Nucleus Drawing: Realistic Guide for Biology Students
The intricate structure of a cell nucleus, often explored through the creation of a nucleus drawing, is a fundamental concept in biology education. Students at institutions like Khan Academy frequently encounter simplified diagrams of cellular components. However, generating a realistic nucleus drawing necessitates understanding the organelle's complex features. Enhanced by tools like specialized microscopy, a detailed nucleus drawing can accurately represent structures such as chromatin and nucleoli, crucial for grasping gene expression. Examining the work of pioneers, such as Walther Flemming, who meticulously observed and documented nuclear division, provides a historical perspective that enriches a student's understanding of the nucleus drawing process.
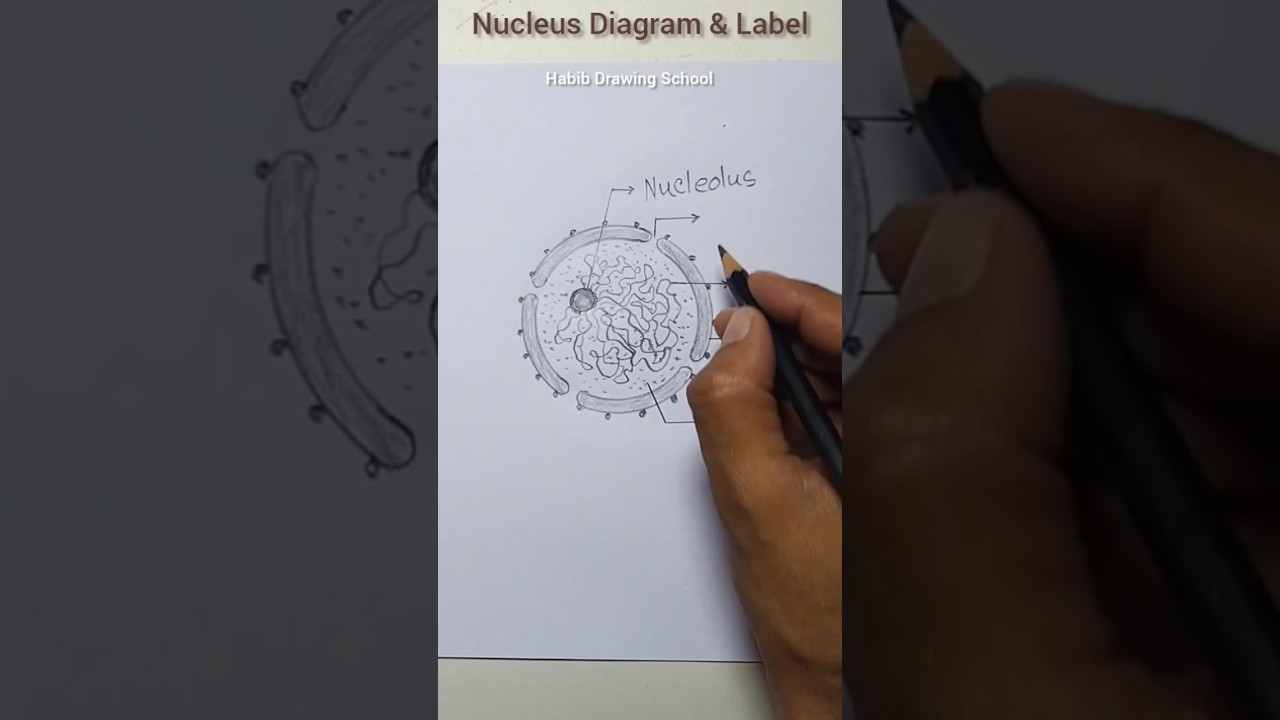
Image taken from the YouTube channel Habib Drawing School , from the video titled Nucleus Diagram & Label #shorts #nucleusdiagram #nucleus .
Unveiling the Eukaryotic Cell Nucleus: The Command Center of Life
The study of cells, known as cell biology, forms the cornerstone of our understanding of life. It delves into the intricate world of cellular structures and functions, unraveling the mechanisms that govern life processes. From metabolism to heredity, cell biology provides the fundamental insights necessary to comprehend the complexity of living organisms. Understanding the inner workings of cells allows scientists to develop treatments for diseases and enhance agricultural productivity.
Prokaryotic vs. Eukaryotic: A Defining Distinction
One of the most fundamental distinctions in biology is the classification of cells into two major types: prokaryotic and eukaryotic. Prokaryotic cells, such as bacteria and archaea, are characterized by their simple structure. They lack a membrane-bound nucleus.
In contrast, eukaryotic cells, found in plants, animals, fungi, and protists, possess a defining feature: the nucleus. The nucleus is a membrane-enclosed organelle. It houses the cell's genetic material, DNA.
This structural difference has profound implications for the organization and regulation of cellular processes. The presence of a nucleus allows for greater complexity and control over gene expression and other cellular functions.
The Nucleus: A Hub of Activity
The nucleus is far more than just a container for DNA. It is a dynamic and highly organized structure that plays a central role in cellular function. The nucleus dictates cellular behavior by regulating gene expression. It also ensures the accurate replication and repair of DNA.
Understanding the structure and function of the nucleus is crucial for comprehending overall cellular behavior. This understanding extends to genetic regulation.
By studying the nucleus, we gain insights into the mechanisms that govern cell growth, differentiation, and response to environmental cues. Exploring the nucleus helps us unravel the complexities of life itself.
The Nuclear Envelope: Guardian of the Genome
Having established the importance of the eukaryotic cell nucleus, we now turn our attention to its outermost boundary: the nuclear envelope. This intricate structure is far more than a simple membrane; it's a highly regulated barrier, a gatekeeper, and a critical player in the orchestration of cellular life.
