellulose, pectin, lignin, and various proteins.
Hemicellulose and pectin contribute to the flexibility and elasticity of the cell wall. Lignin, a complex polymer, provides additional rigidity and impermeability, particularly in woody tissues. The specific composition and organization of the cell wall can vary depending on the plant species, cell type, and stage of development.
Functions of the Cell Wall
The cell wall performs several critical functions that are essential for plant survival and growth.
First and foremost, it provides structural support, allowing plants to maintain their shape and withstand external forces such as wind and gravity. The rigidity of the cell wall also helps to maintain turgor pressure, which is the pressure exerted by the cell contents against the cell wall. Turgor pressure is essential for cell expansion, plant growth, and maintaining the rigidity of non-woody tissues.
The cell wall also acts as a protective barrier, shielding the cell from pathogens, dehydration, and mechanical damage. It can also regulate the movement of molecules into and out of the cell, acting as a filter or selective barrier.
The Cytoplasm: The Cell's Internal Environment
The cytoplasm is the gel-like substance that fills the interior of the cell, surrounding the organelles and providing a medium for cellular activities. It is composed primarily of water, but also contains a variety of dissolved molecules, including ions, proteins, carbohydrates, lipids, and nucleic acids.
The cytoplasm is not simply a passive filler; it is a dynamic and highly organized environment that plays a crucial role in many cellular processes.
Role in Cellular Processes
The cytoplasm provides a medium for biochemical reactions, allowing enzymes and substrates to interact efficiently. It also facilitates the transport of molecules within the cell, ensuring that organelles receive the necessary resources and that waste products are removed.
The cytoplasm also contains the cytoskeleton, a network of protein filaments that provides structural support and helps to organize the organelles. The cytoskeleton plays a role in cell division, cell movement, and cell signaling.
In conclusion, the cell wall and cytoplasm are essential components of plant cells, each playing a unique role in maintaining cell structure, function, and overall plant health. Understanding these structures is crucial for comprehending the complexities of plant cell biology and the vital processes that sustain plant life.
The Molecular Basis of Plant Life: DNA
Understanding plant cell biology is fundamental to grasping the complexities of plant life. To truly appreciate the intricacies of plant cells, we must delve into a detailed examination of their individual components and how these components work together.
This section provides a focused introduction to the role of DNA within plant cells, highlighting its pivotal function as the carrier of genetic information and its crucial role in regulating a myriad of cellular processes. DNA is not merely a molecule; it is the very blueprint of life.
DNA: The Blueprint of Plant Life
Deoxyribonucleic acid (DNA) serves as the fundamental carrier of genetic information in plant cells, as it does in nearly all living organisms. This molecule is responsible for encoding the instructions that determine the development, function, and reproduction of the plant.
It's more than just a molecule; it's the instruction manual passed down through generations.
The Structure of DNA
The elegant structure of DNA, famously described as a double helix, is critical to its function. This structure comprises two strands of nucleotides that coil around each other.
Each nucleotide consists of a deoxyribose sugar, a phosphate group, and one of four nitrogenous bases: adenine (A), guanine (G), cytosine (C), and thymine (T).
The sequence of these bases along the DNA strand encodes the genetic information.
DNA's Role in Heredity
DNA's primary role is in heredity, the passing of traits from parents to offspring. During reproduction, DNA is replicated and transmitted to new cells, ensuring the continuity of genetic information.
This process allows offspring to inherit the characteristics of their parents, with slight variations that drive evolution.
DNA and Protein Synthesis
DNA directs the synthesis of proteins, the workhorses of the cell. Through a process called gene expression, the information encoded in DNA is transcribed into RNA (ribonucleic acid), which is then translated into proteins.
These proteins perform a vast array of functions, from catalyzing biochemical reactions to providing structural support.
Regulation of Cellular Processes
Beyond encoding genetic information, DNA also plays a critical role in regulating cellular processes. Gene regulation mechanisms control when and where specific genes are expressed, allowing plant cells to respond to environmental cues and developmental signals.
This regulation ensures that the right proteins are produced at the right time and in the right amounts, maintaining cellular homeostasis.
DNA in Plant Breeding and Genetic Engineering
Our understanding of DNA has revolutionized plant breeding and genetic engineering. Scientists can now manipulate plant DNA to introduce desirable traits, such as disease resistance, improved yield, or enhanced nutritional content.
These advancements have the potential to address global challenges related to food security and sustainable agriculture.
The Importance of DNA Studies in Plants
Studying DNA is fundamental to understanding plant evolution, adaptation, and response to environmental changes. Through DNA analysis, scientists can trace the ancestry of plant species, identify genes that confer resistance to stress, and develop strategies for conserving plant biodiversity.
DNA's role in plant cells is multifaceted, encompassing heredity, protein synthesis, regulation, and adaptation. It is, without question, the molecular cornerstone of plant life.
Essential Biological Processes in Plant Cells: Photosynthesis and More
Understanding plant cell biology is fundamental to grasping the complexities of plant life. To truly appreciate the intricacies of plant cells, we must delve into a detailed examination of their individual components and how these components work together.
