Is Nitrogen a Metal? Properties & Facts
Nitrogen, a quintessential element in the periodic table, exhibits properties that sharply contrast with those of metals, raising the fundamental question: is nitrogen a metal? The Royal Society of Chemistry characterizes nitrogen primarily as a non-metal, owing to its gaseous state at room temperature and its high electronegativity. Unlike metals such as copper or iron, nitrogen lacks the characteristic metallic luster and electrical conductivity, aligning it more closely with elements like oxygen and chlorine. The debate around whether nitrogen could exhibit metallic properties under extreme conditions, such as those studied at the University of Cambridge's high-pressure laboratories, continues to intrigue scientists.
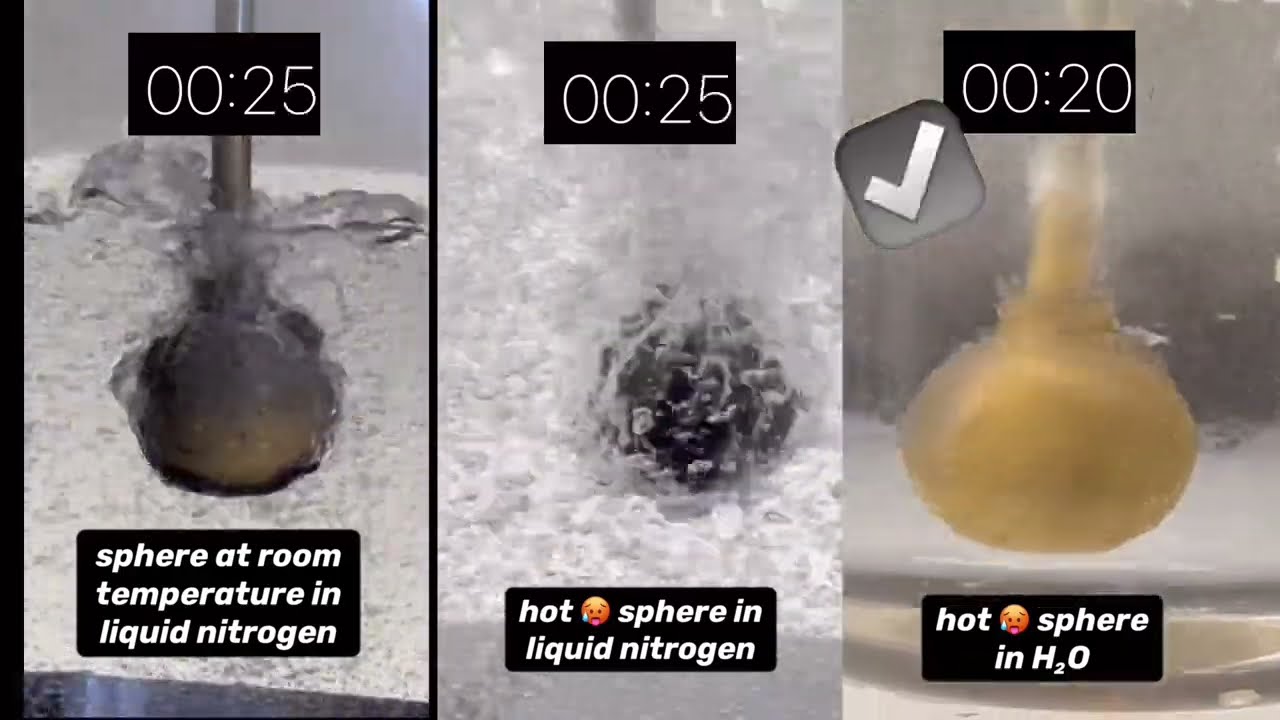
Image taken from the YouTube channel Tommy Technetium , from the video titled HOT METAL balls in liquid nitrogen and water: WHAT HAPPPENS? .
Nitrogen. The very air we breathe is dominated by this element, yet it often goes unnoticed. It’s an unsung hero, a foundational element quietly supporting life and driving countless industrial processes.