The Double-Membrane Structure
The nuclear envelope is a double-membraned structure that encases the nucleus, effectively separating the genetic material and associated processes from the bustling cytoplasm.
This unique arrangement consists of two lipid bilayer membranes: the inner nuclear membrane (INM) and the outer nuclear membrane (ONM).
The space between these two membranes is known as the perinuclear space, which is continuous with the endoplasmic reticulum (ER) lumen, highlighting the structural and functional connection between these two organelles.
Separating Nuclear Contents
One of the primary functions of the nuclear envelope is to maintain a distinct nuclear environment.
By segregating the DNA, RNA, and other nuclear components from the cytoplasm, the nuclear envelope ensures that critical processes such as DNA replication, transcription, and RNA processing can occur with precision and efficiency.
This separation is essential for protecting the genetic material from damage and interference, while also allowing for the specific regulation of gene expression.
Maintaining a Distinct Nuclear Environment
The selective permeability of the nuclear envelope is crucial for maintaining the unique biochemical composition of the nucleus.
This is achieved through nuclear pore complexes (NPCs), which are large protein structures embedded within the nuclear envelope, acting as gateways for the regulated transport of molecules in and out of the nucleus.
These NPCs control the passage of proteins, RNA, and other essential molecules, ensuring that the nuclear environment remains optimized for its specific functions.
Regulating Gene Expression and Nuclear Processes
The nuclear envelope plays a critical role in regulating gene expression and other essential nuclear processes.
By controlling the access of transcription factors and other regulatory proteins to the DNA, the nuclear envelope influences which genes are turned on or off at any given time.
This regulation is essential for cellular differentiation, development, and responses to environmental stimuli.
Furthermore, the nuclear envelope is involved in organizing the chromatin within the nucleus, which can also impact gene expression.
Implications for Cellular Health and Disease
The integrity and proper functioning of the nuclear envelope are essential for maintaining cellular health.
Dysfunction of the nuclear envelope has been implicated in a variety of diseases, including cancer, aging-related disorders, and muscular dystrophies.
Mutations in genes encoding nuclear envelope proteins, such as lamins, can disrupt the structure and function of the nuclear envelope, leading to a range of cellular abnormalities.
Understanding the intricate details of the nuclear envelope and its role in maintaining genomic stability and regulating gene expression is therefore crucial for developing new strategies for preventing and treating these diseases.
Nuclear Pores: Gateways for Molecular Traffic
Having examined the nuclear envelope's role as a barrier, it's crucial to understand how the nucleus communicates with the cytoplasm. This communication occurs through nuclear pores, intricate protein complexes embedded within the nuclear envelope, acting as highly regulated gateways for molecular traffic. These pores are not merely holes; they are sophisticated machines that control the passage of molecules into and out of the nucleus, ensuring the proper functioning of the cell.
Structure of the Nuclear Pore Complex (NPC)
The nuclear pore complex (NPC) is a massive structure, one of the largest protein complexes found in eukaryotic cells. Its intricate architecture reflects its critical role in regulating nucleocytoplasmic transport.
The NPC is composed of approximately 30 different proteins, known as nucleoporins (Nups). These Nups are arranged in an octagonal symmetry around a central channel.
This octagonal framework anchors the NPC within the nuclear envelope, spanning both the inner and outer nuclear membranes. The central channel provides the pathway for molecules to traverse the nuclear envelope, but this pathway is not an open door.
Instead, the central channel is lined with FG-Nups, a subset of nucleoporins containing phenylalanine-glycine repeats. These FG-repeats create a hydrophobic sieve-like structure that restricts the free diffusion of large molecules, ensuring that only specifically targeted molecules are transported.
Regulated Transport: The Key to Nuclear Function
The primary function of nuclear pores is to regulate the transport of molecules between the nucleus and the cytoplasm. This regulation is essential for maintaining the unique biochemical environment of the nucleus and for controlling gene expression.
Small molecules and ions can passively diffuse through the NPC, but the transport of larger molecules, such as proteins and RNA, requires active transport mechanisms. This active transport is mediated by nuclear transport receptors (NTRs), also known as karyopherins.
NTRs recognize specific nuclear localization signals (NLS) on proteins destined for the nucleus, or nuclear export signals (NES) on molecules being exported from the nucleus.
The NTRs then interact with the FG-Nups lining the central channel of the NPC, facilitating the movement of the cargo molecule through the pore. This process is fueled by the Ran GTPase cycle, which provides the energy and directionality for transport.
Molecular Cargo: Examples and Importance
The nuclear pores facilitate the transport of a wide variety of molecules, each playing a critical role in cellular function. Understanding these cargo molecules highlights the importance of regulated transport.
Messenger RNA (mRNA), carrying genetic information from DNA to ribosomes for protein synthesis, must be exported from the nucleus to the cytoplasm. This export is tightly regulated to ensure that only mature, correctly processed mRNA molecules are translated.
Proteins, including transcription factors, DNA repair enzymes, and histones, are imported into the nucleus to carry out their functions in gene expression, genome maintenance, and chromatin organization.
Ribosomal subunits, assembled in the nucleolus, are exported to the cytoplasm where they combine to form functional ribosomes. This export is essential for protein synthesis.
The import of histones and DNA polymerases is critical for DNA replication and chromosome maintenance. These molecules must enter the nucleus to participate in these essential functions.
Dysregulation of nuclear transport has been implicated in various diseases, including cancer and viral infections. Therefore, understanding the mechanisms of nuclear pore function is crucial for developing new therapeutic strategies.
