Mouse Anatomy: Unveiling Secrets You Never Knew! [Guide]
Understanding mouse anatomy is pivotal for researchers at institutions like the Jackson Laboratory, where genetic studies rely heavily on murine models. The musculoskeletal system, a key component of mouse anatomy, allows for locomotion essential for behavioral studies. Furthermore, detailed knowledge of mouse anatomy provides a foundation for developing refined surgical techniques, advancing areas like biomedical engineering. The central nervous system within mouse anatomy offers valuable insights into neurological disorders, informing innovative studies using tools such as confocal microscopy to visualize structures in detail.
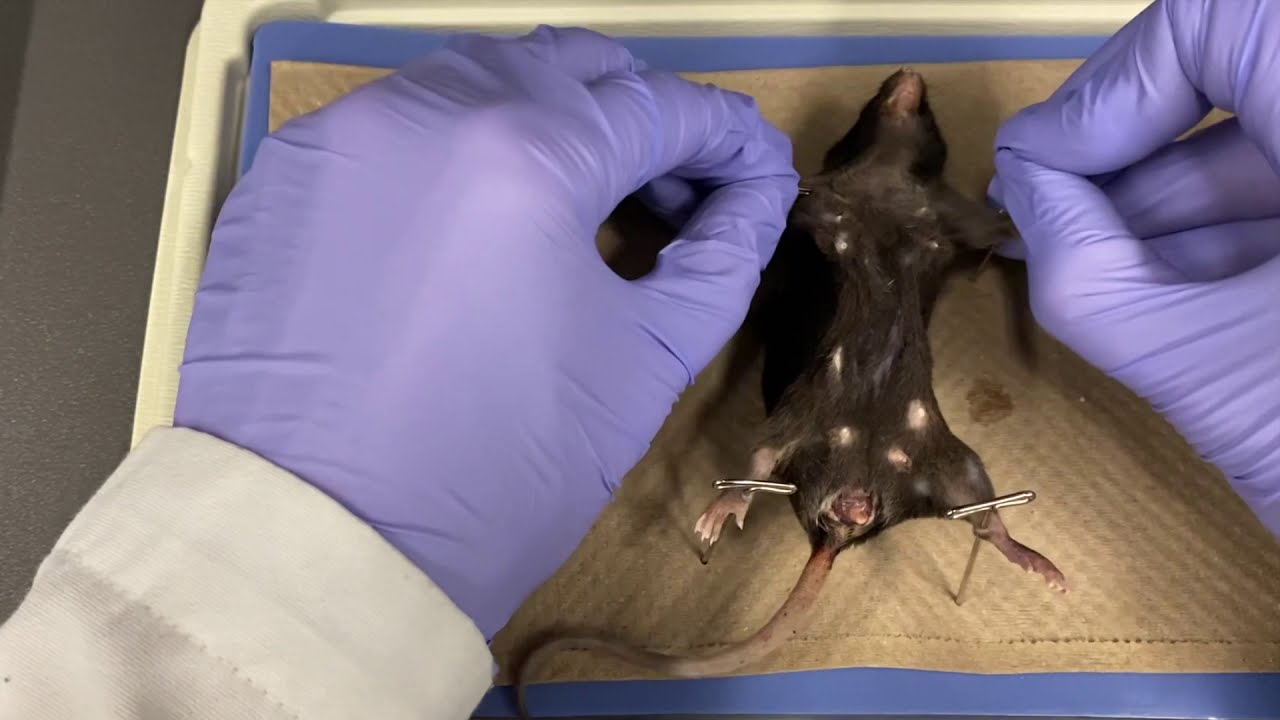
Image taken from the YouTube channel UTM Biology , from the video titled BIO153 Mouse Anatomy .
The laboratory mouse, Mus musculus, stands as a cornerstone of modern biomedical research. Its relatively small size, short lifespan, and genetic similarity to humans make it an invaluable model for studying a wide range of diseases and biological processes. However, effective utilization of the mouse as a research tool hinges on a thorough understanding of its anatomy.
This understanding is not merely an academic exercise; it's a fundamental requirement for accurate data interpretation, precise experimental design, and the ethical conduct of research.
The Indispensable Role of Mouse Anatomy
Mouse anatomy plays a pivotal role in numerous scientific disciplines.
From drug development and toxicology studies to genetics and immunology research, a detailed knowledge of the mouse's internal structures, organ systems, and their interrelationships is crucial.
For example, in vivo drug testing requires precise knowledge of organ locations and tissue types to assess drug distribution, metabolism, and potential toxic effects.
Surgical procedures, a common practice in many research areas, demand intricate knowledge of anatomical landmarks to minimize tissue damage and ensure successful outcomes.
Furthermore, advancements in imaging technologies, such as micro-CT and MRI, necessitate a strong foundation in mouse anatomy to accurately interpret the resulting images and identify subtle changes indicative of disease or treatment effects.
Relevance in Biomedical Research
The mouse model's contribution to biomedical research is undeniable. Its genetic malleability allows scientists to create models that mimic human diseases, providing crucial insights into disease mechanisms and potential therapeutic targets.
