Mastering Methane: Valence Electrons Explained in Detail
Methane, a simple yet crucial hydrocarbon, plays a significant role in various scientific fields, including atmospheric chemistry. The United States Environmental Protection Agency (EPA) monitors methane emissions to understand their impact on global warming. Central to understanding methane's reactivity and stability is the concept of methane valence electrons. A Lewis structure visually represents these valence electrons, showing how carbon and hydrogen atoms share electrons to form stable bonds. The principles of quantum mechanics, specifically valence bond theory, further explain the distribution and behavior of these methane valence electrons. Scientists at the National Renewable Energy Laboratory (NREL) research methane conversion technologies, relying on a deep understanding of these fundamental electron interactions to improve efficiency and sustainability.
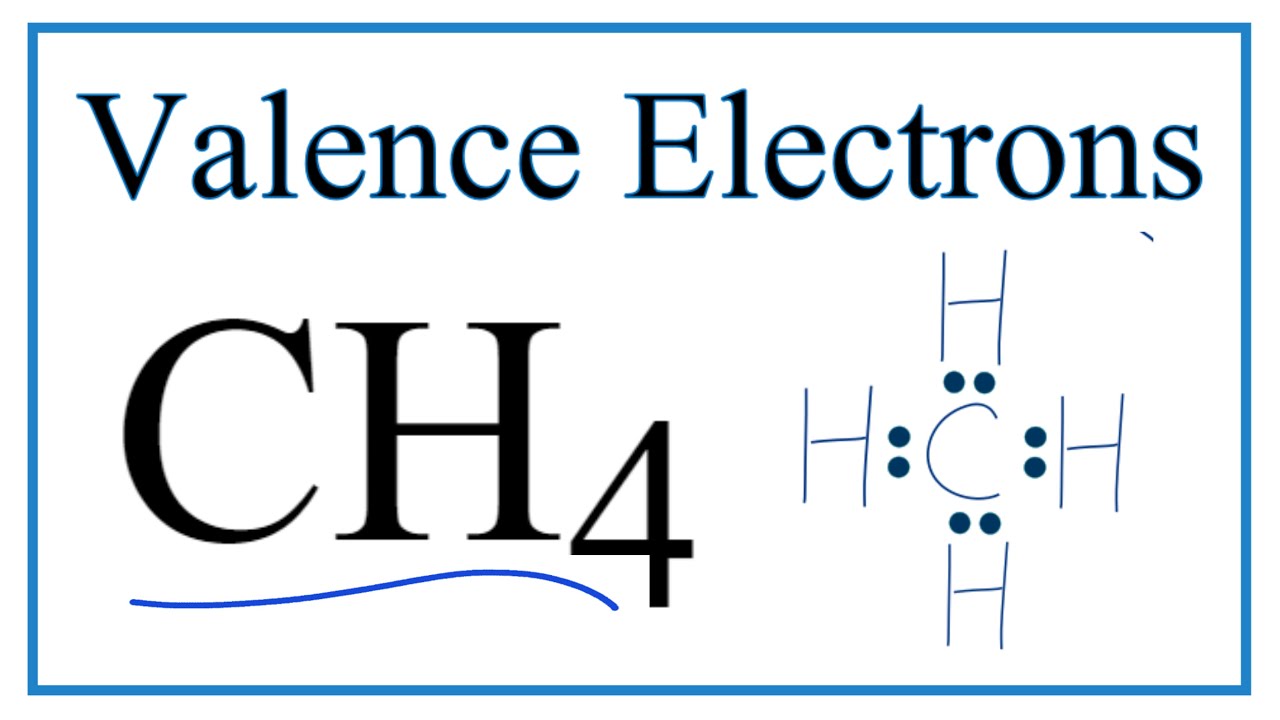
Image taken from the YouTube channel Wayne Breslyn (Dr. B.) , from the video titled How to Find the Valence Electrons for CH4 (Methane) .
Methane (CH4), a seemingly simple molecule composed of one carbon atom and four hydrogen atoms, is anything but ordinary. Its prevalence and significance in various fields, from energy production to atmospheric science, make it a crucial subject of study.
This introduction serves as a gateway to understanding the intricacies of methane, focusing on its fundamental structure and the nature of its chemical bonds. We will explore why a deep understanding of methane is essential, not just for chemistry students but for anyone interested in the world around them.