This section serves as your essential guide to nitrogen, offering a foundational understanding of its properties, definition, and far-reaching significance. Prepare to be amazed by the ubiquity and importance of this seemingly simple element.
What is Nitrogen? An Elemental Overview
Nitrogen, represented by the symbol N and possessing the atomic number 7, is a chemical element that belongs to Group 15 (the pnictogens) of the periodic table. In its most common form, it exists as a diatomic gas, N2, also known as dinitrogen.
At standard temperature and pressure, nitrogen is a colorless, odorless, and relatively inert gas. While it makes up approximately 78% of the Earth's atmosphere, its presence extends far beyond the air we breathe.
Nitrogen is a constituent of amino acids, proteins, nucleic acids (DNA and RNA), and numerous other crucial organic molecules. Without nitrogen, life as we know it would simply not exist.
The Significance of Atomic Number 7
The atomic number is not just an arbitrary label. The atomic number, 7 for Nitrogen, dictates the number of protons within the nucleus of a nitrogen atom.
This number defines nitrogen’s identity and dictates its position within the periodic table. The electronic configuration, directly linked to the atomic number, determines how nitrogen interacts with other elements, forming diverse compounds with unique properties.
The seven protons attract seven electrons, leading to nitrogen's characteristic electron configuration (1s² 2s² 2p³), which strongly influences its bonding behavior.
A Glimpse into History: Discovery and Naming
While seemingly ever-present, nitrogen wasn't always recognized as a distinct element. Its discovery and eventual naming are fascinating chapters in the history of chemistry.
We'll briefly touch upon these historical milestones, setting the stage for a deeper dive into nitrogen's past in the next section. This element has always been around, yet its discovery would mark a new era of understanding.
Unveiling Nitrogen's Past: Discovery and Nomenclature
Nitrogen, a ubiquitous element that forms the foundation of our atmosphere and the building blocks of life, wasn't always recognized for its unique identity. The story of its discovery and naming is a testament to the scientific curiosity and evolving understanding of the natural world.
This section dives into the historical context surrounding nitrogen, shedding light on the pivotal figures and circumstances that led to its recognition as a distinct element. Prepare to journey back in time and uncover the fascinating origins of this essential gas.
The Isolation of "Noxious Air": Daniel Rutherford's Discovery
The credit for the discovery of nitrogen is generally attributed to Daniel Rutherford, a Scottish physician and chemist. In 1772, Rutherford conducted a series of experiments that marked a significant step toward isolating and identifying this previously unknown gas.
His approach involved placing a mouse in a closed container until it could no longer survive. He then burned a candle in the remaining air until it, too, extinguished. Rutherford found that the remaining air could not support combustion or life.
He demonstrated that it was distinct from carbon dioxide (or "fixed air" as it was then known) by using an alkaline solution to absorb the carbon dioxide produced during respiration and combustion. What remained was what Rutherford termed "noxious air" or "mephitic air"—air that was demonstrably unfit for breathing and incapable of supporting combustion.
Circumstances and Significance
It's crucial to understand the context of Rutherford's work. He wasn't necessarily setting out to discover a new element. Instead, he was investigating the properties of air and the effects of respiration and combustion on its composition.
While Rutherford identified the "noxious air," he didn't fully recognize it as a new element. He saw it as a phlogisticated substance, fitting within the then-dominant phlogiston theory of combustion. It was Carl Wilhelm Scheele, independently, who also isolated nitrogen around the same time and called it "vitiated air."
Despite his adherence to the phlogiston theory, Rutherford's meticulous experiments and detailed observations laid the groundwork for later scientists to understand the true nature of nitrogen.
From "Noxious Air" to "Nitrogen": The Influence of Lavoisier
The naming of nitrogen is intertwined with the contributions of Antoine Lavoisier, the renowned French chemist who revolutionized the field with his work on combustion and the rejection of the phlogiston theory.