Anatomical studies are essential for characterizing these models, identifying phenotypic differences between wild-type and genetically modified mice, and evaluating the efficacy of interventions.
Specifically, mouse anatomy is highly relevant in:
- Cancer research: Understanding tumor growth, metastasis, and response to therapies.
- Immunology: Studying immune cell development, function, and response to pathogens.
- Neuroscience: Investigating brain structure, function, and neurological disorders.
- Cardiovascular research: Examining heart function, blood vessel structure, and cardiovascular diseases.
- Developmental biology: Studying embryonic development and congenital anomalies.
Scope of This Article
This article aims to provide a comprehensive overview of key aspects of mouse anatomy.
While a complete anatomical atlas is beyond the scope of a single article, we will focus on the major organ systems and structures that are most relevant to biomedical researchers.
This will include detailed descriptions of:
- The skeletal system, providing structural support and protection.
- The muscular system, enabling movement and locomotion.
- The nervous system, coordinating bodily functions and sensory perception.
- The circulatory and respiratory systems, delivering oxygen and nutrients.
- The digestive and urogenital systems, processing and eliminating waste.
- A detailed examination of major organs, highlighting their specific functions and structures.
By providing a clear and concise overview of these essential anatomical features, this article aims to empower researchers with the knowledge necessary to effectively utilize the mouse model and advance our understanding of human health and disease.
The anatomical landscape of the mouse reveals intricacies that directly influence experimental outcomes and the validity of research findings. Before delving into the complexities of other systems, it is crucial to understand the fundamental support structure that underpins the entire organism: the skeletal system.
The Skeletal System: Foundation and Framework
The mouse skeletal system, a complex assembly of bones and cartilage, serves as the body's foundation and framework. It provides structural support, protects vital organs, and facilitates movement. A comprehensive understanding of its components is essential for researchers across various disciplines.
Overview of the Skeleton: Bone Composition and Function
The mouse skeleton, like that of other mammals, is primarily composed of bone tissue. Bone is a dynamic composite material consisting of:
-
Organic components (mainly collagen), providing flexibility.
-
Inorganic components (calcium phosphate in the form of hydroxyapatite), providing rigidity and strength.
This unique composition allows the skeleton to withstand mechanical stress and resist fractures. Beyond structural support, bones also play a crucial role in:
-
Mineral storage (calcium and phosphorus).
-
Hematopoiesis (blood cell formation) within the bone marrow.
Detailed Examination of the Mouse Skull
The skull, a complex structure composed of multiple fused bones, protects the delicate brain. It also houses sensory organs and provides attachment points for muscles involved in mastication. Key cranial bones include:
- Frontal bone
- Parietal bone
- Temporal bone
- Occipital bone
The intricate sutures between these bones allow for slight movement and flexibility, particularly during development.
The Mandible: Lower Jaw
The mandible, or lower jaw, is the only movable bone in the skull, enabling chewing and other oral functions. Its robust structure is crucial for withstanding the forces generated during feeding.
Vertebral Column: Structure and Flexibility
The vertebral column, or spine, provides axial support and protects the spinal cord. It is composed of a series of vertebrae, each with a characteristic structure. These vertebrae are divided into distinct regions:
- Cervical (neck)
- Thoracic (chest)
- Lumbar (lower back)
- Sacral (pelvic)
- Caudal (tail)
Intervertebral discs, composed of cartilage, lie between adjacent vertebrae, providing cushioning and flexibility. The vertebral column's curvature and ligamentous attachments contribute to its overall stability and range of motion.
Rib Cage: Protecting Vital Organs
The rib cage, formed by the ribs and sternum, protects the thoracic organs, including the heart and lungs. The ribs articulate with the thoracic vertebrae posteriorly and with the sternum anteriorly, forming a protective cage. This bony shield is vital for safeguarding these essential organs from external trauma.
Limb Bones: Adaptations for Quadrupedal Locomotion
The limb bones of the mouse are adapted for quadrupedal locomotion, enabling efficient movement on four limbs.
Forelimbs
The forelimbs consist of the:
- Humerus (upper arm)
- Radius and ulna (lower arm)
- Carpals (wrist)
- Metacarpals (palm)
- Phalanges (digits)
These bones work together to provide a wide range of motion and dexterity.
Hindlimbs
The hindlimbs, which are generally larger and more powerful than the forelimbs, consist of the:
- Femur (thigh)
- Tibia and fibula (lower leg)
- Tarsals (ankle)
- Metatarsals (sole)
- Phalanges (digits)
The hindlimbs are the primary drivers of locomotion, providing thrust and stability. The specific arrangement and proportions of these bones reflect the mouse's adaptation to a terrestrial lifestyle.
The skeletal system provides the static framework, but the muscular system is what brings the mouse's body to life. It translates neural impulses into movement, maintains posture, and even contributes to vital functions like digestion. Understanding the intricacies of this system is crucial for interpreting a wide range of experimental data, from behavioral studies to investigations of muscular dystrophy.
