Phosphate Buffer System: A Beginner's Guide
The phosphate buffer system, a crucial mechanism in maintaining cellular pH, operates primarily within the intracellular fluid, where the concentration of phosphate is significantly higher compared to the extracellular space; cellular metabolism generates acids which affect the intracellular environment. Its efficacy relies on the interplay between dihydrogen phosphate ($H_2PO_4^−$) and hydrogen phosphate ($HPO_4^{2−}$), acting as a proton donor and acceptor, respectively. In biological research, scientists at institutions like the National Institutes of Health (NIH) frequently utilize the Henderson-Hasselbalch equation to calculate and prepare phosphate buffers with specific pH values for in vitro experiments. Proper application of the phosphate buffer system is critical for ensuring accurate and reliable results in biochemical assays and cell culture studies because pH affects the protein.
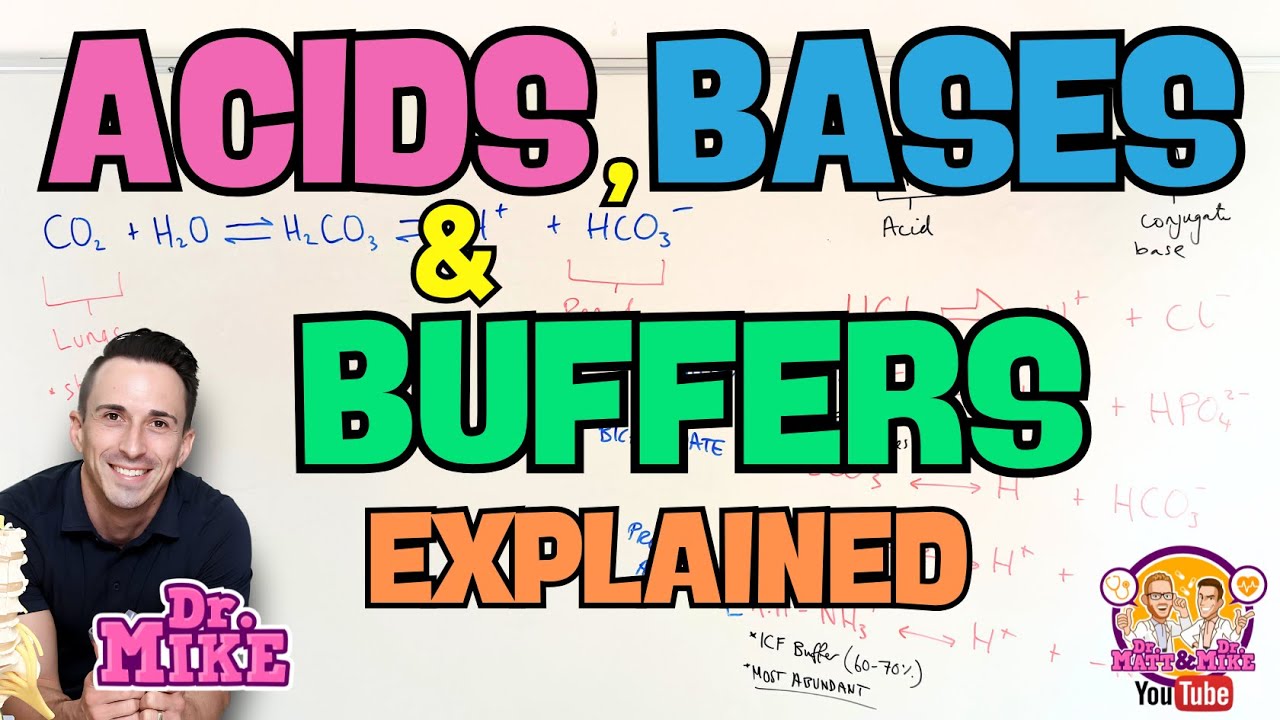
Image taken from the YouTube channel Dr Matt & Dr Mike , from the video titled Acids, Bases, and Buffers .
The Vital Role of Buffers and pH
Buffers are indispensable in maintaining stable pH levels in a multitude of chemical and biological systems. Understanding their function is paramount for anyone working in scientific or industrial fields. Let's explore why.