Lavoisier recognized that the "noxious air" identified by Rutherford was a distinct element and a major component of the atmosphere. However, he initially named it "azote" which translates to "lifeless" or "without life" in Greek, reflecting its inability to support respiration.
"Azote": A Reflection of Inertness
Lavoisier's choice of "azote" highlights a key characteristic of nitrogen: its relative inertness. While not entirely unreactive, nitrogen gas is far less reactive than oxygen, which readily supports combustion and life.
The name "azote" acknowledged this crucial difference and emphasized the element's distinct properties compared to other atmospheric gases. Although "azote" remains the name for nitrogen in French and some other languages, the name "nitrogen" eventually gained prominence internationally.
"Nitrogen": A Connection to Nitre
The term "nitrogen" was later suggested by French chemist Jean-Antoine Chaptal in 1790. The name "nitrogen" derives from the Greek words "nitron" and "genes," meaning "nitre-forming," which refers to nitre (potassium nitrate) and alludes to nitrogen's presence in nitric acid and nitrates.
This naming choice highlighted nitrogen's role in the formation of these important compounds, which were known and used long before the element itself was isolated and identified. "Nitrogen" provided a more accurate and practical association with the element's chemical behavior and applications.
The historical journey of nitrogen's discovery and naming is a reminder of the iterative nature of scientific progress. From Rutherford's initial isolation of "noxious air" to Lavoisier's conceptualization of "azote" and Chaptal's suggestion of "nitrogen," each step built upon the previous one, gradually revealing the true identity and significance of this essential element.
Nitrogen's Place in the Periodic Table: Classification and Implications
Having journeyed through the historical roots of nitrogen's discovery and nomenclature, it's time to anchor this vital element within the landscape of the periodic table. Nitrogen's position is far from arbitrary; it profoundly influences its chemical behavior and dictates its classification as a nonmetal. This section delves into the specifics of nitrogen's placement and its implications.
We'll dissect the key characteristics that set nitrogen apart from its metallic and metalloid counterparts. Prepare to explore the nuanced world of elemental classification and understand why nitrogen proudly resides among the nonmetals.
Nitrogen's Address: Group 15 and Period 2
Nitrogen resides in Group 15 (also known as the pnictogens) and Period 2 of the periodic table. This location is critical. Group 15 dictates its characteristic five valence electrons, a key determinant in its bonding behavior.
Being in Period 2 means that nitrogen's valence electrons occupy the second energy level, influencing its size and electronegativity.
Group 15: The Pnictogens
Group 15 elements share similar valence electron configurations, leading to some common chemical traits. However, metallic character increases as you move down the group. Nitrogen, at the top, is the most nonmetallic.
The other elements in the group (phosphorus, arsenic, antimony, and bismuth) exhibit a gradual transition from nonmetallic to metallic properties. This contrast highlights nitrogen's distinctly nonmetallic nature.
Period 2: Small Size, High Electronegativity
Elements in Period 2 are known for their relatively small atomic radii and high electronegativity values. This is because the valence electrons are closer to the nucleus, experiencing a stronger positive charge.
Nitrogen's small size and high electronegativity make it prone to forming strong covalent bonds, particularly the famously stable triple bond in N2.
Metals, Nonmetals, and Metalloids: Defining the Boundaries
The periodic table is broadly divided into metals, nonmetals, and metalloids (or semimetals). Understanding these categories is crucial to appreciating nitrogen's classification.
Metals are typically shiny, malleable, ductile, and excellent conductors of heat and electricity. Nonmetals, on the other hand, often lack these properties. Metalloids exhibit intermediate characteristics, sometimes behaving like metals and sometimes like nonmetals.
Key Distinctions: Why Nitrogen Isn't a Metal
Nitrogen unequivocally falls into the nonmetal category. Several key properties underscore this classification:
-
Lack of Metallic Luster: Nitrogen, in its gaseous form, is colorless and lacks the characteristic shine of metals.