Muscular System: Powering Movement and Function
The mouse muscular system, like that of all mammals, is a complex network of tissues responsible for generating force and enabling movement. It's not a monolithic entity, but rather a collection of distinct muscle types, each with specialized functions and characteristics. From the powerful muscles of the limbs to the subtle muscles of the face, each component plays a vital role in the animal's survival and interaction with its environment.
There are three primary types of muscle tissue in the mouse: skeletal, smooth, and cardiac. Each type possesses a unique structure and physiological properties that dictate its specific role within the body.
-
Skeletal muscle, as the name suggests, is attached to bones and is responsible for voluntary movements. These muscles are characterized by their striated appearance under a microscope, reflecting the organized arrangement of contractile proteins.
-
Smooth muscle lines the walls of internal organs such as the digestive tract, blood vessels, and bladder. Its contractions are involuntary and contribute to processes like peristalsis, vasoconstriction, and urination.
-
Cardiac muscle is found exclusively in the heart and is responsible for pumping blood throughout the body. Like skeletal muscle, it is striated, but its contractions are involuntary and regulated by the autonomic nervous system.
Major Muscle Groups in the Mouse
The arrangement of muscles within the mouse's body reflects its quadrupedal lifestyle and its reliance on agility and dexterity for survival. Different muscle groups contribute to specific functions, from locomotion to facial expressions.
Muscles of the Limbs: Locomotion and Dexterity
The muscles of the limbs are arguably the most prominent and well-studied muscle groups in the mouse. The hindlimb muscles are particularly powerful, providing the primary propulsive force for locomotion.
These include large muscles like the gastrocnemius and soleus in the calf, responsible for plantar flexion of the foot, and the quadriceps femoris in the thigh, which extends the knee. The forelimb muscles, while smaller, are crucial for fine motor control and dexterity.
These muscles allow the mouse to manipulate objects, climb, and maintain balance.
Muscles of the Trunk: Posture and Stability
The muscles of the trunk play a critical role in maintaining posture, stabilizing the spine, and facilitating breathing. The abdominal muscles, including the rectus abdominis, obliques, and transversus abdominis, provide core strength and protect internal organs.
The back muscles, such as the erector spinae, support the vertebral column and enable movements like extension and lateral flexion.
The intercostal muscles, located between the ribs, assist in respiration by expanding and contracting the rib cage.
Facial Muscles: Limited Facial Expressions
Compared to humans, mice possess a relatively limited range of facial expressions. The facial muscles in mice are primarily involved in functions such as whisking, grooming, and controlling the movement of the ears and eyelids.
Although subtle, these muscles are important for communication and sensory perception. For instance, whisking allows mice to explore their surroundings and detect objects in their immediate vicinity.
Muscle Physiology: Contraction Mechanisms and Energy Sources
Muscle contraction is a complex physiological process that involves the interaction of contractile proteins, the generation of electrical signals, and the consumption of energy.
At the molecular level, contraction is driven by the sliding of actin and myosin filaments within muscle fibers. This process is triggered by the release of calcium ions, which bind to troponin and tropomyosin, exposing binding sites on actin. Myosin heads then attach to actin, forming cross-bridges, and pull the filaments past each other, shortening the muscle fiber.
ATP (adenosine triphosphate) is the primary energy source for muscle contraction. ATP provides the energy needed for the myosin heads to detach from actin and reset for the next cycle. Muscles obtain ATP from several sources, including:
- Creatine phosphate
- Glycolysis
- Oxidative phosphorylation
The relative contribution of each energy source depends on the intensity and duration of muscle activity. For short bursts of high-intensity activity, creatine phosphate is the primary fuel. For longer-duration activities, glycolysis and oxidative phosphorylation become increasingly important.
The muscular system provides the static framework, but the muscular system is what brings the mouse's body to life. It translates neural impulses into movement, maintains posture, and even contributes to vital functions like digestion. Understanding the intricacies of this system is crucial for interpreting a wide range of experimental data, from behavioral studies to investigations of muscular dystrophy.
Now, we turn our attention to the control center that orchestrates all of these functions and more: the nervous system. In the mouse, as in all mammals, this complex network is responsible for receiving, processing, and transmitting information throughout the body, allowing the animal to respond to its environment and maintain internal homeostasis.
Nervous System: The Central Command Center
The nervous system of the mouse, like that of other mammals, is a highly sophisticated information-processing network. It allows the animal to perceive its surroundings, coordinate movements, and regulate a myriad of internal functions essential for survival. Understanding its organization and function is paramount for interpreting many types of experimental data.
Central and Peripheral Components: An Overview
The mouse nervous system is broadly divided into two main components: the central nervous system (CNS) and the peripheral nervous system (PNS).
The CNS, consisting of the brain and spinal cord, acts as the primary processing center, receiving sensory input, generating motor commands, and mediating higher-level cognitive functions.
The PNS, on the other hand, comprises all the nerves and ganglia located outside of the brain and spinal cord. Its role is to connect the CNS to the rest of the body, relaying sensory information from the periphery to the CNS and carrying motor commands from the CNS to muscles and glands.