Defining Buffers
At their core, buffers are substances designed to resist changes in pH. This resistance is crucial because many chemical and biological reactions are highly sensitive to pH fluctuations. Introduce a strong acid or base, and a buffer will work to neutralize the impact, keeping the pH within a narrow, functional range.
Understanding pH and Its Measurement
pH, or potential of hydrogen, is a measure of the acidity or alkalinity of a solution. It’s quantified on a scale from 0 to 14.
A pH of 7 is considered neutral. Values below 7 indicate acidity, while values above 7 indicate alkalinity or basicity. The pH scale is logarithmic, meaning each whole number change represents a tenfold difference in acidity or alkalinity.
Measuring pH typically involves using a pH meter, an electronic instrument that detects the hydrogen ion concentration in a solution, or through the use of chemical indicators that change color depending on the pH.
The Importance of pH Stability
pH stability is not just a matter of precision. In biological systems, it's a matter of life and death.
Enzymes, the catalysts of biochemical reactions, function optimally within specific pH ranges. Even slight deviations can drastically reduce their activity or denature them completely.
Similarly, in industrial processes, maintaining a consistent pH can be crucial for reaction yields and product quality. Uncontrolled pH shifts can lead to unwanted side reactions, reduced efficiency, or even complete failure of the process.
Acid-Base Chemistry and Proton Dynamics
The functionality of buffers is rooted in acid-base chemistry. Acids are substances that donate protons (H⁺ ions), while bases accept them.
A buffer system typically consists of a weak acid and its conjugate base (or a weak base and its conjugate acid). This pairing allows the buffer to neutralize both added acids and bases, maintaining a stable pH. The equilibrium between the acid and base forms is what gives a buffer its capacity to resist pH changes.
In essence, buffers are dynamic systems that respond to changes in their environment by shifting the equilibrium between proton donors and acceptors. This helps stabilize the pH and allowing critical processes to proceed without disruption.
Deconstructing the Phosphate Buffer System: Components Unveiled
Now that we understand the fundamental role of buffers, let's delve into the specific components that constitute the phosphate buffer system. Unveiling these components and their individual roles is crucial for comprehending the system's overall function.
Key Chemical Species
The phosphate buffer system relies on a delicate balance of several key chemical species, each with a distinct role in maintaining pH stability. Let's examine these components in detail:
-
Phosphate (PO₄³⁻): At its core, the phosphate ion (PO₄³⁻) represents the fully deprotonated form.
Its fundamental role is to act as a reservoir for accepting protons (H+), counteracting acidity and preventing drastic pH drops.
-
Dihydrogen Phosphate (H₂PO₄⁻): Dihydrogen phosphate (H₂PO₄⁻) is a protonated form of phosphate.
It functions primarily as a proton donor. Its ability to release H+ ions when the pH increases helps to resist alkalinity.
-
Hydrogen Phosphate (HPO₄²⁻): Hydrogen phosphate (HPO₄²⁻) represents an intermediate state, possessing one proton.
This species can act as both a proton donor and acceptor, enabling it to respond to pH changes in either direction, contributing to the buffer system's versatility.
-
Phosphoric Acid (H₃PO₄): Phosphoric acid (H₃PO₄) stands as the fully protonated form and represents the source acid of the system.
While less prevalent at physiological pH, it plays a role in establishing the equilibrium and buffering capacity, especially in more acidic conditions.
Chemical Formulas and Their Significance
Understanding the chemical formulas is essential for grasping how each species contributes to the buffering process.
Each formula represents a specific arrangement of atoms and charges, dictating its interaction with hydrogen ions (H+) and its role in maintaining equilibrium.
The interplay between these different forms of phosphate, governed by the principles of acid-base chemistry, is what enables the phosphate buffer system to effectively resist pH fluctuations. This dynamic equilibrium is the key to its buffering action.
Mechanism of Action: How the Phosphate Buffer System Works
To truly appreciate the effectiveness of the phosphate buffer system, it’s essential to understand the chemical reactions and equilibrium shifts that underpin its ability to resist pH changes. This buffering capacity stems from the interplay between the dihydrogen phosphate ion (H₂PO₄⁻) and the hydrogen phosphate ion (HPO₄²⁻), enabling it to neutralize both added acids and bases efficiently.