Nucleoplasm: The Nuclear Matrix
Having navigated the nuclear envelope and its pore complexes, we now delve into the nucleus's interior, a realm defined by the nucleoplasm. This seemingly simple gel-like matrix is far more than just filler; it is a dynamic environment essential for nuclear function. Understanding its composition and multifaceted roles is critical to appreciating the overall complexity of the eukaryotic cell.
Composition and Characteristics of the Nucleoplasm
The nucleoplasm, also known as nuclear hyaloplasm, occupies the space between the nuclear envelope and the chromosomes. Imagine a cell's command center filled with a specialized liquid environment where crucial molecular events transpire.
Its primary component is water, providing the necessary solvent for biochemical reactions. Dissolved within are a variety of ions, such as potassium, sodium, and chloride, maintaining the proper ionic balance essential for enzyme activity and DNA stability.
Critically, the nucleoplasm is teeming with enzymes. These enzymes are vital for DNA replication, RNA transcription, and various other metabolic processes exclusive to the nucleus. These biological catalysts enable the numerous processes that keep the cell functioning and help transcribe genes.
Beyond water, ions, and enzymes, the nucleoplasm contains a diverse array of other molecules. This includes structural proteins involved in maintaining nuclear architecture, as well as a plethora of small molecules and metabolites participating in various biochemical pathways. These metabolites include nucleotides and cofactors necessary for enzymatic activities and DNA/RNA synthesis.
Structural Support and Organization
The nucleoplasm is not a homogenous solution; instead, it exhibits complex organization and provides structural support to the nuclear components. It interacts with the nuclear lamina, the inner lining of the nuclear envelope, anchoring it in place.
This interaction helps to maintain the nucleus's shape and integrity. The nucleoplasm also houses various protein networks and sub-compartments that contribute to organizing the genome and other nuclear constituents.
These networks can influence how DNA is organized in the nucleus, as well as the processes that occur within it. It's through these networks that specific chromosomes are arranged in a particular manner, giving rise to organized chromosomes.
Biochemical Reactions and Molecular Transport
The nucleoplasm serves as the medium for a multitude of biochemical reactions essential for cell survival. DNA replication and repair occur within this environment, facilitated by enzymes and other factors present in the nucleoplasm. Transcription, the process of synthesizing RNA from DNA, also takes place within this gel-like matrix.
The nucleoplasm also facilitates molecular transport within the nucleus and between the nucleus and the cytoplasm. Molecules such as proteins, RNA, and other macromolecules must be transported efficiently through the nucleoplasm to reach their destinations.
This transport is mediated by various mechanisms, including diffusion and active transport, ensuring that molecules are delivered to the correct locations within the nucleus or exported to the cytoplasm as needed. The efficient and regulated transport of molecules is critical for maintaining cellular homeostasis and responding to environmental cues.
Chromatin and Chromosomes: Packaging the Genetic Code
Following the exploration of the nucleoplasm, we now turn our attention to the very essence of the nucleus: its genetic material. The organization of DNA within this compartment is not a haphazard affair, but rather a highly orchestrated process involving the formation of chromatin and, ultimately, chromosomes. Understanding this packaging is crucial to grasping the complex interplay between genetic information and cellular function.
The Chromatin Architecture: DNA's Intricate Housing
Within the eukaryotic nucleus, DNA does not exist as a naked, linear molecule. Instead, it is meticulously organized into a complex structure called chromatin.
Chromatin is a dynamic assembly of DNA and proteins, primarily histones, that acts as the fundamental unit of DNA packaging. This packaging is essential for accommodating the vast length of the genome within the limited confines of the nucleus, preventing DNA damage, and regulating gene expression.
Histones: The Architects of DNA Compaction
Histones are a family of small, positively charged proteins that play a central role in chromatin structure. Their positive charge facilitates strong binding to the negatively charged DNA backbone.
The primary structural unit of chromatin is the nucleosome, which consists of approximately 147 base pairs of DNA wrapped around a core of eight histone proteins (two each of H2A, H2B, H3, and H4).
This "beads-on-a-string" arrangement further coils and folds into higher-order structures, achieving significant DNA compaction. The histone H1 protein assists in stabilizing these higher-order structures.
The arrangement of histones protects DNA from degradation and allows for efficient compaction to fit within the nucleus.
Euchromatin vs. Heterochromatin: Accessibility and Gene Expression
Chromatin exists in two primary states: euchromatin and heterochromatin, each characterized by distinct levels of compaction and transcriptional activity.
Euchromatin is loosely packed, allowing for access by transcription factors and other regulatory proteins. This open configuration facilitates gene expression, making euchromatin regions transcriptionally active.
Conversely, heterochromatin is densely packed, restricting access to DNA. This compact state typically silences gene expression, rendering heterochromatin regions transcriptionally inactive.
The dynamic interconversion between euchromatin and heterochromatin is a crucial mechanism for regulating gene expression in response to developmental cues and environmental signals.
Chromosome Formation: Preparing for Cell Division
During cell division, chromatin undergoes a dramatic transformation, condensing even further into visible chromosomes.
This condensation is essential for ensuring accurate segregation of the duplicated genome to daughter cells.
Each chromosome consists of two identical sister chromatids, joined at the centromere. The highly condensed structure of chromosomes provides the structural integrity needed to withstand the forces of cell division.
The organized segregation of chromosomes is crucial for maintaining genetic stability and preventing aneuploidy, a condition characterized by an abnormal number of chromosomes.
The Nucleolus: Ribosome Factory
[Chromatin and Chromosomes: Packaging the Genetic Code Following the intricate organization of DNA into chromatin and chromosomes, our journey into the nucleus leads us to a specialized structure with a singular, vital purpose. This structure, the nucleolus, is not bound by a membrane, yet it orchestrates one of the most fundamental processes in the cell: ribosome biogenesis.]