-
Poor Conductivity: Nitrogen is a poor conductor of both heat and electricity. Metals excel in these areas due to the mobility of their valence electrons.
-
Brittle Nature: While not applicable in its gaseous state, solid forms of nitrogen (achieved under extreme conditions) are expected to be brittle, unlike the malleable and ductile nature of metals.
-
High Ionization Energy and Electronegativity: Nitrogen has a high ionization energy, meaning it requires a lot of energy to remove an electron. It also has a high electronegativity, indicating a strong tendency to attract electrons. Metals typically have low ionization energies and electronegativities.
Nitrogen vs. Metalloids
Metalloids (such as silicon and germanium) possess properties intermediate between metals and nonmetals. While nitrogen shares some similarities with metalloids in terms of bonding versatility, its overall behavior strongly aligns with nonmetals.
Metalloids often exhibit semiconductivity, a property absent in nitrogen. Additionally, nitrogen lacks the characteristic metallic appearance that some metalloids possess.
By understanding nitrogen's placement in the periodic table and comparing its properties to those of metals and metalloids, we can confidently classify it as a nonmetal. This classification is not merely a label; it's a key to understanding nitrogen's chemical behavior and its role in the world around us.
Electronic Structure and Bonding: Understanding Nitrogen's Behavior
Having established nitrogen's nonmetallic classification and its strategic placement on the periodic table, the narrative now pivots to the heart of its behavior: its electronic structure and bonding characteristics. These fundamental aspects dictate how nitrogen interacts with other elements, forging molecules both simple and complex.
This section is dedicated to unpacking the intricacies of nitrogen's electron configuration, electronegativity, and ionization energy. These properties aren't mere data points; they are the keys to understanding nitrogen's unique role in the chemical world.
Delving into Nitrogen's Electron Configuration
At the core of nitrogen's chemical personality lies its electron configuration: 1s22s22p3. This arrangement reveals that nitrogen possesses five valence electrons, residing in its outermost (second) energy level.
These five valence electrons are paramount to understanding nitrogen's bonding preferences. They dictate its ability to form up to three covalent bonds, a capability that underpins countless nitrogen-containing compounds.
The two s electrons are held closer to the nucleus, while the three p electrons are oriented along the x, y, and z axes, respectively. This spatial arrangement is crucial, setting the stage for the formation of sigma (σ) and pi (π) bonds.
The Significance of Half-Filled p Orbitals
Nitrogen's three p electrons each occupy a separate p orbital. This half-filled p orbital configuration contributes significantly to nitrogen's stability, especially in its diatomic form (N2).
According to Hund's rule, electrons individually occupy orbitals within a subshell before doubling up in any one orbital. This arrangement minimizes electron-electron repulsion, resulting in a more stable electronic state.
This increased stability translates into the strong triple bond that characterizes molecular nitrogen, rendering it relatively inert under normal conditions. It's a testament to the power of electron configuration in dictating chemical behavior.
Electronegativity: Nitrogen's Electron Appetite
Electronegativity, a measure of an atom's ability to attract electrons in a chemical bond, is another critical aspect of nitrogen's behavior. With an electronegativity value of approximately 3.04 on the Pauling scale, nitrogen is considered quite electronegative.
This high electronegativity means that nitrogen has a strong affinity for electrons. When bonding with less electronegative elements, nitrogen will tend to pull electron density towards itself, resulting in polar covalent bonds.
This polarity influences the physical and chemical properties of nitrogen-containing compounds, affecting everything from their solubility to their reactivity.
Ionization Energy: Resisting Electron Loss
Ionization energy is the energy required to remove an electron from an atom in its gaseous state. Nitrogen possesses a relatively high ionization energy, reflecting the stability of its electron configuration.
Removing an electron from nitrogen requires a significant energy input. This is because the effective nuclear charge experienced by the valence electrons is relatively high, making them more tightly bound to the nucleus.
