Nitrogen Electron Configuration: A Simple Guide
Understanding how elements behave at the atomic level is key to mastering chemistry, where nitrogen is a crucial element. The nitrogen electron configuration, specifically, dictates how nitrogen interacts with other elements to form various molecules. The Schrödinger equation, a cornerstone of quantum mechanics, provides the theoretical framework for determining the arrangement of electrons in nitrogen. This arrangement influences nitrogen's role in biological processes, such as in the production of amino acids, where it forms the backbone of these essential building blocks. The National Institute of Standards and Technology (NIST) provides reliable data and standards essential for accurately determining and referencing the electron configurations of elements, including nitrogen.
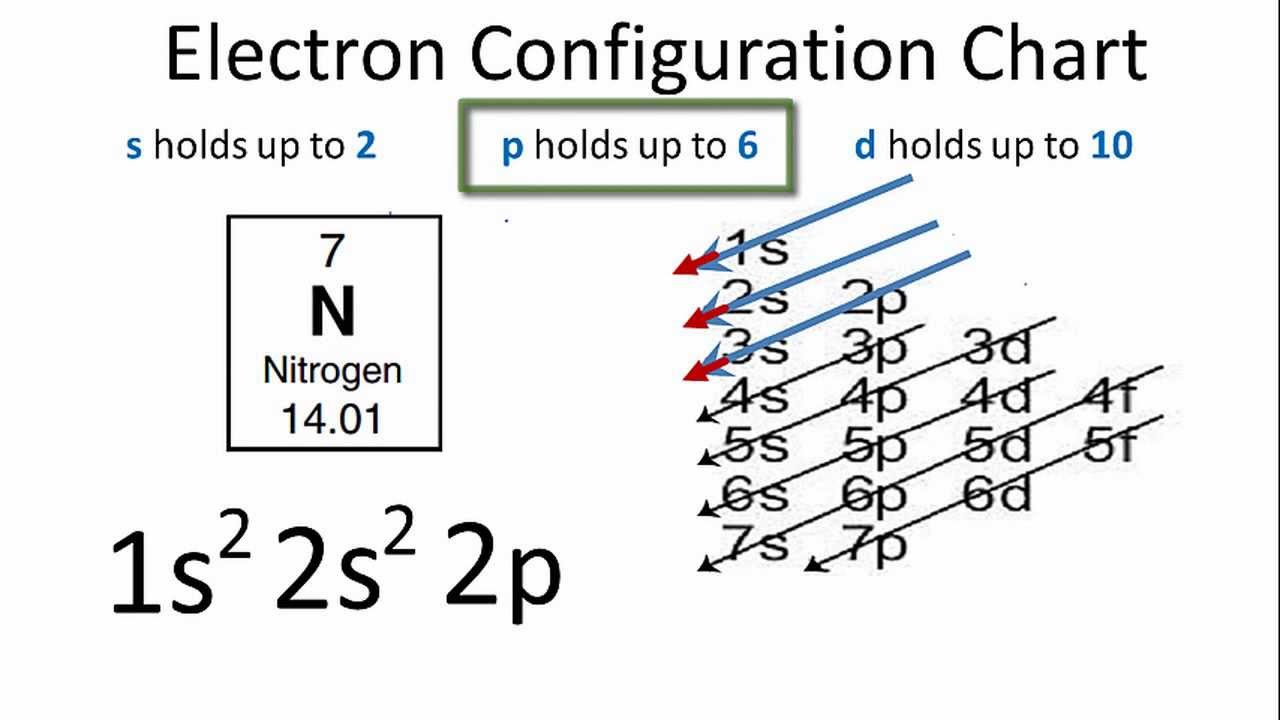
Image taken from the YouTube channel Wayne Breslyn (Dr. B.) , from the video titled Nitrogen Electron Configuration .
Unlocking the Secrets of Electron Configuration: A Deep Dive into Nitrogen
Electron configuration is the foundational blueprint that dictates an atom's chemical behavior. It's the address book of electrons, revealing their arrangement within the atom's energy levels and sublevels. Understanding this arrangement is paramount to predicting how an atom will interact with others, forming molecules and driving chemical reactions.
Think of it as understanding the seating arrangement in a theater. Knowing where each actor (electron) is positioned allows you to anticipate their movements and interactions on stage (chemical reactions).
Why Electron Configuration Matters
The electron configuration dictates an element's properties. From its reactivity to its bonding behavior. Without understanding the electron configuration, predicting chemical interactions is difficult.
