Molecular vs Ionic Compound: Key Differences
The fundamental distinction between molecular vs ionic compound lies in their formation and properties, affecting how they interact in chemical reactions and various applications; specifically, molecular compounds, often associated with covalent bonds and shared electrons, exhibit lower melting and boiling points compared to ionic compounds. The characteristics of molecular compounds are critical in understanding organic chemistry, which largely involves molecules like hydrocarbons, while the strong electrostatic forces in ionic compounds explain their crystalline structures and high solubility in polar solvents like water. Predicting whether a substance will form a molecular vs ionic compound is often guided by the electronegativity differences between the elements involved, a concept thoroughly explored in the periodic table. The International Union of Pure and Applied Chemistry (IUPAC) provides standardized nomenclature rules that help differentiate and name these two types of compounds, ensuring clarity in scientific communication.
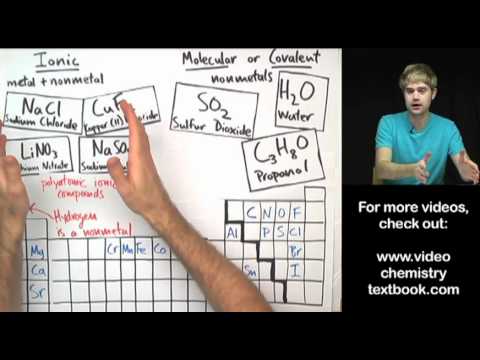
Image taken from the YouTube channel Tyler DeWitt , from the video titled Ionic vs. Molecular .
The World Held Together: Ionic vs. Covalent Bonds
Chemical bonds are the very scaffolding of the universe, the fundamental forces that dictate how atoms interact and assemble into the myriad molecules that constitute all matter around us. Without these connections, the world as we know it would simply cease to exist.
Among the diverse types of chemical bonds, ionic and covalent bonds stand out as the two primary categories, representing distinct mechanisms by which atoms achieve stability.
Two Primary Categories of Chemical Bonds
Ionic bonds arise from the transfer of electrons between atoms, resulting in the formation of charged ions that are attracted to one another. Covalent bonds, on the other hand, involve the sharing of electrons between atoms, creating a stable association through mutual attraction to the shared electron cloud.
The Purpose of this Comparison
This article aims to provide a comprehensive comparison and contrast of ionic and covalent bonds, elucidating their key differences and similarities.
By examining their formation, characteristics, and the resulting properties of the compounds they form, we can gain a deeper appreciation for the diverse and intricate world of chemical bonding.
Chemical Bonds and Predictive Power
Understanding the nuances of ionic and covalent bonds is not merely an academic exercise. It is a powerful tool that allows us to predict and explain the physical and chemical properties of substances.
From melting points and boiling points to conductivity and solubility, the type of chemical bond present in a compound dictates its behavior and its interactions with other substances.
Essential Chemistry Concepts: Building Blocks for Understanding Bonds
Before diving into the intricacies of ionic and covalent bonds, it's crucial to establish a firm foundation in some essential chemistry concepts. These concepts act as the fundamental building blocks that allow us to understand why and how these bonds form. We'll explore electron configuration, the octet rule, electronegativity, and Lewis structures, elucidating their importance in the context of chemical bonding.
Electron Configuration and the Octet Rule
Atoms are most stable when their outermost electron shell, also known as the valence shell, is full. This stability is mirrored in the noble gases, which possess complete valence shells and exhibit remarkable inertness.
The desire to attain a stable, noble gas-like electron configuration drives atoms to form chemical bonds. Atoms will either gain, lose, or share electrons to achieve this stability.
The octet rule states that atoms tend to gain, lose, or share electrons in order to achieve a full valence shell containing eight electrons. Hydrogen is an exception, needing only two electrons to fill its valence shell.
Electronegativity: The Pull of an Atom
Electronegativity is a measure of an atom's ability to attract electrons towards itself within a chemical bond. It's a fundamental property that dictates the type of bond that will form between two atoms.
The concept of electronegativity was championed by Linus Pauling, who developed the widely used Pauling scale. This scale assigns numerical values to elements, allowing for quantitative comparison of their electron-attracting abilities.
Electronegativity generally increases across a period (from left to right) and decreases down a group in the periodic table. This trend is due to factors like increasing nuclear charge and atomic size. By consulting the periodic table, chemists can predict the relative electronegativity of different elements.
Lewis Structures: Visualizing Valence Electrons
Lewis structures are diagrams that visually represent the valence electrons of atoms within a molecule. They use dots to depict valence electrons and lines to represent shared electron pairs in covalent bonds.
Lewis structures are powerful tools for predicting how atoms will bond, how many bonds they will form, and the overall structure of a molecule. They allow us to visualize the distribution of electrons and assess the stability of different bonding arrangements.