The nucleolus stands out as a distinct region within the nucleus.
It's a dynamic structure whose primary function is the production of ribosomes.
These molecular machines are essential for protein synthesis.
Without ribosomes, cells could not translate genetic information into functional proteins, making the nucleolus indispensable for life.
Composition of the Ribosome Factory
The nucleolus is not a uniform structure, but rather a complex assembly of several components.
Its composition reflects its function, encompassing the essential ingredients for ribosome construction.
These elements include ribosomal RNA (rRNA) genes, pre-rRNA molecules, and ribosomal proteins.
Ribosomal RNA (rRNA) Genes
The foundation of ribosome biogenesis lies in the rRNA genes.
These genes serve as the templates for transcribing ribosomal RNA.
Multiple copies of these genes are clustered within the nucleolus.
This arrangement facilitates the efficient production of rRNA needed for ribosome assembly.
Pre-rRNA Molecules
The rRNA genes are transcribed into large precursor molecules known as pre-rRNA.
These pre-rRNA molecules undergo extensive processing and modification within the nucleolus.
This maturation process involves cleavage, trimming, and chemical alterations to produce the mature rRNA molecules.
These mature rRNA molecules are critical components of functional ribosomes.
Ribosomal Proteins
Alongside rRNA, ribosomes also require ribosomal proteins.
These proteins are synthesized in the cytoplasm and then imported into the nucleolus.
Within the nucleolus, ribosomal proteins associate with the pre-rRNA molecules.
This association initiates the assembly of the ribosomal subunits.
The Orchestrated Process of Ribosome Assembly
Ribosome biogenesis is a highly coordinated and intricate process.
This process involves numerous steps, enzymes, and regulatory factors within the nucleolus.
The main events include rRNA transcription, pre-rRNA processing, and the association of rRNA with ribosomal proteins.
rRNA Transcription
The process begins with the transcription of rRNA genes by RNA polymerase I.
This enzyme specifically synthesizes the large pre-rRNA transcript.
The high demand for ribosomes necessitates rapid and efficient transcription of these genes.
Pre-rRNA Processing
The pre-rRNA transcript undergoes a series of processing steps.
These steps are crucial for generating the mature rRNA molecules.
Enzymes such as ribonucleases cleave the pre-rRNA into smaller fragments, which eventually become the mature rRNAs.
Chemical modifications, such as methylation, also occur to fine-tune the rRNA structure and function.
Ribosomal Subunit Assembly
The processed rRNA molecules then associate with ribosomal proteins.
This association leads to the assembly of the small and large ribosomal subunits.
These subunits are not yet fully functional ribosomes.
They require further maturation and transport to the cytoplasm.
The assembly process is tightly regulated to ensure that only properly formed subunits are exported.
Export to the Cytoplasm
Once the ribosomal subunits are assembled and mature, they are transported through the nuclear pores to the cytoplasm.
In the cytoplasm, the small and large subunits combine with mRNA and tRNA.
This combination initiates the process of protein synthesis.
The nucleolus, therefore, acts as a central hub for ribosome biogenesis, ensuring the cell has a sufficient supply of these essential molecular machines.
Its structure and function are tightly linked to the fundamental process of protein synthesis, highlighting its critical role in cellular life.
Nuclear Lamina: Maintaining Nuclear Integrity
Following the intricate packaging of the genetic material, it is equally critical to consider the structures responsible for maintaining the nucleus' structural integrity and functional organization. The nuclear lamina, a meshwork of protein filaments, plays a pivotal role in these processes, acting as a crucial interface between the nuclear envelope and the chromatin within.
The Structure of the Nuclear Lamina
The nuclear lamina is a proteinaceous layer found just beneath the inner nuclear membrane. It is primarily composed of intermediate filament proteins called lamins.
These lamins assemble into higher-order structures that provide mechanical support to the nucleus. The lamina is not static; it undergoes dynamic changes during the cell cycle, particularly during mitosis.
Functions of the Nuclear Lamina
Structural Support and Nuclear Shape
One of the primary functions of the nuclear lamina is to provide structural support to the nucleus. By associating with the inner nuclear membrane and chromatin, the lamina helps maintain the shape of the nucleus and resist mechanical stress.
This is particularly important in cells that experience significant physical forces.
Chromatin Organization
The nuclear lamina plays a role in organizing chromatin within the nucleus. Specific lamin isoforms interact with chromatin, influencing its spatial arrangement and gene expression patterns.
This interaction is thought to contribute to the compartmentalization of the genome, with heterochromatin often found associated with the lamina.
Involvement in DNA Replication
The lamina's involvement in DNA replication is a critical but less overtly discussed aspect of its function.
It contributes to the anchoring and organization of replication factories, which are clustered regions where DNA replication occurs. This ensures efficient and coordinated replication of the genome.
Role in Cell Division
The nuclear lamina undergoes dramatic reorganization during cell division. Lamins are phosphorylated, leading to the disassembly of the lamina and the breakdown of the nuclear envelope.
This is a necessary step for chromosome segregation. After cell division, the lamina reassembles, and the nuclear envelope reforms around the separated chromosomes.
Nuclear Pore Complex Organization
The nuclear lamina also plays a role in the organization of nuclear pore complexes (NPCs), the gateways for molecular traffic in and out of the nucleus.
Lamins interact with NPC components, helping to anchor and position them within the nuclear envelope. This ensures proper distribution and function of NPCs.
Clinical Significance
Mutations in lamin genes have been linked to a variety of human diseases, collectively known as laminopathies. These disorders can affect a wide range of tissues, including muscle, bone, and heart.