It explains why some elements are highly reactive while others are inert. It also helps us predict how they will form bonds and create new compounds.
Nitrogen: An Element of Immense Importance
As we delve into electron configuration, we'll be using Nitrogen (N) as our key example. Nitrogen, with its atomic number of 7, is an exceptionally abundant element, making up approximately 78% of the Earth's atmosphere.
But its significance extends far beyond its prevalence in the air we breathe.
The Significance of Nitrogen
Nitrogen is a critical component of amino acids, the building blocks of proteins, and thus essential for all life. It's also a key element in DNA and RNA, the genetic materials that carry the blueprints for life.
Furthermore, Nitrogen is heavily used in industry, notably in the production of ammonia (NH₃) for fertilizers. Nitrogen compounds are essential for crop growth. They are also found in various other applications, including the manufacturing of explosives and pharmaceuticals.
Unveiling Nitrogen's Electronic Structure
This exploration will provide a thorough explanation of Nitrogen's electron configuration. We will dissect the principles that govern electron arrangement within its atom.
By understanding the placement of its seven electrons, we unlock vital insights into its unique chemistry. We'll see why Nitrogen forms the bonds it does and how it interacts with other elements to create the diverse molecules that shape our world. Get ready to understand the underlying electronic structure of one of the most important elements in our universe.
Laying the Groundwork: Atomic Structure and the Dance of Electrons
Before we can unravel the intricacies of Nitrogen's electron configuration, it's essential to solidify our understanding of the fundamental building blocks: atomic structure and the nature of orbitals. These concepts provide the framework upon which electron configuration is built, much like understanding musical notes is necessary to appreciate a symphony.
The Atomic Number: A Key to Unlocking Electron Configuration
The atomic number (Z) is the defining characteristic of an element, serving as its unique identifier. For Nitrogen, Z = 7, meaning it possesses 7 protons within its nucleus.
In a neutral atom, the number of protons is always equal to the number of electrons. Therefore, Nitrogen also has 7 electrons. These are the electrons we will be arranging in its electron configuration.
This seemingly simple number is the cornerstone of understanding electron configuration because it tells us precisely how many electrons we need to place around the atom.
The atomic number is our guiding star, directing us as we navigate the complex world of electron arrangement.
Electron Shells: Energy Levels and the Arrangement Around the Nucleus
Electrons don't simply float randomly around the nucleus; they exist in specific energy levels, also known as electron shells. These shells are designated by the principal quantum number, n, which can be 1, 2, 3, and so on, with higher numbers indicating higher energy levels.
Think of these shells as floors in a building, where electrons reside on different floors based on their energy.
Electrons closer to the nucleus (lower n values) have lower energy and are more tightly bound to the atom. The first shell (n = 1) is closest to the nucleus and can hold a maximum of 2 electrons.
The second shell (n = 2) is further out and can accommodate up to 8 electrons. This arrangement of electrons around the nucleus is the key to understanding how atoms interact and form bonds.
For Nitrogen (Z = 7), we know it has 7 electrons. The first two electrons fill the n = 1 shell, and the remaining five electrons occupy the n = 2 shell.
Orbitals: The Electron's Address Within the Atom
Within each electron shell, electrons reside in specific regions of space called orbitals. Orbitals are not fixed paths like planets orbiting a sun, but rather probability distributions that describe where an electron is most likely to be found.
Orbitals come in different shapes and energy levels. They are categorized into sublevels designated as s, p, d, and f.
The s Orbitals
The s orbitals are spherical in shape. Each shell contains one s orbital. The s orbital can hold a maximum of 2 electrons.
The p Orbitals
The p orbitals are dumbbell-shaped and exist in sets of three within each shell (starting from n = 2). These three p orbitals are oriented along the x, y, and z axes. Each set of p orbitals can hold a maximum of 6 electrons (2 electrons per orbital).
The d and f Orbitals
The d orbitals are more complex in shape and exist in sets of five (starting from n = 3), capable of holding up to 10 electrons. The f orbitals are even more intricate, existing in sets of seven (starting from n = 4), and can hold up to 14 electrons.
Energy Relationships
The energy levels of these orbitals within a shell follow the order s < p < d < f. This means that within a given shell, s orbitals have the lowest energy, followed by p, then d, and finally f orbitals.