The Brain: A Tripartite Structure
The brain, the control center of the nervous system, is a complex organ with distinct regions that specialize in different functions. In the mouse, as in other mammals, the brain can be broadly divided into three major parts: the cerebrum, the cerebellum, and the brainstem.
Cerebrum: The Seat of Cognition and Sensory Processing
The cerebrum is the largest part of the brain and is responsible for higher-level cognitive functions such as learning, memory, and decision-making. It is also involved in processing sensory information from the eyes, ears, nose, and other sensory organs. The cerebral cortex, the outer layer of the cerebrum, is highly folded, increasing its surface area and allowing for greater information processing capacity.
Cerebellum: Master of Coordination and Motor Control
The cerebellum, located at the back of the brain, plays a crucial role in coordinating movements and maintaining balance. It receives input from the cerebrum, spinal cord, and sensory organs, and uses this information to fine-tune motor commands, ensuring smooth and accurate movements. Damage to the cerebellum can result in ataxia, a condition characterized by impaired coordination and balance.
Brainstem: The Foundation of Vital Functions
The brainstem, located at the base of the brain, connects the cerebrum and cerebellum to the spinal cord. It is responsible for regulating vital functions such as breathing, heart rate, blood pressure, and sleep-wake cycles. The brainstem also contains many important cranial nerve nuclei, which control functions such as eye movement, facial expression, and swallowing.
Spinal Cord: The Information Highway
The spinal cord is a long, cylindrical structure that extends from the brainstem down the back. It serves as a major relay station for signals traveling between the brain and the body. Sensory information from the periphery is transmitted to the brain via ascending tracts in the spinal cord, while motor commands from the brain are transmitted to muscles and glands via descending tracts.
The spinal cord also contains local circuits that mediate reflexes, such as the withdrawal reflex in response to pain.
Peripheral Nerves: Sensory and Motor Pathways
The peripheral nervous system (PNS) consists of all the nerves that lie outside the central nervous system (CNS).
These nerves act as communication lines, relaying sensory information to the CNS and carrying motor commands from the CNS to the rest of the body.
Sensory nerves transmit information about touch, temperature, pain, and other sensations from the skin, muscles, and internal organs to the CNS.
Motor nerves transmit signals from the CNS to muscles and glands, causing them to contract or secrete hormones. The PNS also includes the autonomic nervous system, which regulates involuntary functions such as heart rate, digestion, and sweating.
The intricate network of the nervous system allows the mouse to process information and react accordingly. However, the body also needs a robust support system to sustain its high metabolism and physical activity. Two vital systems work in concert to ensure every cell receives the oxygen and nutrients it needs: the circulatory and respiratory systems.
Circulatory and Respiratory Systems: Essential Life Support
The circulatory and respiratory systems are indispensable for maintaining life. They work synergistically to deliver oxygen and nutrients to tissues. These systems also remove waste products. In mice, as in other mammals, their efficient operation is critical for supporting a high metabolic rate and active lifestyle.
The Circulatory System: A Network of Life
The circulatory system, also known as the cardiovascular system, is responsible for transporting blood throughout the body. This network ensures that oxygen, nutrients, hormones, and immune cells reach every tissue. It also carries away waste products like carbon dioxide.
The Heart: The Pumping Engine
The mouse heart is a four-chambered organ, similar to the human heart. It consists of two atria and two ventricles. The right side of the heart receives deoxygenated blood from the body and pumps it to the lungs for oxygenation. The left side receives oxygenated blood from the lungs and pumps it to the rest of the body. The heart's rhythmic contractions, controlled by specialized cardiac muscle cells, ensure a continuous flow of blood.
Blood Vessels: Highways of the Body
The blood vessels form a complex network that distributes blood throughout the mouse's body. Arteries carry oxygenated blood away from the heart, branching into smaller arterioles that deliver blood to capillaries. Veins carry deoxygenated blood back to the heart. Capillaries are tiny, thin-walled vessels that facilitate the exchange of oxygen, nutrients, and waste products between the blood and surrounding tissues.
Blood: The Fluid of Life
Mouse blood consists of plasma, red blood cells (erythrocytes), white blood cells (leukocytes), and platelets (thrombocytes). Red blood cells contain hemoglobin, a protein that binds to oxygen and transports it from the lungs to the tissues. White blood cells are crucial for the immune system, defending the body against infection. Platelets are essential for blood clotting, preventing excessive bleeding.
The Respiratory System: The Breath of Life
The respiratory system facilitates gas exchange, allowing the mouse to take in oxygen and expel carbon dioxide. This process is essential for cellular respiration, the metabolic process that generates energy.
Lungs: Sites of Gas Exchange
The mouse lungs are paired organs located in the thoracic cavity. They consist of numerous tiny air sacs called alveoli. Alveoli are surrounded by capillaries, allowing for efficient gas exchange between the air and the blood. Oxygen diffuses from the alveoli into the blood, while carbon dioxide diffuses from the blood into the alveoli to be exhaled.
Trachea and Bronchi: Airways to the Lungs
The trachea, or windpipe, is a cartilaginous tube that carries air from the nose and mouth to the lungs. The trachea divides into two main bronchi, each leading to one lung. Within the lungs, the bronchi further branch into smaller bronchioles, eventually leading to the alveoli.