This section provides a focused introduction to the essential biological processes within plant cells, highlighting photosynthesis as the cornerstone of plant life, while also considering broader cellular and biological contexts.
Photosynthesis: The Engine of Plant Life
Photosynthesis, the process by which plants convert light energy into chemical energy, is arguably the most crucial biological function occurring within plant cells. This process, primarily executed within the chloroplasts, enables plants to synthesize glucose (a sugar) from carbon dioxide and water, releasing oxygen as a byproduct.
The implications of photosynthesis extend far beyond the individual plant.
It serves as the primary entry point of energy into most ecosystems, forming the base of the food chain. Without photosynthesis, most life on Earth, as we know it, would be unsustainable.
Furthermore, the oxygen released during photosynthesis is critical for the respiration of countless organisms, including humans.
The Importance of Cell Biology
The study of cell biology provides the framework for understanding these complex processes. By examining the structure and function of individual cellular components, we can decipher how photosynthesis is regulated and how environmental factors impact its efficiency.
Cell biology provides the essential tools and knowledge for addressing critical challenges such as improving crop yields and developing sustainable energy sources.
Plant Biology: A Holistic Perspective
Plant biology offers a broader perspective, encompassing not only the cellular level but also the whole organism and its interactions with the environment. Understanding plant biology allows us to investigate how photosynthetic efficiency varies among different plant species and how plants adapt to diverse environmental conditions.
This holistic approach is essential for developing strategies to conserve plant biodiversity and manage ecosystems effectively.
Eukaryotic Characteristics in Plant Cells
Plant cells, being eukaryotic, possess distinctive characteristics that are crucial for their photosynthetic capabilities. The presence of membrane-bound organelles, such as chloroplasts, allows for specialized compartments where photosynthesis can occur efficiently.
The complex organization of the eukaryotic cell is essential for coordinating the various metabolic pathways involved in photosynthesis.
Moreover, other unique characteristics of plant cells, such as the cell wall, contribute to their structural integrity and ability to withstand environmental stressors.
Pioneers of Plant Cell Biology: Matthias Schleiden
Understanding plant cell biology is fundamental to grasping the complexities of plant life. To truly appreciate the intricacies of plant cells, we must delve into a detailed examination of their individual components and how these components work together.
This section acknowledges the contributions of key scientists who have advanced our understanding of plant cell biology. It highlights the role of Matthias Schleiden in the development of cell theory.
Schleiden and the Genesis of Cell Theory
Matthias Jakob Schleiden, a German botanist, stands as a pivotal figure in the history of cell biology. His meticulous observations and insightful interpretations were instrumental in shaping the cell theory, a cornerstone of modern biology.
Schleiden's work, primarily focused on plant tissues, led him to propose a revolutionary idea: that all plants are composed of cells. This might seem self-evident today, but in the 19th century, it was a groundbreaking concept.
The Botanist's Perspective
Unlike some of his contemporaries who focused on animal tissues, Schleiden's botanical background provided a unique lens through which to view cellular structures. His detailed studies of plant cells revealed the presence of distinct nuclei within these cells.
This observation was crucial, as it suggested that the nucleus played a vital role in the cell's development and function.
Challenging Preconceived Notions
Before Schleiden's work, the prevailing view of plant structure was far less defined. Many scientists believed that plants were composed of interconnected, amorphous tissues, rather than discrete cellular units.
Schleiden's research challenged this notion, demonstrating that plants, like animals, are fundamentally cellular organisms.
Collaboration and Confirmation
It is important to note that the cell theory was not solely the product of Schleiden's work. He collaborated with Theodor Schwann, a zoologist, who extended Schleiden's findings to animal tissues.
Schwann's confirmation that animal tissues are also composed of cells solidified the cell theory as a universal principle of biology.
The Enduring Legacy of Schleiden
While some of Schleiden's initial ideas about cell formation were later proven incorrect, his contribution to the cell theory remains undeniable.
He provided a crucial piece of the puzzle, demonstrating the cellular nature of plants and paving the way for future discoveries in cell biology.
His emphasis on the importance of the nucleus as a key cellular component was particularly significant.
Schleiden's meticulous observations, combined with Schwann's confirmation in animal tissues, established the cell theory as a fundamental principle of biology. This laid the foundation for understanding the intricate workings of life at its most basic level.
Tools and Techniques for Studying Plant Cells
Understanding plant cell biology is fundamental to grasping the complexities of plant life. To truly appreciate the intricacies of plant cells, we must delve into a detailed examination of their individual components and how these components work together.
This section introduces the essential tools and techniques used in plant cell biology research. It emphasizes the importance of microscopy in observing cells and their structures, which are paramount for detailed cellular analysis.
The Indispensable Role of Microscopy
Microscopy serves as the cornerstone of plant cell biology. Without the ability to visualize cells and their intricate components, our understanding would remain rudimentary.
The development and refinement of microscopes have driven major breakthroughs in our knowledge of plant life at the cellular level. Microscopy allows researchers to observe cellular structures, dynamic processes, and responses to various stimuli.