Methane: A Ubiquitous Compound
Methane is abundant in nature.
It is the primary component of natural gas, a major source of energy worldwide.
It's also released from various sources, including wetlands, agricultural activities, and the decomposition of organic matter.
Furthermore, methane is a potent greenhouse gas, playing a significant role in climate change.
Its presence in the atmosphere and its impact on global warming make it imperative to understand its behavior.
The Importance of Structural Understanding
Understanding the structure of methane is fundamental to grasping its properties and reactivity.
The arrangement of atoms in a molecule dictates how it interacts with other molecules.
This knowledge allows scientists to predict its behavior in different environments.
It also enables the development of new technologies for capturing and utilizing methane.
Without a solid grasp of methane's structure, many advanced concepts in chemistry and related fields would remain inaccessible.
Valence Electrons: The Architects of Methane's Properties
The properties of methane are largely determined by the behavior of its valence electrons.
Valence electrons are the outermost electrons of an atom that participate in chemical bonding.
The way carbon and hydrogen share their valence electrons dictates the shape and stability of the methane molecule.
By examining the role of valence electrons, we can begin to understand why methane behaves the way it does. This understanding allows us to appreciate its significance in a wide range of chemical processes.
Methane's properties are largely determined by the behavior of electrons, particularly those furthest from the nucleus. To understand how methane forms and behaves, we must first delve into the world of valence electrons, the architects of chemical bonds.
Deciphering Valence Electrons: The Key to Chemical Bonding
Valence electrons are not just any electrons; they are the outermost electrons of an atom. Their location dictates an element's chemical behavior and its ability to form bonds with other atoms. These electrons are the key players in the drama of chemical reactions, determining how atoms interact and combine to form molecules.
Defining Valence Electrons
Valence electrons reside in the outermost electron shell, also known as the valence shell, of an atom. This shell is the highest energy level that contains electrons. The number of valence electrons an atom possesses determines its chemical properties and how it interacts with other atoms to form chemical bonds.
It's important to distinguish valence electrons from core electrons, which are found in the inner shells and are generally not involved in bonding. Core electrons are tightly bound to the nucleus and do not participate in chemical reactions under normal circumstances.
The Role of Valence Electrons in Chemical Bonding
Atoms are most stable when their valence shells are full, typically with eight electrons (the octet rule). To achieve this stable configuration, atoms participate in chemical bonding, and valence electrons are the primary actors in this process.
One common way atoms achieve a full valence shell is by sharing valence electrons with other atoms, forming covalent bonds. This sharing allows both atoms to effectively "count" the shared electrons towards their valence shell, leading to a more stable configuration. The equal sharing of electrons results in non-polar covalent bonds, while unequal sharing results in polar covalent bonds.
Reactivity and the Number of Valence Electrons
The number of valence electrons an element has directly influences its reactivity. Elements with nearly full or nearly empty valence shells are generally more reactive because they readily gain or lose electrons to achieve a stable configuration.
For example, elements with one or two valence electrons tend to lose these electrons to form positive ions (cations). Conversely, elements with six or seven valence electrons tend to gain electrons to form negative ions (anions).
Elements with a full valence shell, such as the noble gases (helium, neon, argon, etc.), are generally inert or unreactive because they already have a stable electron configuration.
Deciphering the roles of valence electrons sets the stage for understanding how atoms interact. Now, we turn our attention to the specific atoms that combine to form methane: carbon and hydrogen. By examining their individual electronic structures, we can begin to unravel the puzzle of methane's formation.
Methane's Building Blocks: Carbon and Hydrogen Demystified
Methane, the simplest alkane, is composed of just two elements: carbon and hydrogen. Understanding the electronic structure of these elements is fundamental to grasping how they bond together to form this ubiquitous molecule. Let's delve into the atomic structure of each.
Carbon (C): The Tetravalent Foundation
Carbon, with the atomic number 6, occupies a central role in organic chemistry due to its unique ability to form stable bonds with itself and a wide variety of other elements. This versatility stems directly from its electronic configuration.
Electronic Configuration and Valence Electrons
The electronic configuration of carbon is 1s² 2s² 2p². This means that carbon has two electrons in its inner shell (1s²) and four electrons in its outer shell (2s² 2p²).