The first ionization energy of nitrogen is higher than that of oxygen, despite oxygen having one more proton. This seemingly counterintuitive trend stems from nitrogen's half-filled p orbital configuration, which provides extra stability.
Bonding Behavior: The Triple Bond and Beyond
Nitrogen's electronic properties converge to dictate its characteristic bonding behavior. The most iconic example is the formation of the nitrogen molecule (N2), held together by a robust triple bond consisting of one sigma (σ) and two pi (π) bonds.
This triple bond is exceptionally strong, requiring a substantial amount of energy to break. It's the primary reason why molecular nitrogen is so unreactive under ambient conditions.
However, nitrogen is also capable of forming single and double bonds with other elements, leading to a diverse array of nitrogen-containing compounds. Ammonia (NH3), with its single bonds to hydrogen, is a prime example.
The geometry and properties of these compounds are profoundly influenced by the interplay between nitrogen's electron configuration, electronegativity, and ionization energy. Understanding these fundamental aspects unlocks a deeper appreciation for nitrogen's role in chemistry and biology.
Nitrogen's Physical States: Gas, Liquid, and Beyond
Nitrogen, an element vital to life and industry, manifests in distinct physical states depending on temperature and pressure. From the air we breathe to cryogenic applications, understanding these states is crucial to appreciating nitrogen's versatility. Let's delve into the characteristics and applications of nitrogen as a gas, a liquid, and in its more exotic solid forms.
Gaseous Nitrogen: The Ubiquitous Diatomic Molecule
Nitrogen's most common form is as a gas, specifically diatomic molecular nitrogen (N2). This molecule constitutes about 78% of the Earth's atmosphere, making it the most abundant free element on our planet.
Its prevalence is a direct result of the incredibly strong triple bond that holds the two nitrogen atoms together. This bond requires significant energy to break, rendering N2 relatively inert under normal conditions.
Boiling Point and Inert Properties
Nitrogen gas exists as such above its boiling point of -195.79 °C. At room temperature and pressure, it is odorless, colorless, and tasteless.
Its inertness makes it invaluable in various industrial applications.
Nitrogen gas is frequently used to create inert atmospheres in manufacturing processes where oxidation is undesirable, such as in the production of electronics and certain chemicals. It also plays a crucial role in food packaging, preventing spoilage by displacing oxygen.
Liquid Nitrogen: Embracing the Cold
When cooled below its boiling point, nitrogen transitions into its liquid state. Liquid nitrogen (LN2) is a cryogenic fluid with a melting point of -210.01 °C.
Its extremely low temperature makes it an indispensable tool in a variety of scientific and industrial fields.
Cryogenic Applications: Cooling and Preservation
The most prominent application of liquid nitrogen is its use as a cryogen. It is employed to rapidly cool materials, a process that can induce unique properties or preserve biological samples.
In medicine, liquid nitrogen is used for cryosurgery, the freezing and removal of unwanted tissue such as warts or cancerous cells. It also plays a critical role in the long-term preservation of biological samples, including cells, tissues, and even entire organisms.
Furthermore, liquid nitrogen is used for shrink-fitting machine parts, where metal components are cooled to contract them for easy assembly, then allowed to expand for a tight fit. The food industry also uses it for quick freezing of food products, maintaining quality and preventing ice crystal formation.
Allotropes and Phases: Exploring the Extremes
While gaseous and liquid nitrogen are the most familiar states, nitrogen can also exist in various solid forms, known as allotropes, under extreme conditions of pressure and temperature.
These allotropes exhibit different crystal structures and physical properties, revealing the complex nature of nitrogen at the atomic level.
High-Pressure Phases and Transitions
Under intense pressure, nitrogen atoms can rearrange themselves into a variety of crystalline structures. These high-pressure phases are often studied to understand the behavior of matter under extreme conditions, such as those found in the interiors of planets.
The transitions between these phases are governed by complex thermodynamic principles and can be induced by varying temperature and pressure. Research into these phase transitions provides insights into the fundamental forces that govern the interactions between atoms and molecules. These studies often involve sophisticated techniques such as X-ray diffraction and Raman spectroscopy to characterize the crystal structures of the different allotropes.