Diaphragm: The Breathing Muscle
The diaphragm is a large, dome-shaped muscle located at the base of the thoracic cavity. It plays a crucial role in breathing. During inhalation, the diaphragm contracts and flattens, increasing the volume of the thoracic cavity and drawing air into the lungs. During exhalation, the diaphragm relaxes, decreasing the volume of the thoracic cavity and forcing air out of the lungs.
The seamless orchestration of oxygen and nutrient delivery by the circulatory and respiratory systems sets the stage for the next act: processing these vital inputs and eliminating waste. The digestive and urogenital systems take center stage here, ensuring the mouse can extract energy from its food and rid itself of metabolic byproducts. Let's delve into the intricacies of these crucial systems.
Digestive and Urogenital Systems: Processing and Elimination
The digestive and urogenital systems are essential for maintaining internal homeostasis in the mouse. The digestive system breaks down food to extract nutrients and energy. The urogenital system filters waste from the blood and regulates reproductive functions. Understanding their functions is vital for comprehensive knowledge of mouse anatomy.
The Digestive System: From Ingestion to Absorption
The digestive system of the mouse is a complex assembly line. Each organ plays a vital role in breaking down food and absorbing essential nutrients. Let's explore this system in detail.
Oral Cavity and Esophagus: The Entry Point
Digestion begins in the oral cavity, where food is ingested and mechanically broken down by the teeth. Saliva, secreted by salivary glands, initiates the chemical digestion of carbohydrates.
The chewed food then travels down the esophagus, a muscular tube that connects the oral cavity to the stomach. Peristaltic contractions propel the food bolus towards its next destination.
Stomach: Acidic Digestion
The stomach is a J-shaped organ where further digestion takes place. Gastric glands in the stomach lining secrete hydrochloric acid and enzymes like pepsin. These substances break down proteins and kill bacteria.
The stomach's muscular walls churn and mix the food. This creates a semi-digested mixture called chyme.
Intestines: The Hub of Nutrient Absorption
The intestines are divided into the small and large intestines. The small intestine is the primary site for nutrient absorption. Its inner lining is covered with villi and microvilli, which increase the surface area for absorption. Enzymes from the pancreas and bile from the liver aid in the digestion of fats, carbohydrates, and proteins.
The large intestine absorbs water and electrolytes from the remaining undigested material. It forms feces, which are then eliminated from the body through the rectum and anus.
Liver and Pancreas: Essential Accessory Organs
The liver and pancreas are accessory digestive organs. They secrete substances that aid in digestion. The liver produces bile, which emulsifies fats, making them easier to digest. The pancreas secretes digestive enzymes and bicarbonate. The bicarbonate neutralizes the acidic chyme entering the small intestine.
The Urogenital System: A Basic Overview
The urogenital system encompasses both the urinary and reproductive systems. These systems are closely linked, particularly in males. The urinary system filters waste from the blood and excretes it as urine. The reproductive system is responsible for producing gametes and facilitating reproduction.
Urinary System
The urinary system consists of the kidneys, ureters, bladder, and urethra. The kidneys filter waste products from the blood. They regulate electrolyte balance, and produce urine. The urine is transported to the bladder via the ureters. The bladder stores urine until it is excreted through the urethra.
Reproductive System
The reproductive system differs significantly between male and female mice. In males, the testes produce sperm and testosterone. The sperm are transported through the vas deferens and ejaculated through the penis. In females, the ovaries produce eggs and hormones like estrogen and progesterone. The eggs travel through the fallopian tubes to the uterus, where fertilization and embryonic development occur.
The previous sections highlighted the intricate systems working in concert to sustain life within the mouse. Now, let's zoom in for a detailed exploration of the individual workhorses behind these systems. We will examine the architecture and function of the mouse's vital organs, understanding how their unique designs contribute to overall health and well-being.
Vital Organs: A Detailed Examination
A comprehensive understanding of mouse anatomy requires a deep dive into the major organs. Each organ possesses a unique structure intricately linked to its function. Let's explore the heart, lungs, liver, kidneys, brain, and intestines, revealing their specific roles within the mouse's physiology.
The Heart: A Pumping Marvel
The heart, a muscular pump, is the central component of the circulatory system. Its primary function is to circulate blood throughout the body, delivering oxygen and nutrients to tissues and removing waste products.
Chambers, Valves, and Blood Flow
The mouse heart, like that of other mammals, is composed of four chambers: two atria and two ventricles. The atria receive blood returning from the body and lungs, while the ventricles pump blood out to the lungs and the rest of the body.
Valves within the heart ensure unidirectional blood flow. The tricuspid valve and mitral valve separate the atria and ventricles on the right and left sides, respectively. The pulmonary valve and aortic valve control blood flow out of the ventricles.
This coordinated system of chambers and valves ensures efficient and continuous blood circulation. Disruption of any of these components can lead to cardiovascular dysfunction.
The Lungs: Orchestrating Gas Exchange
The lungs are the primary organs of the respiratory system, responsible for gas exchange between the air and the blood. Their unique structure maximizes the surface area available for this crucial process.