Understanding the shapes, numbers, and energy relationships of these orbitals is essential for accurately predicting how electrons will arrange themselves within an atom, including Nitrogen. This groundwork allows us to proceed to more advanced concepts with confidence.
Key Principles: Directing the Dance of Electrons
Understanding electron configuration isn't just about memorizing patterns; it's about grasping the fundamental principles that govern how electrons arrange themselves around the nucleus. These principles are not arbitrary rules, but rather, arise from the very nature of quantum mechanics, defining the architecture of atoms. Mastering these guiding principles, namely the Aufbau principle, Hund's rule, and the Pauli exclusion principle, provides the critical lens for understanding how electrons populate orbitals and ultimately define an element's chemical properties.
The Aufbau Principle: Building from the Ground Up
The Aufbau principle, derived from the German word for "building up," elegantly dictates the order in which electrons fill atomic orbitals. It asserts that electrons first occupy the lowest energy orbitals available before moving to higher energy levels.
Think of it as filling a container with water: you start at the bottom and work your way up, layer by layer. This "bottom-up" approach ensures that the atom achieves the most stable and energetically favorable configuration.
An energy level diagram is a crucial tool for visualizing the Aufbau principle. This diagram represents the relative energy levels of various orbitals, typically showing the 1s orbital as the lowest, followed by 2s, 2p, and so on. The precise ordering can become more complex for higher energy levels due to sublevel interactions, but the basic principle remains the same: fill orbitals in ascending order of energy.
It's critical to remember that exceptions to the Aufbau principle exist, particularly for transition metals. These exceptions stem from the subtle energy differences between certain d and s orbitals, leading to configurations that maximize stability. However, for the majority of elements, including Nitrogen, the Aufbau principle serves as a reliable guideline.
Hund's Rule: Maximizing Spin Multiplicity
Hund's rule delves into the specific manner in which electrons populate orbitals within a subshell. It states that electrons will individually occupy each orbital within a subshell before any orbital is doubly occupied. Moreover, these unpaired electrons will have the same spin (either all spin-up or all spin-down), maximizing the total spin multiplicity.
Imagine electrons as commuters on a bus. They prefer to have their own seat before sharing with someone else. This preference stems from electron-electron repulsion, as spreading out minimizes these repulsive forces.
The concept of spin multiplicity arises from the intrinsic angular momentum of electrons, often referred to as "spin." Electrons can have spin-up (+1/2) or spin-down (-1/2). Hund's rule favors configurations with the maximum number of unpaired electrons with parallel spins, leading to a lower energy state. These electrons are not attracted but repulsed.
For instance, when filling the 2p orbitals, each of the three 2p orbitals will initially receive one electron before any of them receives a second. This arrangement results in a lower energy state compared to pairing electrons in one 2p orbital while leaving the others empty.
The Pauli Exclusion Principle: Defining Individuality
The Pauli exclusion principle is a cornerstone of quantum mechanics, with profound implications for atomic structure. It states that no two electrons in an atom can have the same set of four quantum numbers.
Quantum numbers are a set of values that describe the state of an electron: principal quantum number (n, energy level), azimuthal quantum number (l, orbital shape), magnetic quantum number (ml, orbital orientation), and spin quantum number (ms, spin direction).
Since each orbital is defined by a unique set of n, l, and ml values, the Pauli exclusion principle dictates that an orbital can hold a maximum of two electrons, and these two electrons must have opposite spins (+1/2 and -1/2). This principle prevents all the electrons from collapsing into the lowest energy state.
The Pauli exclusion principle is responsible for the structure of the periodic table, as it limits the number of electrons that can occupy each energy level and sublevel, creating the periodic recurrence of chemical properties.
Nitrogen's Electron Configuration: A Detailed Look
Now that we have established the fundamental principles guiding electron arrangement, let's apply them specifically to Nitrogen (N), an element vital to life and industry. Understanding how to derive Nitrogen's electron configuration is not just about arriving at the correct answer; it's about solidifying your grasp of these core chemical concepts. In this section, we will dissect the process step-by-step, illuminating how the Aufbau principle, Hund's rule, and the Pauli exclusion principle converge to define the electronic structure of this essential element.
Unveiling Nitrogen's Electronic Blueprint: 1s²2s²2p³
The electron configuration of Nitrogen is 1s²2s²2p³. This notation encapsulates the complete picture of how Nitrogen's seven electrons are distributed among its various energy levels and orbitals. Let's break down how we arrive at this configuration by applying the previously discussed rules.