Reaction Equations and Equilibrium
The core of the phosphate buffer system lies in the reversible reaction between its two primary components:
H₂PO₄⁻ (aq) ⇌ H⁺ (aq) + HPO₄²⁻ (aq)
This equilibrium, governed by the acid dissociation constant (Ka), allows the system to adapt to changes in hydrogen ion concentration. The system acts as a dynamic reservoir for protons, readily releasing or absorbing them to maintain a stable pH.
Buffering Against Added Acid (H⁺)
When an acid is added to the system, increasing the concentration of H⁺ ions, the equilibrium shifts to the left.
The hydrogen phosphate ion (HPO₄²⁻) acts as a base and accepts the excess H⁺ ions to form dihydrogen phosphate (H₂PO₄⁻):
HPO₄²⁻ (aq) + H⁺ (aq) → H₂PO₄⁻ (aq)
By consuming the added H⁺ ions, the buffer minimizes the decrease in pH, effectively neutralizing the acid.
Buffering Against Added Base (OH⁻)
Conversely, when a base is added, it consumes H⁺ ions, thus reducing their concentration in the solution.
To counteract this, the equilibrium shifts to the right. The dihydrogen phosphate ion (H₂PO₄⁻) acts as an acid and donates H⁺ ions to neutralize the added hydroxide (OH⁻) ions:
H₂PO₄⁻ (aq) + OH⁻ (aq) → HPO₄²⁻ (aq) + H₂O (l)
By releasing H⁺ ions, the buffer minimizes the increase in pH, effectively neutralizing the base. The continuous interconversion between H₂PO₄⁻ and HPO₄²⁻ ensures the pH remains relatively stable, within the buffer's effective range. This dynamic equilibrium is the cornerstone of the phosphate buffer system's ability to maintain pH homeostasis.
Calculating Buffer pH: Mastering the Henderson-Hasselbalch Equation
To fully harness the power of phosphate buffers, it is essential to accurately determine the pH of the buffer solution. The Henderson-Hasselbalch equation provides a straightforward method to achieve this, connecting the pH to the acid dissociation constant (pKa) and the relative concentrations of the buffer's components. This section will thoroughly explain the equation, its variables, and practical applications in buffer preparation.
Understanding the Henderson-Hasselbalch Equation
The Henderson-Hasselbalch equation is defined as follows:
pH = pKa + log([A⁻]/[HA])
Where:
- pH represents the acidity or basicity of the solution.
- pKa is the negative logarithm of the acid dissociation constant (Ka) and indicates the acid strength.
- [A⁻] is the concentration of the conjugate base.
- [HA] is the concentration of the weak acid.
This equation elegantly demonstrates that the pH of a buffer solution is determined by the pKa of the weak acid and the ratio of the concentrations of the conjugate base and weak acid. Understanding these variables is crucial for accurately calculating and adjusting buffer pH.
Practical Application: Calculating Buffer pH
Let's illustrate the utility of the Henderson-Hasselbalch equation with a practical example. Suppose you want to prepare a phosphate buffer using dihydrogen phosphate (H₂PO₄⁻) as the weak acid and hydrogen phosphate (HPO₄²⁻) as the conjugate base. The pKa of H₂PO₄⁻ is approximately 7.2.
If you prepare a solution with equal concentrations of H₂PO₄⁻ and HPO₄²⁻ (i.e., [H₂PO₄⁻] = [HPO₄²⁻]), the equation becomes:
pH = 7.2 + log(1)
Since log(1) = 0, the pH of the buffer will be 7.2.
Now, if you have a solution where the concentration of HPO₄²⁻ is twice that of H₂PO₄⁻ (i.e., [HPO₄²⁻] = 2[H₂PO₄⁻]), the equation becomes:
pH = 7.2 + log(2) pH ≈ 7.2 + 0.301 pH ≈ 7.5
This calculation demonstrates how changing the ratio of conjugate base to weak acid directly influences the buffer's pH.
Factors Affecting Buffer pH
While the Henderson-Hasselbalch equation provides a theoretical pH, certain factors can influence the actual pH of the buffer solution.
Ionic Strength
The ionic strength of the solution, which is influenced by the presence of other ions, can alter the activity coefficients of the buffer components, leading to deviations from the calculated pH.