The clinical manifestations of laminopathies highlight the critical importance of the nuclear lamina in maintaining cellular function and tissue integrity.
DNA Replication and Repair: Preserving the Genetic Blueprint
Following the intricate packaging of the genetic material, it is equally critical to consider the mechanisms that safeguard the fidelity of this information. DNA replication, the process of genome duplication, and DNA repair, which corrects errors and damage, are fundamental to cellular health and survival. Both processes occur within the nucleus and are essential for maintaining genomic integrity.
DNA Replication: A High-Fidelity Duplication Process
DNA replication is a complex process that ensures the accurate duplication of the genome before cell division. This process relies on a suite of enzymes and proteins that work in a coordinated manner.
The entire process begins with the unwinding of the DNA double helix, creating a replication fork. DNA polymerase, the primary enzyme involved, then synthesizes new DNA strands using the existing strands as templates.
The Role of DNA Polymerase
DNA polymerase is not only responsible for adding nucleotides to the growing DNA strand but also possesses a proofreading function. This proofreading ability allows it to correct errors during replication, significantly reducing the mutation rate.
Despite this proofreading, errors can still occur. The overall fidelity of DNA replication is remarkably high, with error rates of approximately one mistake per billion base pairs.
DNA Repair Mechanisms: Correcting Errors and Damage
Despite the high fidelity of DNA replication, DNA is constantly subjected to damage from various sources, including:
- Environmental factors
- Metabolic byproducts
- Replication errors
To combat this, cells have evolved a variety of DNA repair mechanisms.
Types of DNA Repair Pathways
Several distinct DNA repair pathways address different types of DNA damage:
- Mismatch Repair (MMR): Corrects base-pairing errors that escape proofreading during replication.
- Base Excision Repair (BER): Removes damaged or modified single bases.
- Nucleotide Excision Repair (NER): Repairs bulky DNA lesions, such as those caused by UV radiation.
- Homologous Recombination (HR): Repairs double-strand breaks using a homologous DNA template.
- Non-Homologous End Joining (NHEJ): Repairs double-strand breaks by directly joining the broken ends, although this can sometimes introduce errors.
Consequences of Defective DNA Repair
Defects in DNA repair mechanisms can have profound consequences, including:
- Increased mutation rates
- Genomic instability
- Increased risk of cancer
Many hereditary cancer syndromes are linked to mutations in genes involved in DNA repair pathways, highlighting the critical role of these pathways in preventing tumorigenesis.
In conclusion, DNA replication and repair are indispensable processes that ensure the accurate transmission of genetic information from one generation to the next. These processes safeguard against mutations and maintain genomic stability, underscoring their importance for cellular function and organismal health.
Transcription: From DNA to RNA
[DNA Replication and Repair: Preserving the Genetic Blueprint Following the intricate packaging of the genetic material, it is equally critical to consider the mechanisms that safeguard the fidelity of this information. DNA replication, the process of genome duplication, and DNA repair, which corrects errors and damage, are fundamental to cellular health. This next stage describes how DNA serves as a template for RNA.]
Transcription is a pivotal process within the nucleus, serving as the initial step in gene expression. It involves the synthesis of RNA molecules from DNA templates.
This intricate procedure dictates which genes are activated and when, influencing cellular function and development. It is vital for translating genetic information into functional molecules.
The Transcription Process
At its core, transcription involves using a strand of DNA as a template to create a complementary RNA molecule. This process is orchestrated by RNA polymerase, an enzyme crucial for reading the DNA sequence.
The stages of transcription can be broadly classified into:
- Initiation
- Elongation
- Termination
The DNA template unwinds, and RNA polymerase begins synthesizing an RNA strand that is complementary to the template DNA strand during initiation. Elongation involves the continuous addition of RNA nucleotides to the growing RNA molecule.
This process continues until RNA polymerase reaches a termination signal, prompting the release of the newly synthesized RNA and the enzyme from the DNA template. It's important to ensure proper RNA creation for all cells in the body.
Role of RNA Polymerase and Transcription Factors
RNA polymerase is the central enzyme, but its functionality is intricately linked with transcription factors. These proteins bind to specific DNA sequences.
They regulate the polymerase activity and help initiate transcription at specific gene locations.
These factors help RNA polymerase bind to the promoter region of a gene, effectively turning the gene on or off.
The interplay of RNA polymerase and transcription factors ensures precise regulation of gene expression, tailoring cellular responses to varying stimuli. These stimuli directly affect the synthesis of protein creation.
Types of RNA and Their Functions
Transcription produces several types of RNA, each with a unique role in protein synthesis:
- Messenger RNA (mRNA) serves as the template for protein synthesis.
- Transfer RNA (tRNA) is responsible for carrying amino acids to the ribosome.
- Ribosomal RNA (rRNA) forms an integral part of the ribosome itself.
Each type of RNA plays a specific part. mRNA is the blueprint. tRNA brings building blocks. rRNA constitutes the factory where proteins are assembled.
The coordinated function of these RNA types ensures accurate and efficient protein production.
Ribosome Biogenesis: Building the Protein Synthesis Machinery
Following the intricate packaging of the genetic material and the mechanisms ensuring its fidelity, it is now essential to examine the creation of the cellular machinery that translates this genetic information into functional proteins. Ribosome biogenesis, a complex and highly regulated process, is the creation of ribosomes, the protein synthesis factories of the cell. This process, primarily occurring within the nucleolus, involves a coordinated series of events, starting with rRNA gene transcription and culminating in the export of mature ribosomes to the cytoplasm.
rRNA Transcription: The Foundation of Ribosome Assembly
The genesis of a ribosome begins with the transcription of ribosomal RNA (rRNA) genes. Eukaryotic cells possess multiple copies of these genes, organized in tandem repeats within the nucleolus.