The Step-by-Step Derivation
Step 1: The Aufbau Principle and Energy Level Ordering
We begin by invoking the Aufbau principle, which directs us to fill orbitals in order of increasing energy. For Nitrogen, this means we start with the 1s orbital, the lowest energy orbital in the atom.
The 1s orbital can accommodate a maximum of two electrons (according to the Pauli Exclusion Principle), so we fill it completely: 1s².
Next, we move to the second energy level (n=2). The 2s orbital is the next lowest in energy. It too can hold a maximum of two electrons, giving us 2s².
Now we've accounted for four of Nitrogen's seven electrons. We still have three electrons to place. The next available orbitals are the 2p orbitals.
Step 2: Hund's Rule and the 2p Orbitals
The 2p subshell consists of three degenerate (equal energy) orbitals. Here, Hund's rule comes into play. Hund's rule tells us that electrons will individually occupy each of these 2p orbitals before any pairing occurs.
This leads to each of the three 2p orbitals receiving one electron each. Thus, we get 2p³.
It's crucial to remember that each of these three electrons will have the same spin (we can arbitrarily define it as 'up' spin). This maximizes the overall spin and minimizes electron-electron repulsion, leading to a more stable configuration.
Step 3: Confirming with the Pauli Exclusion Principle
Finally, we must ensure that our derived electron configuration aligns with the Pauli exclusion principle.
Each electron in our configuration (1s²2s²2p³) has a unique set of quantum numbers. Specifically, the two electrons in the 1s orbital have different spin quantum numbers. The same is true for the two electrons in the 2s orbital. And according to Hund's Rule, the 2p orbitals each have electrons in separate orbitals with the same spin.
Therefore, we can be confident that our result does not violate the Pauli exclusion principle.
Bringing it all Together: Nitrogen's Configuration Explained
By systematically applying the Aufbau principle, Hund's rule, and the Pauli exclusion principle, we have successfully determined Nitrogen's electron configuration: 1s²2s²2p³.
Each principle played a vital role: the Aufbau principle established the filling order, Hund's rule dictated the filling of the 2p orbitals, and the Pauli Exclusion Principle places the limit for each electron orbital capacity.
This configuration is not merely an arbitrary arrangement of electrons. It's a carefully orchestrated distribution that minimizes energy and maximizes stability, giving rise to Nitrogen's characteristic chemical behavior.
Valence and Core Electrons in Nitrogen: Unveiling Chemical Reactivity
To fully grasp Nitrogen's behavior, we must distinguish between valence and core electrons. These two electron categories play dramatically different roles in shaping chemical interactions. Understanding this distinction unlocks deeper insights into Nitrogen's bonding capabilities and reactivity.
Valence Electrons: The Architects of Chemical Bonds
Valence electrons are those residing in the outermost electron shell of an atom. These are the electrons actively involved in forming chemical bonds with other atoms. They are the "social butterflies" of the atomic world, engaging in interactions that lead to the creation of molecules and compounds.
For Nitrogen, the electron configuration (1s²2s²2p³) reveals that the outermost shell is the second shell (n=2).
Within this shell, there are two 2s electrons and three 2p electrons. This means Nitrogen possesses a total of five valence electrons (2s²2p³).
These five valence electrons are responsible for Nitrogen's diverse bonding behavior. Nitrogen can form single, double, or triple bonds. It can also participate in coordinate covalent bonds, depending on the chemical environment.
Core Electrons: The Silent Guardians
In contrast to valence electrons, core electrons are those occupying the inner electron shells. They are much closer to the nucleus and tightly bound to the atom. As a result, they are generally considered to be chemically inert. They are the silent guardians of the atomic structure.
In the case of Nitrogen, the two 1s electrons (1s²) constitute its core electrons. These electrons reside in the innermost shell (n=1).
The core electrons are buried deep within the atom. They are shielded from external influences by the valence electrons.
Because of their location and strong attraction to the nucleus, core electrons typically do not participate in chemical bonding or reactions. Their primary role is to contribute to the overall nuclear charge experienced by the valence electrons.
The Significance of Differentiation
The distinction between valence and core electrons is crucial for simplifying our understanding of chemical reactions. By focusing on the valence electrons, we can accurately predict and explain how atoms will interact with each other.
We can predict the types of chemical bonds they will form. Ignoring the core electrons allows us to streamline our analysis, focusing only on the electrons actively participating in chemical change.