Temperature
Temperature can also affect the pKa of the weak acid and thus shift the pH. Most pKa values are temperature-dependent, and significant temperature changes can lead to measurable pH changes. Therefore, it is best practice to measure and adjust the pH at the temperature at which the buffer will be used.
Deciphering the Role of pKa
The pKa is a critical parameter in buffer chemistry. It is the pH at which the concentrations of the weak acid and its conjugate base are equal.
pKa and Buffering Capacity
The pKa value is central to determining a buffer’s effectiveness. A buffer has the highest buffering capacity when the pH of the solution is close to the pKa value of the weak acid. This is because, at this point, there are roughly equal concentrations of the acid and its conjugate base available to neutralize both added acids and bases.
Optimal Buffering Range
Generally, a buffer is most effective within a pH range of ±1 pH unit around its pKa value. In the case of the phosphate buffer (pKa ≈ 7.2), it is most effective between pH 6.2 and 8.2. This range needs to be considered when selecting the phosphate buffer for any experimental conditions. If the desired pH is far from the pKa, a different buffer system might be more appropriate.
Factors Influencing Performance: Optimizing Your Buffer System
To ensure the phosphate buffer system operates at its peak effectiveness, it's essential to understand the various factors that influence its performance. Buffering capacity, environmental conditions, and molarity each play crucial roles in determining how well a phosphate buffer resists changes in pH. Mastering these elements allows for fine-tuning the system to meet specific experimental or application requirements.
Buffering Capacity: Defining the Limits of Effectiveness
Buffering capacity refers to the amount of acid or base a buffer can neutralize before its pH begins to change significantly. It's not an unlimited resource; every buffer has its limits.
Understanding these limits is vital for preventing unexpected pH shifts that could compromise experimental results or the stability of biological samples.
Concentration Effects on Buffering Capacity
The concentration of the buffer components directly impacts its capacity. Higher concentrations generally provide greater buffering capacity, enabling the buffer to neutralize larger amounts of acid or base. However, exceedingly high concentrations can introduce other issues, such as increased ionic strength, which may negatively affect sensitive biological assays.
Buffer Range: Where the Buffer Shines
Each buffer system operates most effectively within a specific pH range, known as the buffer range. This range is typically defined as pH = pKa ± 1. The phosphate buffer system has multiple pKa values due to its polyprotic nature, but the most relevant pKa for physiological applications is around 7.2. Therefore, a phosphate buffer is most effective in the range of approximately 6.2 to 8.2. Outside this range, the buffering capacity diminishes, and the buffer becomes less effective at resisting pH changes.
Environmental Conditions: Temperature and Ionic Interference
The surrounding environment can significantly impact the phosphate buffer system's performance. Temperature and the presence of other ions are particularly important considerations.
Temperature's Influence on pH and Stability
Temperature affects both the pH and the stability of buffer solutions. Changes in temperature can alter the equilibrium constants of the buffering components, leading to slight shifts in pH.
Furthermore, extreme temperatures can degrade the buffer components, reducing its overall effectiveness. For critical applications, it is crucial to prepare and use buffers at a controlled temperature to maintain optimal performance.
Ionic Interference: When Other Ions Interfere
The presence of other ions in the solution can also interfere with the buffer's performance. High concentrations of salts or other charged molecules can alter the ionic strength of the solution, which affects the activity coefficients of the buffer components. This, in turn, can impact the buffer's pH and capacity. Additionally, certain ions may react with the phosphate buffer components, reducing their availability for buffering.
Molarity (M): The Key to Precise Preparation
Molarity (M) is a measure of the concentration of a solution, expressed as moles of solute per liter of solution (mol/L).
Why Molarity Matters
Molarity is essential for accurately preparing buffer solutions. It allows scientists to precisely control the concentration of the buffer components, ensuring the desired buffering capacity and pH. Without a clear understanding of molarity, it is impossible to reliably reproduce buffer solutions or compare results across different experiments.
Calculating Molarity: A Practical Approach
To calculate the molarity of a solution, you need to know the mass of the solute (in grams), the molecular weight of the solute (in g/mol), and the volume of the solution (in liters). The formula is as follows:
Molarity (M) = (Mass of solute (g) / Molecular weight (g/mol)) / Volume of solution (L)
For example, to prepare a 0.1 M solution of monobasic sodium phosphate (NaH₂PO₄, molecular weight = 119.98 g/mol) in 1 liter of water, you would need to dissolve 11.998 grams of NaH₂PO₄ in enough water to make a final volume of 1 liter. Accurate weighing and volume measurement are critical for achieving the desired molarity and ensuring the buffer performs as expected.