RNA polymerase I (Pol I) is the dedicated enzyme responsible for transcribing the large pre-rRNA precursor, a single transcript encoding the 18S, 5.8S, and 28S rRNA molecules. This initial transcription event is the rate-limiting step in ribosome biogenesis, highlighting the importance of regulating Pol I activity.
The efficiency of rRNA transcription is tightly controlled in response to cellular growth signals and nutrient availability, ensuring that ribosome production is matched to the cell's protein synthesis needs. Disruptions in rRNA transcription can have profound consequences on cell growth and proliferation.
Processing and Modification of Pre-rRNA
The initial pre-rRNA transcript undergoes extensive processing and modification to generate the mature rRNA molecules. This intricate process involves a cascade of enzymatic reactions, including:
- Cleavage: Endonucleases and exonucleases cleave the pre-rRNA at specific sites, releasing the individual rRNA molecules.
- Modification: Ribose methylation and pseudouridylation occur at specific nucleotides within the rRNA, guided by small nucleolar RNAs (snoRNAs).
These modifications are crucial for proper ribosome structure and function. snoRNAs act as guide RNAs, directing the modifying enzymes to their target sites on the pre-rRNA. The accurate processing and modification of pre-rRNA are essential for ribosome assembly and activity.
Ribosomal Protein Association and Ribosome Assembly
Simultaneous with rRNA processing, ribosomal proteins (r-proteins) are imported from the cytoplasm into the nucleolus. These r-proteins, synthesized in the cytoplasm, must be chaperoned through the nuclear pore complexes and then assemble onto the pre-rRNA molecules in a specific order.
The association of r-proteins with pre-rRNA is a highly regulated process, involving numerous assembly factors that act as chaperones, preventing premature folding and aggregation. This ordered assembly ensures the correct stoichiometry and spatial arrangement of ribosomal components.
As the assembly progresses, the pre-ribosomal particles undergo conformational changes, gradually maturing into the 40S (small subunit) and 60S (large subunit) ribosomal subunits.
Export of Mature Ribosomes to the Cytoplasm
The final step in ribosome biogenesis is the export of the mature 40S and 60S ribosomal subunits from the nucleus to the cytoplasm. This export process is mediated by specific transport factors that recognize and bind to the mature ribosomal subunits, escorting them through the nuclear pore complexes.
The export of ribosomes is a highly regulated process, ensuring that only fully assembled and functional ribosomes are released into the cytoplasm. Quality control mechanisms exist to prevent the export of defective ribosomes, protecting the cell from synthesizing aberrant proteins.
Once in the cytoplasm, the 40S and 60S subunits associate with mRNA and tRNA molecules to initiate protein synthesis. The coordinated events of ribosome biogenesis within the nucleolus are essential for maintaining cellular homeostasis and protein synthesis capacity. Dysregulation of ribosome biogenesis can lead to various diseases, including cancer and developmental disorders.
Microscopy: Visualizing the Invisible
Following the examination of ribosome biogenesis and the intricate processes within the cell nucleus, it becomes imperative to delve into the techniques that allow us to visualize these minute structures and processes. Microscopy stands as an indispensable tool, bridging the gap between theoretical understanding and empirical observation in cell biology.
Microscopy is the science of visualizing small objects and structures that are not visible to the naked eye. It employs various instruments and techniques to magnify and resolve minute details, providing invaluable insights into the intricacies of biological systems.
For the study of the nucleus, microscopy is not merely a tool, but a necessity. It is through these visual windows that we can discern the complex organization of chromatin, the dynamic movements within the nucleoplasm, and the intricate architecture of the nuclear envelope.
Light Microscopy: A Foundation for Cellular Observation
Light microscopy, also known as optical microscopy, utilizes visible light and a system of lenses to magnify images of small samples. This technique is fundamental to cell biology, allowing researchers to observe cells, tissues, and cellular components with relative ease.
Principles of Light Microscopy
The basic principle involves passing light through a thin specimen, which is then magnified by a series of lenses. Key factors influencing image quality include:
- Magnification: The extent to which the image is enlarged.
- Resolution: The ability to distinguish between two closely spaced objects.
- Contrast: The difference in light intensity between the specimen and the background.
Applications in Nuclear Visualization
Light microscopy can reveal the basic structure of the nucleus, including its size, shape, and location within the cell.
Different staining techniques can be employed to enhance contrast and highlight specific nuclear components, such as DNA (using DAPI) or proteins.
However, the resolution of light microscopy is limited by the wavelength of visible light, which restricts its ability to resolve structures smaller than approximately 200 nanometers.
Electron Microscopy: Unveiling the Nanoscale
To overcome the limitations of light microscopy and visualize the nucleus at a much higher resolution, electron microscopy techniques are employed. Transmission electron microscopy (TEM) and scanning electron microscopy (SEM) are two primary types, each offering unique perspectives on nuclear structure.
Transmission Electron Microscopy (TEM)
TEM involves transmitting a beam of electrons through a very thin specimen. The electrons interact with the specimen, and the transmitted electrons are used to create an image.
TEM provides exceptional resolution, allowing visualization of nuclear structures at the nanometer scale. This technique is invaluable for studying the detailed organization of chromatin, the structure of nuclear pores, and the ultrastructure of the nucleolus.
However, TEM requires extensive sample preparation, including fixation, embedding, and sectioning, which can potentially introduce artifacts.
Scanning Electron Microscopy (SEM)
SEM, on the other hand, scans a focused beam of electrons across the surface of a specimen. The electrons interact with the surface atoms, producing signals that are used to create an image.