These four outermost electrons are the valence electrons, which determine carbon's bonding behavior.
The Drive for Four Covalent Bonds
Carbon's four valence electrons mean it needs four more electrons to achieve a stable octet configuration. To fulfill this requirement, carbon readily forms four covalent bonds with other atoms.
This tetravalent nature is the cornerstone of carbon's ability to form complex and diverse organic molecules. It allows carbon to create chains, rings, and three-dimensional structures, making it the backbone of life.
Hydrogen (H): The Simplest Element
Hydrogen, with the atomic number 1, is the simplest and most abundant element in the universe. Its atomic structure is straightforward but crucial for understanding its role in chemical bonding.
Electronic Configuration and Valence Electron
The electronic configuration of hydrogen is 1s¹. This indicates that hydrogen has only one electron, residing in its 1s orbital. This single electron is its valence electron.
The Pursuit of a Stable Duet
Unlike most elements that strive for an octet, hydrogen only needs one more electron to achieve a stable configuration, resembling helium (1s²). This stable configuration is often referred to as a 'duet'.
Consequently, hydrogen readily forms one covalent bond with another atom. This tendency to form a single bond makes it an ideal "terminal" atom in molecules like methane. It caps off bonding sites on other atoms like carbon.
Deciphering the roles of valence electrons sets the stage for understanding how atoms interact. Now, we turn our attention to the specific atoms that combine to form methane: carbon and hydrogen. By examining their individual electronic structures, we can begin to unravel the puzzle of methane's formation.
The Covalent Connection: How Methane's Bonds are Formed
Methane's unique stability and structure arise from the covalent bonds formed between its carbon and hydrogen atoms. These bonds are the glue that holds the molecule together. They determine its properties. Let's examine how these bonds are specifically created in methane.
Understanding Covalent Bonds
A covalent bond is a chemical bond formed through the sharing of electron pairs between atoms. This sharing allows atoms to achieve a more stable electron configuration. Instead of transferring electrons (like in ionic bonds), atoms "pool" their valence electrons.
This mutual sharing satisfies the electronic needs of both participating atoms. The shared electrons effectively become part of the outer shell of both atoms involved in the bond. This allows each to achieve a full or more stable outer electron shell.
Electron Sharing in Methane
In methane (CH₄), one carbon atom forms covalent bonds with four hydrogen atoms. Carbon, with its four valence electrons, requires four more to complete its octet. Hydrogen, with its single valence electron, needs one more to achieve a stable duet (similar to helium).
The beauty of covalent bonding is that it simultaneously addresses the needs of both carbon and hydrogen. Each hydrogen atom shares its single electron with the carbon atom. Carbon, in turn, shares one of its four valence electrons with each hydrogen atom.
This mutual sharing results in each hydrogen atom effectively having two electrons (1s² configuration). Carbon effectively gains eight electrons in its outer shell (octet rule). Thus, fulfilling the stability requirements of both.
The Process of Covalent Bond Formation in Detail
The formation of a covalent bond in methane is a dynamic process. It involves the interaction of atomic orbitals. Initially, the valence electrons of carbon and hydrogen reside in their respective atomic orbitals.
As the atoms approach each other, these atomic orbitals begin to overlap. This overlap creates a new molecular orbital. The shared electrons now occupy this molecular orbital. It is this shared molecular orbital that constitutes the covalent bond.
The shared electrons are not static. They are constantly in motion, orbiting both the carbon and hydrogen nuclei. This movement creates a region of high electron density between the atoms, effectively "gluing" them together.
This sharing of electron density lowers the overall energy of the system. Lower energy states are inherently more stable. Thus, the formation of covalent bonds leads to a stable methane molecule. The stable methane molecule resists dissociation.
The beauty of covalent bonding is that it simultaneously addresses the needs of both carbon and hydrogen. Each hydrogen atom shares its single valence electron with carbon, and carbon, in turn, shares one of its four valence electrons with each hydrogen. This mutual sharing satisfies the electronic needs of both atoms. Now, we'll look at a way to visually represent this sharing, and the bonds it creates.
Visualizing Methane: Lewis Structures and Their Limitations
Lewis structures provide a simple yet valuable method for visualizing the arrangement of atoms and the distribution of electrons within a molecule. While useful for understanding basic bonding patterns, it's crucial to recognize their limitations, especially when dealing with the three-dimensional nature of molecules like methane.