The concept of Lewis structures is attributed to Gilbert N. Lewis, a pioneer in the field of chemical bonding. His method revolutionized the way chemists understand and represent molecular structures, providing a simple, yet effective visual tool to conceptualize the electronic structure of molecules.
Ionic Bonds: Electron Transfer and Electrostatic Attraction
Having established the foundational concepts of electronegativity and the octet rule, we can now explore the specifics of ionic bond formation. Ionic bonds represent a fundamental interaction in chemistry, driven by the transfer of electrons and the subsequent electrostatic attraction between oppositely charged ions.
The Genesis of Ionic Bonds: Electron Transfer
Ionic bonds arise between atoms exhibiting a significant difference in electronegativity. This difference is crucial, as it dictates the complete transfer of one or more electrons from one atom to another. The atom that loses electrons becomes a positively charged ion, known as a cation.
Conversely, the atom that gains electrons transforms into a negatively charged ion, termed an anion.
This electron transfer is not arbitrary; it is driven by the pursuit of a stable electron configuration, often resembling that of a noble gas.
Characteristics of Ionic Interactions
The hallmark of an ionic bond is the electrostatic attraction between the resulting cation and anion. This attraction, governed by Coulomb's Law, is powerful and far-reaching, dictating many of the properties observed in ionic compounds.
Ionic bonds typically form between a metal and a nonmetal. Metals, with their low electronegativity, readily lose electrons to form cations, while nonmetals, possessing high electronegativity, readily gain electrons to form anions.
It is important to note that ionic compounds do not exist as discrete molecules. Instead, they are represented by a formula unit, which signifies the simplest ratio of ions in the compound. For example, NaCl represents one sodium ion (Na+) for every chloride ion (Cl-).
The Crystal Lattice: A Symphony of Ions
Ionic compounds arrange themselves in a highly ordered, three-dimensional structure called a crystal lattice.
Within this lattice, each ion is surrounded by ions of opposite charge, maximizing the electrostatic attraction and minimizing repulsion.
This arrangement results in a stable and energetically favorable structure. The specific arrangement of ions within the lattice depends on the size and charge of the constituent ions.
Properties Dictated by Strong Bonds
The strong electrostatic forces within the crystal lattice give rise to several characteristic properties of ionic compounds.
High melting and boiling points are a direct consequence of the energy required to overcome the strong interionic forces.
Ionic compounds are generally poor conductors of electricity in the solid state. This is because the ions are fixed in their positions within the lattice and are unable to move freely.
However, when dissolved in water, ionic compounds dissociate into individual ions, allowing the solution to conduct electricity effectively. These solutions are known as electrolytes.
The solubility of ionic compounds in polar solvents such as water is another important property. Water molecules, being polar, can effectively solvate the ions, disrupting the crystal lattice and allowing the compound to dissolve.
Exemplifying Ionic Bonding
Several common compounds serve as excellent examples of ionic bonding in action.
Sodium Chloride (NaCl), common table salt, is a quintessential ionic compound. The strong electrostatic attraction between Na+ and Cl- ions gives rise to its characteristic crystalline structure and high melting point.
Magnesium Oxide (MgO), utilized in various industrial applications, also exhibits strong ionic bonding. The higher charges of the Mg2+ and O2- ions result in an even stronger electrostatic attraction, leading to an exceptionally high melting point.
Covalent Bonds: Sharing is Caring (Electrons, That Is)
Having explored the world of ionic bonds and the electron transfer mechanisms that define them, we now turn our attention to covalent bonds. Covalent bonds represent a different approach to achieving stable electron configurations. They involve the sharing of electron pairs between atoms, leading to the formation of molecules with distinct properties.
Formation: A Cooperative Approach
Covalent bonds typically form between atoms with similar electronegativity values. This contrasts sharply with ionic bonds, which arise from large electronegativity differences.
Instead of one atom completely taking electrons from another, atoms involved in covalent bonding cooperate by sharing electrons. This sharing allows each atom to achieve a stable electron configuration, often satisfying the octet rule.
Characteristics: Building Blocks of Molecules
Unlike ionic compounds which form extended crystal lattices, covalent bonds create discrete units called molecules.
These molecules consist of two or more atoms held together by shared electrons. Covalent bonds predominantly occur between two nonmetal atoms.
Types of Covalent Bonds: Polarity Matters
Covalent bonds aren't all created equal; they can be further categorized based on the equality of electron sharing:
Polar Covalent Bonds
In polar covalent bonds, electrons are unequally shared between atoms. This occurs when there is a slight difference in electronegativity between the bonding atoms.