SEM provides high-resolution images of the surface topography of the nucleus. This technique is useful for studying the three-dimensional architecture of the nuclear envelope and the distribution of nuclear pores.
While SEM offers excellent surface detail, it generally has lower resolution than TEM and requires samples to be coated with a conductive material.
TEM vs. SEM: A Comparative Overview
Feature | Transmission Electron Microscopy (TEM) | Scanning Electron Microscopy (SEM) |
---|---|---|
Electron Beam | Transmitted through the specimen | Scanned across the surface |
Image Type | Two-dimensional projection | Three-dimensional surface image |
Resolution | Higher | Lower |
Sample Prep | Extensive, thin sections required | Coating with conductive material |
Information Gained | Internal ultrastructure | Surface topography |
The Significance of Magnification and Scale Bars
Regardless of the microscopy technique used, accurate interpretation of microscopic images requires careful attention to magnification and scale bars. Magnification indicates the degree to which the image is enlarged compared to the actual size of the specimen.
Scale bars, which are typically included in microscopic images, provide a visual reference for determining the actual size of structures within the image.
Both magnification and scale bars are essential for quantifying measurements and comparing images across different studies.
By understanding the principles and applications of various microscopy techniques, researchers can unlock the secrets hidden within the nucleus, advancing our knowledge of cell biology and genetics.
Scientific Illustration: Communicating Visual Information
Following the examination of ribosome biogenesis and the intricate processes within the cell nucleus, it becomes imperative to delve into the techniques that allow us to visualize these minute structures and processes. Scientific illustration serves as a critical bridge, translating complex data into accessible visual representations.
The nucleus, with its intricate architecture and dynamic processes, presents a unique challenge and opportunity for illustrators. Accurate and informative drawings are paramount, conveying the nucleus's complex anatomy and function with clarity and precision. These illustrations are vital for educational resources, research publications, and public understanding of cellular biology.
The Enduring Value of Traditional Techniques
Traditional illustration methods, though seemingly antiquated in the digital age, remain invaluable for developing a deep understanding of form and structure. Tools like pencils, erasers, rulers, and compasses provide a tactile connection to the subject matter, fostering a nuanced appreciation for detail and accuracy.
Mastering Pencil Grades and Shading
The strategic use of various pencil grades is essential for rendering different textures and structural details. Harder pencils (e.g., 2H-4H) are ideal for creating fine lines and precise outlines, while softer pencils (e.g., 2B-6B) allow for rich, dark tones and expressive shading.
Shading techniques such as stippling, hatching, and blending can effectively convey the three-dimensionality of nuclear components and differentiate between various structures within the nucleus.
Erasers: More Than Just Correction Tools
Erasers are not merely tools for correcting mistakes; they are essential for creating highlights and refining details. A kneaded eraser, in particular, can be molded into various shapes, allowing for precise removal of graphite and the creation of subtle tonal variations.
The Precision of Rulers and Compasses
Rulers and compasses are indispensable for ensuring accurate measurements and shapes, especially when depicting symmetrical structures or maintaining scale. The precise depiction of the nuclear envelope, nuclear pores, and chromosome morphology relies heavily on these tools.
Embracing the Power of Digital Illustration
Digital illustration tools offer a powerful alternative to traditional methods, providing enhanced flexibility, efficiency, and the ability to create highly polished visuals. Labeling software and specialized drawing programs are essential components of the modern scientific illustrator's toolkit.
The Clarity of Specialized Labeling Software
Clear and concise annotations are crucial for conveying information effectively in scientific diagrams. Specialized labeling software allows for the creation of precise labels, leaders, and callouts that seamlessly integrate with the illustration. These tools also ensure consistent font styles, sizes, and placement, enhancing the overall clarity and professionalism of the visual.
Digital Drawing Programs: Precision and Versatility
Drawing software such as Adobe Illustrator and Inkscape offer a vast array of tools and features for creating professional-quality scientific illustrations. Vector-based graphics allow for scalable images without loss of resolution, ensuring that illustrations remain sharp and clear across various media.
These programs also provide precise control over color, line weight, and other visual elements, enabling illustrators to create highly detailed and visually compelling representations of the nucleus.
The Importance of Reference Images
Regardless of whether traditional or digital techniques are employed, the use of reference images, particularly micrographs, is paramount for ensuring accuracy in scientific illustrations. Micrographs provide visual evidence of the nucleus's structure and should serve as the foundation for any illustration.
Careful observation and interpretation of micrographs are essential for capturing the subtle details and complexities of the nucleus, transforming raw data into informative and visually engaging representations.
The Role of Professionals in Visualizing the Nucleus
Following the examination of scientific illustration and its techniques, it is crucial to understand the roles of various professionals who contribute to and utilize visual representations of the nucleus. From scientific illustrators crafting detailed diagrams to educators employing these visuals in classrooms, a diverse group of experts relies on accurate depictions of this essential cellular component.
Scientific Illustrators: Accuracy in Visual Representation
Scientific illustrators are specialized artists who possess both artistic skill and a deep understanding of scientific concepts. Their expertise lies in creating visual representations of complex structures like the nucleus, ensuring accuracy and clarity.
-
They translate intricate scientific data into accessible images, making them invaluable for researchers, educators, and the public. They meticulously study micrographs and scientific literature to produce illustrations that accurately reflect the nucleus's structure and function.
Their work often involves creating detailed diagrams showing the various components of the nucleus, such as the nuclear envelope, chromatin, and nucleolus.
-
The use of specific colors and shading techniques helps to highlight the different features and their relationships to one another.
This level of detail demands a strong foundation in both biology and art.