Chemical Reactivity and Compounds: A Look at Nitrogen's Interactions
While nitrogen often projects an image of passivity, its chemical behavior reveals a fascinating duality. Nitrogen's relative inertness stems from the formidable triple bond within the N2 molecule, but this doesn't tell the whole story. When conditions are right, nitrogen engages in critical chemical transformations, forming compounds essential for life and industry. These reactions, though sometimes requiring significant energy input, are the cornerstone of nitrogen's role in our world.
The Inert Majesty of Molecular Nitrogen
Nitrogen's reluctance to react under normal conditions is a direct consequence of its electronic structure. The diatomic nitrogen molecule (N2) boasts a remarkably stable triple bond, comprised of one sigma bond and two pi bonds. This triple bond requires a staggering amount of energy to break, making N2 exceptionally unreactive with most substances at ambient temperatures.
This inherent inertness is what makes nitrogen gas such a valuable tool in industrial settings. It can blanket sensitive processes, preventing unwanted oxidation or other reactions. However, the very stability that makes nitrogen useful also presents a challenge: how do we unlock its potential to form vital compounds?
Nitrogen Fixation: Taming the Inert
The process of converting atmospheric nitrogen (N2) into reactive forms, such as ammonia (NH3), is known as nitrogen fixation. This is a crucial step in the nitrogen cycle and is essential for supporting life on Earth. The strong triple bond of N2 must be broken to allow nitrogen atoms to bond with hydrogen or other elements, and this requires significant energy input.
Natural Processes: A Symphony of Microbes
Nature accomplishes nitrogen fixation through various means, primarily through the action of certain microorganisms. Diazotrophs, including bacteria like Rhizobium found in the root nodules of leguminous plants, possess the unique ability to fix atmospheric nitrogen. These bacteria use the enzyme nitrogenase to catalyze the reduction of N2 to ammonia (NH3), providing a natural source of fixed nitrogen for plant growth.
Atmospheric lightning strikes also contribute to nitrogen fixation, albeit to a lesser extent. The intense energy of lightning can break the nitrogen triple bond, allowing nitrogen to react with oxygen in the air to form nitrogen oxides, which eventually get converted to nitrates in the soil.
The Haber-Bosch Process: An Industrial Revolution
The 20th century witnessed a revolution in nitrogen fixation with the development of the Haber-Bosch process. This industrial process, developed by German chemists Fritz Haber and Carl Bosch, enables the large-scale production of ammonia from nitrogen and hydrogen gas.
The Haber-Bosch process, which utilizes high temperatures and pressures along with an iron catalyst, has transformed agriculture by providing a readily available source of nitrogen fertilizer. While it has significantly boosted food production worldwide, its energy intensity and environmental impacts are subjects of ongoing research and optimization efforts.
Ammonia (NH3): The Cornerstone of Nitrogen Chemistry
Ammonia (NH3) stands as a central figure in the realm of nitrogen compounds. Its significance stems from its role as a primary source of fixed nitrogen, essential for the synthesis of fertilizers, polymers, and various other chemicals.
Fertilizer Production: Nourishing the World
The vast majority of ammonia produced globally is used in the manufacture of nitrogen-based fertilizers. These fertilizers provide plants with the nitrogen they need for growth and development, boosting crop yields and sustaining global food production.
Ammonia is converted into various fertilizer forms, including ammonium nitrate (NH4NO3), urea (CO(NH2)2), and ammonium sulfate ((NH4)2SO4). These fertilizers are widely used in agriculture to replenish soil nitrogen levels and support healthy plant growth.
Nitrogen in Action: Industrial and Scientific Applications
Nitrogen's influence extends far beyond the realm of basic chemistry; it is a workhorse in both industrial processes and cutting-edge scientific investigations. Its unique properties, from inertness to extreme cold, make it indispensable across diverse applications. This section explores some of the key ways nitrogen is harnessed, showcasing its practical utility and its role in shaping modern technology and research.