This simplification is fundamental to understanding and predicting the chemical properties of Nitrogen and other elements. It allows us to move from a complex electronic structure to a more intuitive understanding of chemical behavior.
Visualizing Electron Configuration: Nitrogen's Orbital Diagram
Having established the electron configuration of Nitrogen, we now turn to visualizing this arrangement through orbital diagrams, often referred to as "box diagrams." These diagrams provide a tangible representation of electron placement within each orbital, solidifying our understanding of the underlying principles.
Constructing the Orbital Diagram for Nitrogen
The orbital diagram uses boxes to represent individual orbitals within each subshell. Each box can hold a maximum of two electrons, adhering to the Pauli Exclusion Principle. We label each box to clearly indicate the corresponding orbital (1s, 2s, 2p).
For Nitrogen (1s²2s²2p³), we begin by drawing boxes for the 1s, 2s, and 2p orbitals. The 1s and 2s subshells each have one orbital (one box each). The 2p subshell has three orbitals (three boxes).
Therefore, the initial setup of the diagram looks like this:
1s: ▢ 2s: ▢ 2p: ▢ ▢ ▢
Filling the Orbitals: Applying Hund's Rule
Next, we fill the orbitals with electrons, following the Aufbau principle (filling from lowest to highest energy) and, crucially, Hund's rule. Hund's rule dictates that within a subshell, electrons individually occupy each orbital before any orbital is doubly occupied. This maximizes spin multiplicity and contributes to a more stable configuration.
First, we fill the 1s orbital with two electrons, represented by upward and downward arrows (↑↓) to indicate opposite spins. This gives us:
1s: ↑↓ 2s: ▢ 2p: ▢ ▢ ▢
We then fill the 2s orbital with two electrons (↑↓):
1s: ↑↓ 2s: ↑↓ 2p: ▢ ▢ ▢
Finally, we populate the 2p orbitals. According to Hund's rule, we first add one electron to each of the three 2p orbitals, all with the same spin (e.g., all upward arrows):
1s: ↑↓ 2s: ↑↓ 2p: ↑ ↑ ↑
Therefore, the completed orbital diagram for Nitrogen is:
1s: ↑↓ 2s: ↑↓ 2p: ↑ ↑ ↑
Interpreting the Diagram: Hund's Rule and Pauli Exclusion Principle in Action
The orbital diagram provides a visual testament to Hund's rule. The three 2p electrons are distributed individually across the three 2p orbitals, each with the same spin. This arrangement illustrates the principle of maximizing unpaired electrons before pairing begins.
Furthermore, the diagram implicitly embodies the Pauli Exclusion Principle. Each orbital (each box) contains a maximum of two electrons, and if two electrons occupy the same orbital, they must have opposite spins (one upward arrow, one downward arrow). No two electrons within the Nitrogen atom share the same set of quantum numbers. The visual language reinforces the underlying scientific rules.
The orbital diagram provides a powerful, intuitive tool for understanding electron configuration. It translates abstract quantum mechanical principles into a clear visual representation, allowing us to better grasp the electronic structure of Nitrogen and its influence on chemical behavior. It allows a clear mental picture to form.
Shorthand Notation: Noble Gas Configuration
Having explored the detailed representation of Nitrogen's electron configuration, we can now delve into a simplified method: noble gas configuration. This shorthand notation offers a concise and efficient way to express electron configurations, especially for larger atoms, while still capturing essential information.
The Need for Shorthand
Writing out the full electron configuration for elements beyond the first few rows of the periodic table can become tedious and space-consuming. The noble gas configuration streamlines this process by leveraging the stable electron configurations of the noble gases. These elements (Helium, Neon, Argon, Krypton, Xenon, and Radon) possess filled electron shells, representing exceptional chemical stability.
By encapsulating the core electrons within the noble gas configuration, we can focus on the valence electrons, which are crucial for chemical bonding.
Identifying the Preceding Noble Gas
To use noble gas notation, we must first identify the noble gas that precedes our element of interest (in this case, Nitrogen) on the periodic table. For Nitrogen (atomic number 7), the preceding noble gas is Helium (atomic number 2).
Helium's electron configuration is 1s². This means that the 1s² portion of Nitrogen's electron configuration (1s²2s²2p³) can be represented by the symbol [He].