Lewis structures, also known as electron dot diagrams, are diagrams that show the bonding between atoms of a molecule as well as the lone pairs of electrons that may exist in the molecule.
These diagrams use dots to represent valence electrons. Lines represent covalent bonds formed by the sharing of electron pairs. For simple molecules, Lewis structures are invaluable tools for predicting molecular geometry and understanding chemical reactivity.
The Lewis Structure of Methane
In the case of methane (CH₄), the Lewis structure depicts a central carbon atom (C) surrounded by four hydrogen atoms (H). Each hydrogen atom is connected to the carbon atom by a single line. This line represents a single covalent bond.
Each covalent bond consists of two shared electrons, one from the carbon atom and one from the hydrogen atom. The Lewis structure clearly shows that the carbon atom shares four pairs of electrons, achieving a complete octet (eight valence electrons), while each hydrogen atom shares one pair, achieving a stable duet (two valence electrons).
This visual representation reinforces the concept of electron sharing and helps in understanding how methane achieves its stable electronic configuration.
Limitations of Lewis Structures
Despite their usefulness, Lewis structures have some important limitations, especially when it comes to representing the true three-dimensional structure of molecules. Lewis structures are inherently two-dimensional, and they cannot accurately depict the spatial arrangement of atoms in a molecule.
For example, the Lewis structure of methane suggests a square planar geometry. But, in reality, methane has a tetrahedral geometry. This means that the four hydrogen atoms are arranged around the carbon atom in a three-dimensional tetrahedron. The bond angles between the hydrogen atoms are approximately 109.5 degrees.
Bond Angles and Molecular Shape
Lewis structures do not provide any information about bond angles. They cannot be used to predict the shape of a molecule accurately. This is because Lewis structures only show the connectivity of atoms. They do not account for the repulsive forces between electron pairs that influence the molecule's ultimate three-dimensional shape.
A Simplified Model
Therefore, while Lewis structures serve as a good starting point for understanding chemical bonding, they should be complemented with other models and theories, such as Valence Shell Electron Pair Repulsion (VSEPR) theory. This is to gain a more accurate understanding of molecular geometry.
In conclusion, Lewis structures offer a valuable visual aid for understanding electron sharing and covalent bond formation in methane. However, it is crucial to remember their limitations. Particularly, their inability to accurately represent the three-dimensional shape and bond angles of the molecule. Relying solely on Lewis structures can lead to misconceptions about molecular properties.
Each covalent bond consists of two shared electrons, one from the carbon atom and one from each of the hydrogen atoms. Understanding how these bonds contribute to the stability of the methane molecule requires delving into the fundamental principle known as the octet rule.
The Octet Rule in Action: Achieving Stability in Methane
The octet rule is a guiding principle in chemistry. It explains the tendency of atoms to achieve a stable electron configuration similar to that of noble gases. Noble gases possess a full outer shell of eight valence electrons (except for helium, which has two). This full shell configuration is exceptionally stable.
The octet rule dictates that atoms will gain, lose, or share electrons in order to achieve this stable configuration. For many elements, especially those in the second period like carbon, achieving an octet is paramount to forming stable compounds. In methane, the octet rule is elegantly satisfied.
Understanding the Octet Rule
The octet rule is intrinsically linked to the electronic structure of atoms. Atoms are most stable when their outermost electron shell (the valence shell) is full. This full shell typically contains eight electrons. Hence, atoms often react with each other to achieve this stable octet configuration.
Methane and the Octet: A Perfect Match
In the methane molecule (CH₄), carbon forms four covalent bonds with four hydrogen atoms. Each covalent bond represents the sharing of two electrons. Carbon contributes one electron to each bond. Each hydrogen atom contributes the other.
Therefore, carbon effectively gains four electrons through these shared bonds. Since carbon inherently has four valence electrons, sharing four more gives it a total of eight electrons in its valence shell. This satisfies the octet rule, making the carbon atom in methane remarkably stable.
Each hydrogen atom, in turn, achieves a stable electron configuration by sharing one electron with carbon. Hydrogen follows the duet rule, achieving a full valence shell with two electrons, similar to helium. This mutual satisfaction of electron requirements is what makes methane such a stable and prevalent molecule.