Applications in Science: Where Phosphate Buffers Shine
To ensure the phosphate buffer system operates at its peak effectiveness, it's essential to understand the various factors that influence its performance. Buffering capacity, environmental conditions, and molarity each play crucial roles in determining how well a phosphate buffer resists changes in pH. With an understanding of those concepts, let's explore how Phosphate buffers are an indispensable tool across diverse scientific fields, maintaining the crucial pH balance required for countless biological and chemical processes.
Biological Systems: Maintaining Cellular Equilibrium
In biology, the phosphate buffer system is fundamental to maintaining the delicate pH balance within living organisms. It plays a vital role in both intracellular and extracellular fluids, ensuring optimal conditions for cellular function.
Intracellular pH Regulation
Within cells, the phosphate buffer system helps to maintain the pH within a narrow range that is essential for enzyme activity, metabolic processes, and overall cellular health. Without this buffering capacity, fluctuations in pH could disrupt cellular functions, leading to cell damage or death.
Extracellular pH Regulation
Similarly, the phosphate buffer system contributes to maintaining the pH of extracellular fluids, such as blood and interstitial fluid. This is critical for the proper functioning of tissues and organs. Deviations from the normal pH range can have severe physiological consequences.
Biochemical Assays and Protein Stability
Biochemistry relies heavily on phosphate buffers for enzyme assays and protein purification. The precise control of pH is crucial for accurate and reliable results.
Enzyme Assays
Enzyme assays require specific pH conditions to ensure optimal enzyme activity. Phosphate buffers provide the stability needed to maintain these conditions, allowing researchers to accurately measure enzyme kinetics and activity.
Protein Purification and Stability
During protein purification, phosphate buffers help to stabilize proteins by preventing denaturation and aggregation. This is particularly important for maintaining the integrity and functionality of the purified proteins.
Molecular Biology: DNA and RNA Applications
In molecular biology, the phosphate buffer system is essential for various DNA and RNA-related procedures. It ensures the stability and integrity of nucleic acids during experiments.
Phosphate buffers are used in DNA and RNA extraction, purification, and storage to prevent degradation. They are also crucial for maintaining the proper pH during PCR amplification, restriction enzyme digestion, and ligation reactions.
Cell Culture: Optimal Growth Conditions
Cell culture requires a carefully controlled environment, including a stable pH, to promote cell growth and viability. Phosphate buffers help to maintain this stability.
By maintaining a constant pH, phosphate buffers ensure that cells can grow and proliferate under optimal conditions. This is essential for cell-based assays, drug screening, and tissue engineering.
Reagent Formulation: Ensuring Solution Stability
Phosphate buffers are widely used in reagent formulation to stabilize the pH of various solutions. This is particularly important for reagents that are sensitive to pH changes.
The addition of a phosphate buffer helps to prevent pH drift over time, ensuring that the reagents remain effective and reliable. This is crucial for maintaining the accuracy and reproducibility of experimental results.
Phosphate-Buffered Saline (PBS): A Versatile Biological Buffer
Phosphate-Buffered Saline (PBS) is a widely used biological buffer that combines the buffering capacity of phosphate with the isotonic properties of saline. Its biocompatibility makes it ideal for a wide range of applications.
PBS is commonly used for washing cells, diluting samples, and as a base for cell culture media. Its versatility and ease of use have made it an indispensable tool in biological research. The widespread adoption of PBS underscores the critical role that phosphate buffers play in supporting scientific discovery.
Essential Equipment for Preparation: Setting Up Your Lab
Applications in Science: Where Phosphate Buffers Shine To ensure the phosphate buffer system operates at its peak effectiveness, it's essential to understand the various factors that influence its performance. Buffering capacity, environmental conditions, and molarity each play crucial roles in determining how well a phosphate buffer resists change... Now let's explore the laboratory equipment you'll need.