Expressing Nitrogen's Electron Configuration with Noble Gas Notation
Using this knowledge, we can rewrite Nitrogen's electron configuration as:
[He] 2s²2p³
This notation signifies that Nitrogen has the same electron configuration as Helium, plus an additional 2s²2p³ electron configuration.
Decoding the Notation:
- [He]: Represents the core electrons, equivalent to the electron configuration of Helium (1s²).
- 2s²: Indicates that the 2s orbital contains two electrons.
- 2p³: Indicates that the 2p orbitals contain three electrons.
Advantages of Noble Gas Notation
The noble gas configuration offers several advantages:
- Conciseness: It significantly shortens the representation of electron configurations.
- Focus on Valence Electrons: It highlights the valence electrons, which are responsible for an element's chemical behavior.
- Ease of Interpretation: It provides a quick overview of the electronic structure and its relationship to the nearest noble gas.
- Enhanced Understanding: It reinforces the concept of filled electron shells and their association with stability.
Practical Application and Interpretation
The noble gas configuration is a valuable tool for quickly assessing an element's reactivity and bonding potential. By focusing on the valence electrons, we can easily predict how an element will interact with other atoms to achieve a more stable electron configuration. This simplified notation enhances comprehension, ensuring a clearer and more approachable learning experience.
Magnetic Properties of Nitrogen
Having established Nitrogen's electron configuration, we can now explore how this arrangement dictates its magnetic properties. Understanding this connection reveals a fascinating aspect of how electron structure influences macroscopic behavior.
Nitrogen, in its atomic state, exhibits a phenomenon known as paramagnetism. This magnetic behavior directly stems from the presence of unpaired electrons within its electron configuration. Let's unpack this further.
Paramagnetism Explained
Paramagnetism arises in substances that possess unpaired electrons. These unpaired electrons possess an intrinsic magnetic moment due to their spin.
In the absence of an external magnetic field, these magnetic moments are randomly oriented, resulting in no net magnetic field.
However, when a paramagnetic substance is placed in a magnetic field, these magnetic moments tend to align themselves with the external field. This alignment results in a weak attraction to the magnetic field.
The strength of the paramagnetic effect is proportional to the number of unpaired electrons present.
Nitrogen's Paramagnetism and Unpaired Electrons
Nitrogen's electron configuration (1s²2s²2p³) is key to understanding its paramagnetism. As we saw earlier, the 2p subshell contains three electrons.
According to Hund's rule, these electrons will individually occupy each of the three 2p orbitals before pairing up in any one orbital.
This results in three unpaired electrons, each contributing to the overall magnetic moment of the Nitrogen atom.
The presence of these three unpaired electrons is precisely what causes Nitrogen in its atomic state to be paramagnetic. The more unpaired electrons, the stronger the interaction.
Contrasting with Diamagnetism
It's helpful to contrast paramagnetism with diamagnetism, another type of magnetic behavior. Diamagnetic substances are characterized by having all their electrons paired.
In these materials, there are no unpaired electron spins to align with an external magnetic field. Instead, an applied magnetic field induces a small magnetic moment opposing the applied field.
This results in a weak repulsion from the magnetic field.
Nitrogen, with its three unpaired electrons, clearly does not exhibit diamagnetism in its atomic state. All electrons must be paired for the element to exhibit this behavior.
Molecular Nitrogen (N₂) and Magnetism
It is important to note that while atomic nitrogen is paramagnetic, molecular nitrogen (N₂) is diamagnetic.
This change occurs because when two nitrogen atoms bond to form N₂, the unpaired electrons pair up to form a triple bond.
This pairing eliminates the unpaired electrons, resulting in the diamagnetic behavior of the molecule.
Understanding the subtle interplay between electron configuration and magnetic properties provides valuable insights into the fundamental nature of matter. Examining how electron arrangements give rise to observable magnetic behavior enhances comprehension and ensures a comprehensive learning experience.
Predicting Electron Configuration: Tools and Resources
Successfully navigating the intricacies of electron configuration relies not only on a firm grasp of principles but also on leveraging available tools. The periodic table and online resources serve as invaluable aids in both predicting and verifying electron configurations.
These resources empower chemists and students alike to confidently explore the electronic structures of elements. By effectively using these tools, we can significantly enhance our understanding and mastery of this fundamental chemical concept.
The Periodic Table: A Roadmap to Electron Configuration
The periodic table isn't merely a catalog of elements; it's an ingeniously organized chart that directly reflects electron configuration. Its very structure provides crucial clues for predicting how electrons are arranged within an atom.