Exceptions to the Octet Rule
While the octet rule is a powerful tool, it is essential to recognize that it's not universally applicable. Some molecules and ions violate the octet rule. This is especially common for elements in the third period and beyond.
For instance, elements like phosphorus and sulfur can accommodate more than eight electrons in their valence shells. This phenomenon is known as an expanded octet. Molecules like phosphorus pentachloride (PCl₅) and sulfur hexafluoride (SF₆) are examples of this.
However, methane strictly adheres to the octet rule. The carbon atom achieves a perfect octet through covalent bonding with hydrogen atoms. Understanding this compliance is key to grasping the molecule's stability and reactivity.
The elegance of methane's structure, stemming from the octet rule, naturally raises a question: how do the underlying principles of electron configuration govern this bonding behavior? Diving deeper into the electronic arrangement of carbon and hydrogen provides a crucial lens through which to understand the stability of the methane molecule.
Electron Configuration and Energy Levels: A Deeper Dive
Electron configuration describes the arrangement of electrons within an atom. It is a fundamental concept for understanding chemical bonding.
The Basics of Electron Configuration
Electrons occupy specific energy levels or shells around the nucleus.
Each shell can hold a certain maximum number of electrons.
The first shell (n=1) holds up to two electrons. The second shell (n=2) holds up to eight electrons, and so on.
Within each shell are subshells (s, p, d, f), each with its own characteristic shape and energy level.
Electrons fill these subshells according to specific rules, such as the Aufbau principle and Hund's rule, to achieve the lowest possible energy state.
Electron Configuration of Carbon and Hydrogen
Understanding the electron configurations of carbon and hydrogen is key to understanding their bonding behavior in methane.
Carbon's Electron Configuration
Carbon (C) has an atomic number of 6, meaning it has six electrons.
Its electron configuration is 1s² 2s² 2p².
This indicates two electrons in the 1s subshell, two in the 2s subshell, and two in the 2p subshell.
Carbon has four valence electrons (2s² 2p²) in its outermost shell, which accounts for its ability to form four covalent bonds.
Hydrogen's Electron Configuration
Hydrogen (H) has an atomic number of 1, meaning it has one electron.
Its electron configuration is 1s¹.
Hydrogen has one valence electron in its outermost shell, which means it needs one more electron to achieve a full outer shell (like helium).
This explains its tendency to form one covalent bond.
Energy Levels and Stable Covalent Bonds
The formation of covalent bonds is driven by the principle of achieving a lower, more stable energy state.
When atoms combine to form a molecule, the electrons rearrange themselves to occupy lower energy levels.
For instance, when carbon and hydrogen form covalent bonds in methane, the shared electrons are in a lower energy state than they would be in isolated atoms.
This decrease in energy is what drives the formation of the stable methane molecule.
The shared electrons are more stable because they are attracted to the positive charges of both the carbon and hydrogen nuclei.
The greater the decrease in energy, the stronger and more stable the covalent bond.
Methane's 3D Shape: Tetrahedral Geometry Explained
The story of methane doesn't end with understanding electron sharing. While Lewis structures offer a flat, two-dimensional representation of bonding, molecules like methane exist in three-dimensional space. This spatial arrangement, known as molecular geometry, significantly influences a molecule's properties and behavior.
Understanding Molecular Geometry
Molecular geometry describes the three-dimensional arrangement of atoms within a molecule. It's not just about connectivity, but also about the spatial relationships between atoms and the overall shape of the molecule. This shape dictates how the molecule interacts with other molecules, affecting its physical properties (like boiling point and melting point) and its chemical reactivity.
For instance, a molecule's shape can determine whether it can fit into a specific receptor site in the body, influencing its biological activity. Therefore, understanding molecular geometry is crucial for predicting and explaining a wide range of chemical phenomena.
The Tetrahedral Geometry of Methane
Methane (CH₄) adopts a tetrahedral geometry. This means that the carbon atom sits at the center of a tetrahedron, with each of the four hydrogen atoms positioned at the corners.
Imagine a pyramid with a triangular base; that's essentially a tetrahedron. What's particularly noteworthy is the bond angle – the angle between any two hydrogen atoms connected to the central carbon. In methane, this bond angle is approximately 109.5 degrees. This specific angle is key to understanding the stability and properties of methane.