Alveoli and Gas Exchange Efficiency
The lungs consist of millions of tiny air sacs called alveoli. These alveoli are surrounded by a dense network of capillaries. It is within the alveoli that oxygen diffuses from the inhaled air into the blood, while carbon dioxide diffuses from the blood into the alveoli to be exhaled.
The thin walls of the alveoli and capillaries, combined with the vast surface area, facilitate efficient gas exchange. The efficiency of this exchange is vital for maintaining oxygen levels and removing carbon dioxide from the body.
The Liver: Metabolic Hub and Detoxification Center
The liver is a multifunctional organ playing a crucial role in metabolism, detoxification, and storage. Its diverse functions are essential for maintaining homeostasis.
Metabolic Functions and Detoxification
The liver is involved in carbohydrate, protein, and lipid metabolism. It synthesizes essential proteins, such as albumin and clotting factors. It also stores glycogen, a form of glucose, for energy.
The liver also plays a vital role in detoxification. It filters toxins from the blood and metabolizes drugs and alcohol, converting them into less harmful substances that can be excreted.
The Kidneys: Filtration and Waste Removal Experts
The kidneys are responsible for filtering waste products from the blood and regulating fluid and electrolyte balance. Their intricate structure allows for efficient waste removal while retaining essential nutrients.
Filtration and Waste Removal
Each kidney contains millions of nephrons, the functional units of the kidney. Nephrons filter blood, reabsorbing essential substances like glucose, amino acids, and water, while excreting waste products such as urea and creatinine.
The kidneys also play a crucial role in regulating blood pressure and producing hormones, such as erythropoietin, which stimulates red blood cell production.
The Brain: The Central Processing Unit
The brain, the control center of the nervous system, is responsible for processing information, coordinating movement, and regulating bodily functions. Its complex structure allows for a wide range of cognitive and behavioral capabilities.
Regional Specialization and Neural Pathways
The mouse brain, like that of other mammals, is divided into several regions, each with specialized functions. The cerebrum is responsible for higher-level cognitive functions, such as learning and memory. The cerebellum coordinates movement and balance. The brainstem regulates vital functions like breathing and heart rate.
Neural pathways connect different regions of the brain and transmit information throughout the nervous system.
The Intestines: Nutrient Absorption and Gut Microbiome
The intestines are responsible for digesting food, absorbing nutrients, and eliminating waste. The gut microbiome, a complex community of microorganisms residing in the intestines, plays a vital role in digestion and immunity.
Absorption Mechanisms and Gut Microbiome
The small intestine is the primary site of nutrient absorption. Its inner lining is folded into villi and microvilli, increasing the surface area available for absorption.
The gut microbiome aids in digestion by breaking down complex carbohydrates and synthesizing vitamins. It also plays a crucial role in immune system development and protecting against pathogens. Disruptions in the gut microbiome have been linked to a variety of health problems.
The previous sections highlighted the intricate systems working in concert to sustain life within the mouse. Now, let's turn our attention to how the mouse perceives the world around it. We will explore the fascinating realm of sensory systems, understanding how these organs act as the interface between the mouse and its environment.
Sensory Systems: Interacting with the Environment
A mouse's survival hinges on its ability to accurately perceive and interpret its surroundings. This capability is facilitated by a suite of sophisticated sensory organs, each uniquely adapted to detect specific stimuli. These senses—sight, hearing, smell, taste, and touch—work together to provide the mouse with a comprehensive understanding of its environment, enabling it to navigate, find food, avoid predators, and interact with other mice.
An Overview of Sensory Perception in Mice
Mice, being primarily nocturnal creatures, rely heavily on senses other than sight. Their sensory perception is intricately linked to their ecological niche, where detecting subtle changes in their environment is crucial for survival. While their visual acuity may not be as sharp as diurnal animals, their senses of smell and hearing are exceptionally well-developed.
These senses provide detailed information about their surroundings, allowing them to thrive in low-light conditions.
The Dominance of Olfaction: A World of Scents
Perhaps the most crucial sense for a mouse is its sense of smell, or olfaction. Mice possess an incredibly sensitive olfactory system, allowing them to detect and discriminate between a vast array of odors.
This ability is vital for locating food sources, identifying potential predators, recognizing other individuals, and navigating their environment. Scent marking plays a significant role in mouse communication, with individuals depositing urine and other secretions to establish territories, attract mates, and convey social information.
Vomeronasal Organ and Pheromonal Communication
A specialized structure called the vomeronasal organ (VNO), also known as Jacobson's organ, is dedicated to detecting pheromones. Pheromones are chemical signals that trigger specific behavioral responses in other individuals of the same species. Through the VNO, mice can detect pheromones related to sex, social status, and alarm, influencing their reproductive behavior, social interactions, and predator avoidance strategies.
Auditory Acuity: Hearing the Unheard
Mice have a remarkable sense of hearing, capable of detecting a wide range of frequencies, including ultrasonic sounds inaudible to humans. This ability is essential for detecting predators, communicating with other mice, and navigating their environment.