Understanding the periodic table's layout is fundamental. Each row (period) corresponds to a principal quantum number (n), signifying the energy level of the outermost electrons.
The table is also divided into blocks (s, p, d, and f). These blocks indicate which type of orbital is being filled by the element's valence electrons.
For example, elements in the s-block (Groups 1 and 2) are filling their s orbitals. Elements in the p-block (Groups 13-18) are filling their p orbitals, and so on.
Decoding Nitrogen's Configuration from the Periodic Table
Let's consider how we can predict Nitrogen's electron configuration using the periodic table. Nitrogen is in the second period (n=2) and the p-block. This immediately tells us that its valence electrons will be in the 2s and 2p orbitals.
Nitrogen is the third element in the p-block of the second period, indicating that it has three electrons in the 2p orbitals. Combining this with the filled 1s and 2s orbitals leads us to the electron configuration of 1s²2s²2p³.
Utilizing the Periodic Table for Other Elements
This same approach can be applied to predict the electron configurations of other elements. By systematically moving across the periodic table, accounting for the filling of orbitals in each block, we can confidently determine the electron configurations of numerous elements.
The periodic table is an indispensable tool for quickly estimating the ground-state electron configuration of any element.
Online Electron Configuration Calculators
While the periodic table provides a powerful means of prediction, online electron configuration calculators offer a convenient and efficient way to verify your predictions.
These tools automate the process of applying the Aufbau principle, Hund's rule, and the Pauli exclusion principle, providing a reliable confirmation of the electron configuration for a given element.
Benefits of Using Online Calculators
Online calculators offer several advantages. They eliminate the risk of human error in applying the rules for electron filling. They also provide a quick and easy way to check your work, ensuring accuracy in your understanding of electron configuration.
Furthermore, some calculators provide orbital diagrams, offering a visual representation of electron placement. This visualization can deepen your understanding of the spatial arrangement of electrons within an atom.
Cautions and Considerations
While online calculators are useful, it's crucial to remember that they are tools to aid learning, not replace it. Blindly relying on calculators without understanding the underlying principles will hinder your grasp of the fundamental concepts.
It's best to first attempt to predict the electron configuration yourself using the periodic table and then use the calculator to verify your answer. This approach reinforces your understanding and develops your problem-solving skills.
Recommended Resources
Numerous online calculators are available. Look for calculators that not only provide the electron configuration but also offer explanations of the process and visual representations of the orbital diagrams. Khan Academy and similar educational platforms provide interactive electron configuration tools.
By combining a solid understanding of the principles with the strategic use of the periodic table and online calculators, one can master the art of predicting and verifying electron configurations. This skillset forms a critical foundation for further exploration in chemistry.
Video: Nitrogen Electron Configuration: A Simple Guide
Nitrogen Electron Configuration FAQs
Why is understanding nitrogen's electron configuration important?
Understanding nitrogen's electron configuration (1s² 2s² 2p³) is crucial because it determines how nitrogen interacts with other atoms, forming chemical bonds and influencing its reactivity in various compounds. It explains nitrogen's ability to form single, double, or triple bonds.
What do the numbers and letters in the nitrogen electron configuration (1s² 2s² 2p³) represent?
The numbers (1, 2) represent the electron shell (energy level). The letters (s, p) denote the subshells, indicating the shape of the electron orbitals. The superscripts (², ³) show the number of electrons in each subshell. So, 1s² means there are two electrons in the 1s subshell. This arrangement fully defines the nitrogen electron configuration.
How does nitrogen's electron configuration relate to its valence electrons?
Nitrogen has 5 valence electrons, which are the electrons in its outermost shell (the 2nd shell). These valence electrons are the 2s² and 2p³ electrons in the nitrogen electron configuration. These are responsible for nitrogen's bonding behavior.
Can nitrogen achieve a stable electron configuration? If so, how?
Yes, nitrogen can achieve a stable octet electron configuration, similar to noble gases. It does this by forming three covalent bonds, either through three single bonds (like in ammonia, NH₃) or combinations of single, double, or triple bonds with other atoms. By gaining or sharing three electrons, nitrogen attains a full outer shell, leading to a more stable nitrogen electron configuration in compounds.
So, there you have it! Hopefully, understanding nitrogen electron configuration isn't so daunting anymore. With a little practice and these simple guidelines, you'll be confidently writing out electron configurations for elements all across the periodic table. Now go forth and conquer chemistry!