Minimizing Repulsion: VSEPR Theory
Why does methane adopt this specific tetrahedral shape? The answer lies in minimizing repulsion between electron pairs.
The Valence Shell Electron Pair Repulsion (VSEPR) theory provides a simple but powerful explanation. VSEPR theory posits that electron pairs, whether they are bonding pairs (involved in covalent bonds) or lone pairs (non-bonding electrons), repel each other.
These electron pairs arrange themselves around the central atom in a way that maximizes the distance between them, thereby minimizing repulsion and achieving the lowest possible energy state.
In methane, there are four bonding pairs of electrons around the central carbon atom. To maximize the distance between these four pairs, they arrange themselves in a tetrahedral geometry. This arrangement allows each bonding pair to be as far away from the others as possible, resulting in the observed 109.5-degree bond angles and the overall stable structure of methane.
Methane's tetrahedral geometry arises from the need to minimize electron repulsion. But what does that shape, and those covalent bonds, actually do for methane?
Chemical Bonding and Methane's Unique Properties
The properties of any molecule are a direct consequence of its structure and the types of chemical bonds it possesses. In the case of methane (CH₄), its unique characteristics are deeply intertwined with its four covalent bonds.
Covalent Bonds and Methane's Intrinsic Stability
Covalent bonds are formed through the sharing of electrons between atoms. This sharing creates a stable electron configuration for both the carbon and hydrogen atoms in methane.
Carbon achieves an octet (eight valence electrons), while each hydrogen atom achieves a duet (two valence electrons), resembling the stable electron configurations of noble gases.
This shared electron arrangement results in a strong attractive force holding the atoms together, contributing significantly to methane's overall stability.
The stability afforded by covalent bonding means methane requires a considerable amount of energy to break apart its constituent atoms.
Bonding's Influence on Physical Properties
The type of bonding dramatically affects a substance's physical properties. Methane, held together by covalent bonds, exhibits a low boiling point (-161.5 °C) and a low melting point (-182.5 °C).
This is because the intermolecular forces between methane molecules are relatively weak. These forces, known as Van der Waals forces or London Dispersion forces, are temporary, induced dipoles.
Unlike ionic compounds with strong electrostatic interactions, methane's molecules aren't as strongly attracted to each other. Therefore, less energy is required to overcome these forces and transition methane from a liquid to a gas, or a solid to a liquid.
Methane's Non-Polar Nature and Its Impact
While the carbon-hydrogen bonds in methane are slightly polar due to a small difference in electronegativity, the tetrahedral geometry of the molecule cancels out these individual bond dipoles.
This results in an overall non-polar molecule. The symmetrical arrangement ensures that the electron density is evenly distributed, leading to a negligible net dipole moment.
Consequences of Non-Polarity
The non-polar nature of methane has significant consequences.
First, it makes methane insoluble in water, a polar solvent ("like dissolves like" principle). It cannot form favorable interactions with water molecules.
Second, it influences methane's behavior in various chemical reactions. It tends to interact more readily with other non-polar substances.
Understanding the non-polar nature of methane is crucial in various applications, including predicting its behavior in environmental contexts and designing chemical processes.
Video: Mastering Methane: Valence Electrons Explained in Detail
Frequently Asked Questions: Mastering Methane Valence Electrons
These FAQs address common questions about methane and its valence electrons.
What are valence electrons, and why are they important for methane?
Valence electrons are the electrons in the outermost shell of an atom. They're crucial because they determine how an atom interacts with other atoms to form chemical bonds. For methane (CH4), understanding the valence electrons of carbon and hydrogen explains how these elements bond together.
How many valence electrons does carbon have in methane?
Carbon has four valence electrons. These four valence electrons allow it to form four covalent bonds with four hydrogen atoms, resulting in the stable methane molecule.
How many valence electrons does hydrogen have in methane?
Hydrogen has one valence electron. Each hydrogen atom shares its single valence electron with carbon to form a covalent bond in methane.
What is the resulting structure of methane based on its valence electrons?
The methane valence electrons arrangement results in a tetrahedral structure. Carbon sits in the center, and the four hydrogen atoms are positioned symmetrically around it. This specific arrangement minimizes repulsion between the electron pairs, leading to a stable and non-polar molecule.