Light Microscopy: A Foundation for Cellular Observation
Light microscopy is one of the most accessible and widely used techniques in plant cell biology. It utilizes visible light to illuminate and magnify samples, providing a foundational method for cellular observation.
Principles of Light Microscopy
Light microscopes use a system of lenses to focus light through a sample, creating a magnified image. Magnification, resolution, and contrast are the key factors determining the quality of the observed image. Different light microscopy techniques can enhance contrast and reveal specific cellular components.
Applications of Light Microscopy
Light microscopy is invaluable for observing living cells and dynamic cellular processes. Techniques such as phase contrast and differential interference contrast (DIC) enhance the visibility of transparent structures without staining.
Fluorescence microscopy, a specialized form of light microscopy, uses fluorescent dyes or proteins to label specific cellular components. This enables researchers to visualize the location and movement of molecules within cells.
Electron Microscopy: Unveiling the Ultrastructure of Plant Cells
Electron microscopy provides significantly higher magnification and resolution than light microscopy. This allows for the detailed visualization of cellular ultrastructure.
Principles of Electron Microscopy
Electron microscopes use beams of electrons instead of light to image samples. Because electrons have a much shorter wavelength than visible light, electron microscopes can resolve structures at the nanometer scale.
There are two primary types of electron microscopy: transmission electron microscopy (TEM) and scanning electron microscopy (SEM).
Transmission Electron Microscopy (TEM)
TEM involves transmitting a beam of electrons through a thin sample. Electrons interact with the sample, and the resulting image reveals the internal structure of cells and organelles with exceptional detail.
TEM is particularly useful for visualizing membranes, ribosomes, and other sub cellular structures.
Scanning Electron Microscopy (SEM)
SEM involves scanning a focused beam of electrons across the surface of a sample. Electrons reflected from the surface are detected to create a three-dimensional image of the sample's topography.
SEM is ideal for studying the surface features of plant cells, such as cell walls, trichomes, and stomata.
Sample Preparation Techniques
Proper sample preparation is crucial for obtaining high-quality images with both light and electron microscopy. Preparation techniques vary depending on the type of microscopy used and the specific structures being studied.
Fixation and Staining
Fixation is used to preserve cellular structures by cross-linking proteins and other macromolecules. Staining enhances contrast and highlights specific cellular components.
Different stains selectively bind to certain molecules, allowing researchers to visualize their distribution within cells.
Embedding and Sectioning
For TEM, samples are typically embedded in resin and sectioned into ultra-thin slices using an ultramicrotome. This allows electrons to pass through the sample and create a high-resolution image.
Immunolabeling
Immunolabeling involves using antibodies to specifically label target molecules within cells. The antibodies are conjugated to fluorescent dyes or electron-dense particles.
This technique enables researchers to visualize the location of specific proteins and other molecules within cells.
Emerging Microscopy Techniques
The field of microscopy is constantly evolving. New techniques, such as super-resolution microscopy and cryo-electron microscopy, are pushing the boundaries of what can be visualized in plant cells.
These advanced techniques offer the potential to reveal even more detail about the structure and function of plant cells. They also provide new insights into the complex processes that govern plant life.
Video: Are Plantae Prokaryotic or Eukaryotic? Plant Cell
Frequently Asked Questions About Plant Cells and Their Classification
Are Plantae organisms prokaryotic or eukaryotic?
Plantae are eukaryotic. All organisms in the Plantae kingdom are composed of eukaryotic cells. This means their cells have a nucleus and other membrane-bound organelles. Therefore, are plantae prokaryotic or eukaryotic? They are definitively eukaryotic.
What is a key difference between prokaryotic and plant cells?
The most significant difference is the presence of a nucleus. Plant cells, being eukaryotic, have a nucleus that houses their DNA. Prokaryotic cells, like bacteria, lack a defined nucleus; their DNA floats freely in the cytoplasm. So, while both types of cells contain genetic information, how it's organized drastically differs, classifying are plantae prokaryotic or eukaryotic correctly.
Do plant cells possess membrane-bound organelles?
Yes, plant cells are characterized by having various membrane-bound organelles such as mitochondria, chloroplasts (for photosynthesis), and a Golgi apparatus. These organelles perform specific functions within the cell. The presence of these organelles confirms why are plantae prokaryotic or eukaryotic and their classification as eukaryotes.
What cellular structure is unique to plant cells, and does it exist in prokaryotes?
Plant cells have a rigid cell wall made of cellulose. Prokaryotic cells also have cell walls, but their composition is different (e.g., peptidoglycan in bacteria). However, the plant cell wall's cellulose structure is unique. This, alongside other features, clearly shows why are plantae prokaryotic or eukaryotic – the complex structure excludes them from being prokaryotic.
So, are plantae prokaryotic or eukaryotic? The answer is a resounding eukaryotic! Hopefully, this clears up any confusion. Now you can confidently tell your friends all about the wonderful world of plant cells and their complex inner workings. Happy learning!