Industrial Prowess: Nitrogen as a Manufacturing Essential
Nitrogen's industrial applications are vast and varied, reflecting its versatility as a chemical element. Its two most prominent roles are as an inert atmosphere and as a cryogenic fluid, each contributing significantly to manufacturing, food processing, and more.
The Shield of Inertness: Preventing Unwanted Reactions
Nitrogen gas, thanks to its inherent inertness, serves as an essential protective atmosphere in numerous industrial processes. By displacing oxygen and other reactive gases, nitrogen creates an environment where unwanted oxidation or other chemical reactions are prevented.
This is particularly crucial in the electronics industry, where even trace amounts of oxygen can damage sensitive components during manufacturing. Similarly, in the pharmaceutical sector, nitrogen blanketing ensures the integrity of drug production by minimizing degradation from atmospheric exposure.
The food processing industry also relies heavily on nitrogen to preserve food quality and extend shelf life. Modified atmosphere packaging (MAP) replaces the air inside food packages with nitrogen, slowing down spoilage and preserving freshness.
This technique is widely used for packaging fresh produce, meats, and other perishable items, helping to reduce food waste and ensure product safety.
Cryogenic Applications: Liquid Nitrogen's Deep Freeze
Liquid nitrogen (LN2), with its extremely low boiling point (-195.79 °C), is a powerful cryogenic fluid with a wide range of industrial and scientific applications. Its ability to rapidly cool materials makes it invaluable for processes requiring precise temperature control or extreme cold.
One of the most well-known uses of liquid nitrogen is in cryogenics, the study and application of very low temperatures. It is used for preserving biological samples, such as sperm, eggs, and tissues, for medical and research purposes.
LN2 is also used in the metalworking industry for shrink-fitting, a technique where metal parts are cooled to contract them before assembly. The cooled part then expands upon warming, creating a tight, secure fit.
Beyond that, LN2 is used for cooling superconductors, freezing foods quickly to maintain quality, and even creating special effects in the entertainment industry.
Scientific Frontiers: Nitrogen in Research and Development
Nitrogen plays a critical role in scientific research, enabling experiments and discoveries across diverse fields. Its behavior under extreme conditions and its ability to modify material surfaces make it an invaluable tool for scientists.
Probing the Extremes: High-Pressure Physics
The study of nitrogen under extreme pressure, known as high-pressure physics, reveals fascinating insights into the behavior of matter at the atomic level. By subjecting nitrogen to immense pressures, scientists can observe novel phases and structures that do not exist under normal conditions.
These experiments often involve diamond anvil cells, which can compress samples to pressures exceeding those found at the Earth's core. High-pressure studies of nitrogen have led to the discovery of new allotropes and phases, broadening our understanding of chemical bonding and material properties.
This knowledge can then be applied to the development of new materials with enhanced strength, hardness, or other desirable characteristics.
Nitriding: Surface Hardening and Material Stabilization
Nitriding is a surface hardening process that uses nitrogen to improve the wear resistance, fatigue strength, and corrosion resistance of metals. In this process, nitrogen atoms are diffused into the surface of a metal, forming a hard, wear-resistant layer.
Nitriding is widely used in the automotive, aerospace, and toolmaking industries to enhance the performance and lifespan of critical components. By creating a hard surface layer, nitriding protects the underlying metal from wear and tear, extending the service life of the component.
This technique is particularly valuable for components subjected to high stress or harsh environments, such as gears, bearings, and cutting tools. The incorporation of nitrogen into the metal lattice also enhances its stability and resistance to corrosion.
Video: Is Nitrogen a Metal? Properties & Facts
So, is nitrogen a metal? Hopefully, now you have a clearer picture! It's a fascinating element with some surprising properties, but at the end of the day, it firmly remains in the nonmetal category. Keep exploring the periodic table; you never know what you might discover!