The atom with the higher electronegativity will have a partial negative charge (δ-), while the other atom will have a partial positive charge (δ+). This creates a dipole moment within the bond.
Nonpolar Covalent Bonds
In nonpolar covalent bonds, electrons are shared equally between atoms.
This typically happens when the atoms involved have identical or very similar electronegativity values. Diatomic molecules such as H₂, O₂, and Cl₂ exemplify nonpolar covalent bonds.
Properties: A World of Diversity
The properties of covalently bonded substances differ significantly from those of ionic compounds.
Generally, covalent compounds exhibit lower melting and boiling points compared to ionic compounds.
This is because the intermolecular forces between molecules are weaker than the electrostatic forces holding ions together in a crystal lattice.
Covalent compounds are generally poor conductors of electricity because there are no freely moving ions or electrons to carry a charge. Solubility varies depending on the polarity of the molecule and the solvent. "Like dissolves like" is a useful rule of thumb. Polar molecules tend to dissolve in polar solvents (like water), while nonpolar molecules dissolve in nonpolar solvents (like oil).
Examples: Illustrating Covalent Bonding
Several common molecules illustrate the principles of covalent bonding:
- Methane (CH₄): A nonpolar molecule where carbon shares electrons equally with four hydrogen atoms.
- Water (H₂O): A polar molecule where oxygen shares electrons unequally with two hydrogen atoms, resulting in a bent shape and a dipole moment.
- Carbon Dioxide (CO₂): A linear molecule with polar bonds, but due to its symmetry, the overall molecule is nonpolar.
- Ammonia (NH₃): Exhibits hydrogen bonding, a special type of intermolecular force that significantly affects its properties. This hydrogen bonding arises from the highly polar N-H bonds.
Bond Type and Molecular Properties: A Tangible Connection
Having explored the world of ionic and covalent bonds and the electron transfer/sharing mechanisms that define them, it is crucial to understand how these bonds dictate tangible molecular properties. The type of bond formed between atoms directly influences a molecule's polarity, which in turn affects intermolecular forces (IMFs). These IMFs ultimately govern a substance's physical characteristics and solubility. Let's examine this intricate connection.
Polarity and Dipole Moments
Polarity arises from the unequal sharing of electrons in covalent bonds due to differences in electronegativity between bonded atoms. This unequal sharing creates partial positive (δ+) and partial negative (δ-) charges on the atoms, resulting in a dipole moment.
The dipole moment is a vector quantity representing the magnitude and direction of the charge separation in a molecule. It is a crucial indicator of a molecule's overall polarity. Molecules with significant dipole moments are considered polar, while those with negligible or zero dipole moments are nonpolar.
The impact of polarity extends beyond individual molecules, profoundly affecting intermolecular interactions.
Intermolecular Forces (IMFs)
Intermolecular forces are attractive or repulsive forces between molecules. These forces are significantly weaker than intramolecular forces (ionic, covalent, and metallic bonds). But they are critical in determining the physical properties of substances. Several types of IMFs exist, each with varying strengths:
-
Van der Waals Forces:
These are weak, short-range forces arising from temporary fluctuations in electron distribution, leading to temporary dipoles.
They are present in all molecules, but more significant in nonpolar molecules.
-
Dipole-Dipole Interactions:
Occur between polar molecules due to the attraction between the positive end of one dipole and the negative end of another.
Stronger than Van der Waals forces.
-
Hydrogen Bonding:
A particularly strong type of dipole-dipole interaction that occurs when hydrogen is bonded to a highly electronegative atom (oxygen, nitrogen, or fluorine).
It results in a large partial positive charge on the hydrogen atom and strong attraction to a lone pair of electrons on another electronegative atom.
Hydrogen bonding significantly elevates boiling points and affects the structure of many biological molecules.
Influence on Physical Properties
IMFs profoundly impact a substance's physical properties, such as boiling point, melting point, viscosity, and surface tension. Stronger IMFs require more energy to overcome, leading to higher boiling and melting points.
For example, water (H₂O) has a relatively high boiling point due to strong hydrogen bonding between molecules. Similarly, viscosity (resistance to flow) is also affected by IMFs; substances with stronger IMFs tend to be more viscous.
Solubility and the "Like Dissolves Like" Principle
Solubility, the ability of a substance to dissolve in a solvent, is critically dependent on the intermolecular forces between the solute and the solvent. The general rule of thumb is "like dissolves like." This principle suggests that polar substances dissolve well in polar solvents, while nonpolar substances dissolve well in nonpolar solvents.
This occurs because polar solvents can effectively interact with and stabilize polar solutes through dipole-dipole interactions or hydrogen bonding. Nonpolar solvents, on the other hand, can only interact effectively with nonpolar solutes through Van der Waals forces.