Their large ears help capture and amplify faint sounds, while their sophisticated inner ear allows them to discriminate between subtle differences in sound.
Ultrasonic Vocalizations: A Language Beyond Human Hearing
Mice communicate extensively using ultrasonic vocalizations (USVs), complex calls that convey a variety of information. These USVs are used in a range of social contexts, including courtship, pup-mother interactions, and aggressive encounters. Researchers are increasingly interested in understanding the complexities of mouse USVs, as they may provide insights into their emotional states and social dynamics.
Vision: More Than Meets the Eye
While not their primary sense, vision still plays an important role in a mouse's perception of the world. Mice have relatively poor visual acuity compared to humans, but they can detect movement and perceive some colors, particularly in the ultraviolet range.
Their eyes are positioned laterally, providing a wide field of view that is advantageous for detecting predators.
Ultraviolet Vision: A Hidden World
The ability to see ultraviolet (UV) light allows mice to perceive patterns and signals that are invisible to humans. For example, they can use UV cues to track urine trails, identify food sources, and assess the reproductive status of other mice. This UV vision provides them with valuable information about their environment that would otherwise be undetectable.
Touch and Taste: Close Encounters
The sense of touch is important for exploring their immediate surroundings. Vibrissae, or whiskers, are highly sensitive tactile organs that provide mice with detailed information about the surfaces they encounter. These whiskers are particularly useful for navigating in dark or confined spaces. The sense of taste, while less crucial than smell, allows mice to discriminate between different food sources and avoid potentially harmful substances.
In conclusion, the sensory systems of the mouse represent a sophisticated array of adaptations that enable it to thrive in its environment. The interplay of olfaction, audition, vision, touch, and taste provides a comprehensive understanding of the world, allowing the mouse to navigate, find food, avoid predators, and interact with other members of its species.
The previous sections highlighted the intricate systems working in concert to sustain life within the mouse. Now, let's shift gears and discuss the pivotal role the mouse plays in scientific discovery. We will now explore the significance of mouse models in research, focusing on how these small creatures contribute to advancements in understanding human biology and disease.
Mouse Models in Research: The Power of Laboratory Mice
Laboratory mice have become indispensable tools in modern biomedical research, serving as powerful models for understanding human biology and disease. Their relatively short lifespans, ease of breeding, and genetic similarity to humans make them ideal subjects for studying a wide range of conditions. From cancer and cardiovascular disease to neurological disorders and infectious diseases, mice have played a crucial role in unraveling disease mechanisms and developing new therapies.
The Significance of Laboratory Mice in Scientific Advancement
The widespread use of laboratory mice is rooted in several key advantages. First, mice share a significant portion of their genome with humans, making them susceptible to similar diseases and physiological processes. This genetic similarity allows researchers to extrapolate findings from mouse studies to humans with a reasonable degree of confidence.
Second, mice are relatively easy to maintain and breed in laboratory settings, enabling researchers to conduct large-scale studies with controlled variables. This is critical for isolating specific factors that contribute to disease development and progression.
Third, the short lifespan of mice allows researchers to observe the effects of interventions over multiple generations within a relatively short period. This is particularly valuable for studying age-related diseases and the long-term consequences of genetic manipulations.
Finally, the ethical considerations surrounding the use of mice in research are generally less stringent than those associated with larger animals or human subjects, facilitating the exploration of complex biological questions.
Genetic Manipulations and Their Impact on Anatomic Studies
One of the most powerful aspects of using laboratory mice in research is the ability to manipulate their genes. Genetic engineering techniques allow researchers to create mouse models that mimic specific human diseases or exhibit altered physiological functions. These models provide invaluable insights into the genetic and molecular mechanisms underlying disease pathogenesis.
Transgenic Mice
Transgenic mice are created by introducing foreign DNA into the mouse genome, resulting in the expression of a new gene or the overexpression of an existing gene.
This approach can be used to study the effects of specific genes on development, physiology, and disease susceptibility.
Knockout Mice
Knockout mice, on the other hand, have a specific gene inactivated or "knocked out." This allows researchers to assess the role of that gene in various biological processes.
By studying the phenotypic consequences of gene knockout, researchers can gain a deeper understanding of gene function and its contribution to normal physiology and disease.
The Impact on Anatomic Studies
Genetic manipulations have had a profound impact on anatomic studies. By creating mouse models with specific anatomical abnormalities, researchers can study the development and progression of diseases that affect organ structure and function.
For example, mouse models of muscular dystrophy, characterized by progressive muscle wasting, have provided valuable insights into the structural and molecular changes that occur in affected muscles. Similarly, mouse models of cystic fibrosis, which causes severe lung damage, have allowed researchers to study the anatomical changes in the lungs and develop new therapies to improve lung function.
Furthermore, genetic manipulations can be used to study the anatomical consequences of altered gene expression in specific tissues or organs. By targeting gene expression to specific cell types, researchers can dissect the complex interplay between genes and anatomical structures, providing a deeper understanding of the relationship between genotype and phenotype.