Crafting a reliable phosphate buffer system demands meticulous preparation and, therefore, relies on the use of specific laboratory equipment. Accuracy and precision are paramount when preparing any buffer, as deviations in pH can significantly impact experimental results. A well-equipped lab is not just about having the tools, but also about understanding their importance and proper usage.
Core Equipment for Buffer Preparation
Let's examine the indispensable tools necessary to prepare a phosphate buffer system with accuracy and confidence.
The pH Meter: The Keystone of pH Measurement
A calibrated pH meter is absolutely essential for accurately determining the pH of your buffer solution. It provides a quantitative measurement, allowing for precise adjustments to achieve the desired pH.
Without a reliable pH meter, you are essentially flying blind, unable to confirm the success of your buffering efforts. The pH meter should be calibrated regularly using standard buffer solutions to ensure accuracy.
Beakers and Flasks: Vessels for Reactions
Beakers and flasks, preferably made of borosilicate glass, serve as the containers for dissolving reagents and mixing the buffer solution. Different sizes are needed to accommodate various volumes required for the experiments.
They must be chemically inert and clean to prevent contamination. Erlenmeyer flasks are particularly useful for mixing, while volumetric flasks provide the most accurate volume measurements for preparing stock solutions.
Pipettes and Graduated Cylinders: Measuring Volumes Precisely
Accurate volume measurement is critical for achieving the correct molarity and ratio of acid to conjugate base within the phosphate buffer system. Pipettes (both graduated and volumetric) and graduated cylinders are indispensable tools for dispensing liquids.
Volumetric pipettes deliver a single, highly accurate volume, while graduated pipettes allow for variable volume dispensing. Graduated cylinders are suitable for less critical volume measurements. Employing the correct type of pipette for the desired accuracy is of paramount importance.
Stir Plate and Magnetic Stirrer: Ensuring Homogeneity
A stir plate with a magnetic stirrer ensures thorough and consistent mixing of the buffer components. Homogeneity is critical for a buffer to function properly, as it guarantees that the concentration of each component is uniform throughout the solution.
The magnetic stirrer, placed inside the beaker or flask, is driven by the rotating magnet in the stir plate, creating a vortex that efficiently mixes the solution. This is particularly important when dissolving solids or mixing solutions of different densities.
Analytical Balance: Weighing with Precision
An analytical balance is crucial for accurately weighing the chemical reagents needed to prepare the buffer. These balances offer a high degree of precision, often to the milligram or even microgram level, ensuring that the correct molar ratios are achieved.
Always use appropriate weighing paper or boats to avoid contaminating the balance and to ensure that all of the chemical reagent is transferred to the solution. Calibration of the balance is essential for accurate measurements.
Video: Phosphate Buffer System: A Beginner's Guide
Frequently Asked Questions
Why is the phosphate buffer system important in biological systems?
The phosphate buffer system is crucial because it helps maintain a stable pH within cells. It operates effectively near physiological pH, protecting sensitive biochemical processes from harmful pH fluctuations. This makes it essential for proper cellular function.
What are the key components of the phosphate buffer system?
The primary components are dihydrogen phosphate (H2PO4-) and hydrogen phosphate (HPO42-). H2PO4- acts as a weak acid, donating protons (H+) when the pH is too high. HPO42- acts as a weak base, accepting protons when the pH is too low. Together, they maintain equilibrium in the phosphate buffer system.
How does the phosphate buffer system work in maintaining pH?
The phosphate buffer system maintains pH by reacting with either excess acid (H+) or excess base (OH-) in a solution. If the solution is too acidic, HPO42- accepts H+ to form H2PO4-. If the solution is too basic, H2PO4- releases H+ to form HPO42-, thus resisting changes in pH.
Is the phosphate buffer system more important inside or outside of cells?
The phosphate buffer system is particularly important inside cells. This is because phosphate concentrations are generally higher intracellularly than extracellularly. Its buffering capacity is more significant within the cellular environment, helping maintain optimal pH for enzyme activity and other cellular processes.
So, that's the phosphate buffer system in a nutshell! Hopefully, this guide has demystified it a bit. While it might seem complex at first, understanding the basics can really help you appreciate how crucial it is in maintaining stable pH levels, both in the lab and in our own bodies. Now you've got a foundational understanding of the phosphate buffer system – go forth and buffer!