For instance, water (polar) readily dissolves ionic compounds like sodium chloride (NaCl) and polar covalent compounds like ethanol (C₂H₅OH). Oil (nonpolar) does not dissolve in water, but it dissolves well in other nonpolar solvents such as hexane.
Understanding the interplay between bond types, molecular polarity, IMFs, and physical properties is crucial for predicting and explaining the behavior of chemical substances. These concepts are essential tools in chemistry, materials science, and other related fields.
Acids and Bases: An Application of Bond Understanding
Having explored the world of ionic and covalent bonds and the electron transfer/sharing mechanisms that define them, it is crucial to understand how these bonds dictate tangible molecular properties. The type of bond formed between atoms directly influences a molecule's polarity, which in turn governs its behavior in various chemical reactions. A prime example of this connection is observed in the behavior of acids and bases.
The Acidic Realm: Covalent Bonds and Ionization
Acids, at the molecular level, often consist of atoms connected by covalent bonds. Hydrogen chloride (HCl) and sulfuric acid (H₂SO₄) are exemplary cases.
These acids are molecular compounds. However, their distinctive characteristic lies in their ability to ionize when dissolved in water.
This ionization process involves the breaking of covalent bonds and the subsequent formation of ions. HCl, for instance, dissociates into H+ (hydronium) and Cl- ions in water.
The electronegativity difference between hydrogen and chlorine causes the H-Cl bond to be polarized, with a partial positive charge on the hydrogen atom. This polarization facilitates the separation of H+ ions in water.
The resulting hydronium ion (H₃O+) is what contributes to the acidic properties of the solution. The strength of an acid depends on the extent to which it ionizes in water; strong acids like HCl undergo nearly complete ionization, while weak acids ionize only partially.
The Basic Frontier: Ionic Compounds and Dissociation
Bases, in contrast to acids, are often ionic compounds. Sodium hydroxide (NaOH) and potassium hydroxide (KOH) exemplify this category.
These compounds readily dissociate into their constituent ions when introduced to water.
The ionic bonds holding the metal cation (Na+ or K+) and hydroxide anion (OH-) together are disrupted by the polar water molecules.
The strong electrostatic attraction between water and the ions causes them to separate, liberating hydroxide ions into the solution.
The presence of these hydroxide ions increases the pH of the solution, giving it basic properties. Strong bases like NaOH and KOH fully dissociate in water, releasing a high concentration of OH- ions.
Acids, Bases, and Water: A Balancing Act
Both acids and bases interact with water to produce ions, demonstrating the role of ionic and covalent bonds in their behavior. Acids, through covalent bond ionization, increase the concentration of H+ ions. Bases, often via ionic compound dissociation, increase the concentration of OH- ions.
These interactions highlight the delicate balance of hydrogen and hydroxide ions in aqueous solutions, a balance crucial for life and myriad chemical processes.
The strength of an acid or base is directly linked to the extent to which it can alter this equilibrium by either donating or accepting protons (H+), an exchange that underscores the profound influence of chemical bonding on macroscopic properties.
Video: Molecular vs Ionic Compound: Key Differences
Frequently Asked Questions
Why do ionic compounds have such high melting points compared to molecular compounds?
Ionic compounds have strong electrostatic forces between their ions, requiring a lot of energy (high temperatures) to break. Molecular compounds only have weaker intermolecular forces between their molecules, needing less energy to overcome. This is a key difference between a molecular vs ionic compound.
Do molecular compounds conduct electricity like ionic compounds sometimes do?
Generally, no. Molecular compounds are typically poor conductors of electricity because they don't contain freely moving ions or electrons. Ionic compounds can conduct electricity when dissolved in water or melted, as the ions become mobile. Therefore, conductivity is a significant distinction between molecular vs ionic compounds.
What does the term "molecule" actually mean in the context of an ionic compound?
Ionic compounds don't form discrete "molecules" in the same way that molecular compounds do. Instead, they form a continuous lattice structure of alternating positive and negative ions. While we write formulas like NaCl, it represents the ratio of ions, not an isolated molecule. This is different in a molecular vs ionic compound.
Are there exceptions to the "metal + nonmetal = ionic" rule?
Yes, there are exceptions. Some compounds formed between metals and nonmetals exhibit significant covalent character and properties more similar to molecular compounds, especially with elements exhibiting borderline metallic behavior. The electronegativity difference between the elements is key to determining if it is an ionic or a molecular vs ionic compound.
So, next time you're staring at a bottle of water (molecular) or sprinkling salt on your fries (ionic), remember the fundamental differences we've explored. Understanding the contrast between molecular vs ionic compounds really boils down to grasping how atoms share or transfer electrons, and that little bit of knowledge unlocks a whole new level of understanding about the world around us!