The previous sections highlighted the intricate systems working in concert to sustain life within the mouse. Now, let's shift gears and discuss the pivotal role of anatomical terminology in research. It's a universal, shared language across scientific disciplines to precisely describe anatomical structures and relationships. This section will delve into the importance of mastering this terminology, focusing on its application to mice and other organisms commonly used in anatomical studies.
Anatomical Terminology: A Shared Language
In the realm of anatomical studies, precise and consistent communication is paramount. This is where anatomical terminology steps in, acting as a standardized language that transcends geographical boundaries and disciplinary silos. It provides a framework for accurately describing the location, orientation, and relationships of anatomical structures, ensuring clarity and avoiding ambiguity.
The Importance of Standardized Terms
Imagine trying to describe the location of a specific blood vessel without a common reference point. Confusion would inevitably arise, hindering effective communication and potentially leading to errors. Anatomical terminology resolves this issue by providing a set of universally accepted terms, based primarily on Latin and Greek roots.
This standardization ensures that researchers worldwide can understand each other, regardless of their native language or specific field of expertise. This shared language is critical for accurate data interpretation, effective collaboration, and the advancement of scientific knowledge.
Key Anatomical Terms and Concepts
Several fundamental terms form the bedrock of anatomical description. Understanding these terms is crucial for navigating anatomical literature and engaging in meaningful discussions.
Directional Terms
Directional terms describe the relative position of structures in relation to each other. These terms are always used with respect to the anatomical position, which is the standard reference point for anatomical descriptions.
- Anterior (cranial): Toward the head or front of the body.
- Posterior (caudal): Toward the tail or back of the body.
- Superior (dorsal): Toward the upper part of the body (or back in quadrupeds).
- Inferior (ventral): Toward the lower part of the body (or belly in quadrupeds).
- Medial: Toward the midline of the body.
- Lateral: Away from the midline of the body.
- Proximal: Closer to the point of attachment or origin.
- Distal: Farther from the point of attachment or origin.
Planes of Section
Planes of section are imaginary flat surfaces that pass through the body, allowing for visualization of internal structures from different perspectives.
- Sagittal plane: Divides the body into right and left portions. A midsagittal plane divides the body into equal halves.
- Frontal (coronal) plane: Divides the body into anterior and posterior portions.
- Transverse (horizontal) plane: Divides the body into superior and inferior portions.
Terms of Movement
Understanding terms of movement helps to describe the actions of muscles and joints.
- Flexion: Decreasing the angle between two body parts.
- Extension: Increasing the angle between two body parts.
- Abduction: Moving a body part away from the midline.
- Adduction: Moving a body part toward the midline.
- Rotation: Turning a body part around its longitudinal axis.
Applying Terminology to Mice and Other Organisms
While the fundamental anatomical terms remain consistent across species, their application may vary slightly depending on the organism's body plan and posture. For example, the terms "dorsal" and "superior" are interchangeable in humans, but "dorsal" refers to the back of a quadruped, while "superior" refers to the upper part of its body.
When studying mouse anatomy, it's essential to remember that mice are quadrupedal. Therefore, the terms anterior/posterior and superior/inferior are often used in conjunction with cranial/caudal and dorsal/ventral, respectively, to provide more precise descriptions.
Resources for Learning Anatomical Terminology
Several resources are available to aid in mastering anatomical terminology. Anatomical atlases, textbooks, and online dictionaries can provide comprehensive definitions and illustrations of anatomical terms. Interactive tools and virtual dissection platforms can also be valuable for visualizing anatomical structures in three dimensions and practicing anatomical descriptions.
By investing time and effort in learning anatomical terminology, researchers can enhance their ability to communicate effectively, interpret anatomical data accurately, and contribute meaningfully to the advancement of scientific knowledge.
Video: Mouse Anatomy: Unveiling Secrets You Never Knew! [Guide]
Frequently Asked Questions About Mouse Anatomy
Here are some common questions readers have after learning about the fascinating anatomy of mice. We hope these answers provide further clarity.
Why are a mouse's whiskers so important?
A mouse's whiskers are highly sensitive sensory organs. They help the mouse navigate its environment, detect air currents, and identify objects, especially in the dark. This sensitivity is crucial for survival.
How does a mouse's skeletal structure differ from a human's?
While both mice and humans are mammals, their skeletal structures have distinct differences. Mice possess a more flexible spine, allowing for greater agility. Their bone density is also lower due to their smaller size. Understanding these differences is key to comprehending mouse anatomy.
What is the purpose of the Harderian gland in mice?
The Harderian gland, located behind the eye, produces a secretion containing lipids and porphyrins. This secretion lubricates the eye and helps in thermoregulation. It also plays a role in social signaling and communication within the mouse community.
How does the high surface area to volume ratio of a mouse affect its physiology?
Due to their small size, mice have a high surface area to volume ratio. This leads to rapid heat loss, requiring a high metabolic rate to maintain body temperature. This also affects their respiration and overall energy needs concerning mouse anatomy.
Well, that's a wrap on our little journey through mouse anatomy! Hopefully, you learned a thing or two. Now go forth and amaze your friends with your newfound knowledge of tiny rodent innards. Until next time!