Biology Educators: Visual Aids for Effective Teaching
Biology educators play a vital role in conveying the complexities of cell biology to students. Visual aids, including illustrations of the nucleus, are essential tools in their teaching arsenal.
-
These visuals help students grasp abstract concepts and visualize structures that are otherwise invisible to the naked eye. A well-crafted diagram of the nucleus can significantly enhance student understanding of its components and functions.
Teachers utilize these illustrations in lectures, presentations, and textbooks.
- By incorporating visuals into their teaching, educators can cater to different learning styles and make the subject matter more engaging.
Cell Biologists: Visual Data in Research and Discovery
Cell biologists are researchers who dedicate their careers to studying the intricacies of cells, including the nucleus. Visual representations are indispensable in their research, allowing them to analyze and interpret experimental data.
- Microscopy images, in particular, provide direct visual evidence of nuclear structures and processes.
-
Cell biologists often generate their own illustrations to communicate their findings in scientific publications and presentations. These visuals serve as a critical component of their research, enabling them to share their discoveries with the broader scientific community.
The ability to accurately depict the nucleus and its associated processes is fundamental to their work.
Textbook Authors: Compiling Visual Information
Authors of biology textbooks play a crucial role in synthesizing and presenting complex scientific information in an accessible format.
-
They rely heavily on diagrams and illustrations to explain the structure and function of the nucleus. Textbook authors work closely with scientific illustrators to ensure that the visuals used in their books are accurate, clear, and informative.
These visuals act as a critical component in helping students learn and retain information about this essential cellular component. The quality and accuracy of these illustrations significantly impact the effectiveness of the textbook as a learning tool.
- The selection of appropriate visuals and their integration into the text is a key aspect of textbook authoring.
The Nucleus in the Learning Environment
Following the examination of scientific illustration and its techniques, it is crucial to understand the roles of various professionals who contribute to and utilize visual representations of the nucleus. From scientific illustrators crafting detailed diagrams to educators employing these visuals in the classroom, a multi-faceted approach is required for its educational efficacy. The study of the nucleus transcends mere memorization of its components; it necessitates active engagement and critical thinking, especially within formal learning settings.
Drawing the Nucleus: Reinforcing Conceptual Understanding
Drawing the nucleus in biology classrooms offers a powerful method to solidify students’ understanding of its complex structure. This isn't simply an exercise in artistic skill; it's a cognitive process that demands careful observation and synthesis of information.
Active recall is a key component, as students must actively retrieve knowledge about the nucleus's components and their spatial relationships. This method contrasts with passive learning, where information is simply received without active processing.
By sketching the nuclear envelope, nuclear pores, chromatin, and nucleolus, students actively engage with the material, enhancing retention. The act of drawing forces students to think critically about the relative sizes, shapes, and positions of each component, leading to a deeper, more nuanced comprehension.
Furthermore, drawing allows students to personalize their learning experience. Each student's drawing will reflect their unique understanding and interpretation of the nuclear structure. This fosters a sense of ownership and investment in the learning process.
Biology Labs: Microscopes and Real-World Observations
Biology laboratories provide an invaluable opportunity for students to observe real cells and their nuclei under a microscope. This hands-on experience complements textbook knowledge and diagrams, creating a more holistic learning experience.
From Diagrams to Reality
Observing prepared slides of eukaryotic cells allows students to bridge the gap between abstract representations and tangible reality. This encounter can be a pivotal moment in their understanding of cell biology.
While the resolution of light microscopes may not reveal the intricate details of nuclear pores or chromatin structure, students can clearly discern the nucleus as a distinct, membrane-bound organelle within the cell. This visual confirmation reinforces the fundamental difference between eukaryotic and prokaryotic cells.
Experiential Learning: Engaging Multiple Senses
The integration of visual observations with tactile experiences, such as preparing slides or adjusting the microscope, enhances student engagement and promotes deeper learning. These opportunities offer direct validation of textbook concepts and foster curiosity.
The process of staining cells to enhance nuclear visibility further solidifies their knowledge of the nucleus’s biochemical properties. Observing stained nuclei allows for the recognition of DNA and other key components, promoting understanding of the molecule structures.
Through hands-on lab work, students develop crucial scientific skills such as observation, data collection, and analysis. These skills are not only essential for future scientists but also valuable for informed decision-making in everyday life. The hands-on lab experience, paired with drawing and illustrations, provide a rich and more easily remembered understanding of the subject.
Video: Nucleus Drawing: Realistic Guide for Biology Students
FAQs: Nucleus Drawing
Why is it important to draw a realistic nucleus?
A realistic nucleus drawing helps visualize and understand its complex structure. Accurate representation reinforces learning key components like the nucleolus and chromatin, crucial for grasping cellular functions.
What are the key components to include in a nucleus drawing?
Your nucleus drawing should include the nuclear envelope (with nuclear pores), chromatin (heterochromatin and euchromatin), and at least one nucleolus. These elements are essential for a complete and accurate depiction.
How can I make my nucleus drawing more realistic?
Focus on representing textures and shading. Use varied line weights to differentiate between the envelope and the internal structures. Showing chromatin as granular and the nucleolus as dense will increase realism in your nucleus drawing.
What's the difference between heterochromatin and euchromatin in a nucleus drawing?
Heterochromatin should appear darker and more condensed in your nucleus drawing, representing tightly packed DNA. Euchromatin should look lighter and more dispersed, indicating less condensed, actively transcribed DNA.
So, there you have it! Hopefully, this guide demystified the process and you feel a bit more confident tackling your next nucleus drawing. Remember, practice makes perfect, so grab your pencils, look at some real microscope images, and have fun bringing those cellular structures to life on paper. Good luck!