Unlock the Secrets: Methane Phase Diagram Explained!
Understanding methane's behavior under varying conditions necessitates exploration of its phase diagram. A crucial concept in chemical engineering, the methane phase diagram visually represents the thermodynamic stability of methane in different phases. Thermodynamics governs these phase transitions, dictating the conditions under which methane exists as a solid, liquid, or gas. The United States Geological Survey (USGS) often utilizes methane phase diagrams in assessing natural gas reserves and predicting methane hydrate stability. Moreover, software like CoolProp facilitates precise calculations related to methane's thermodynamic properties, including its phase transitions as depicted on the methane phase diagram.
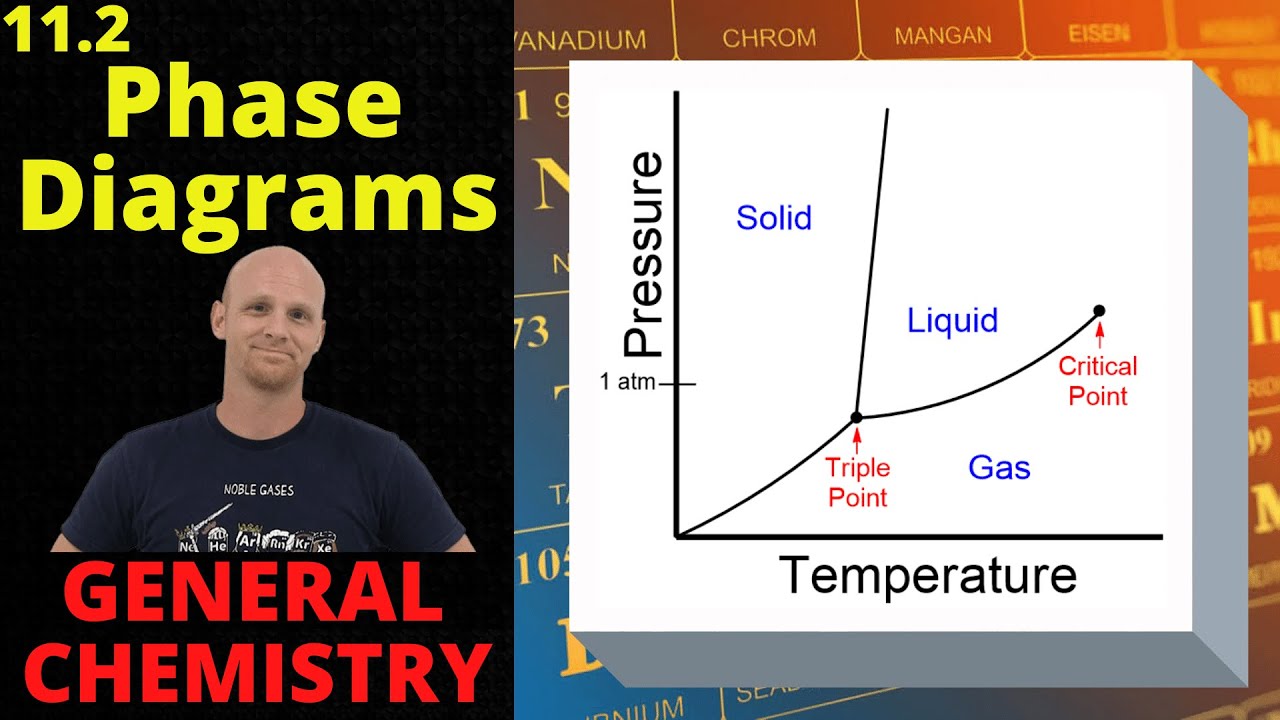
Image taken from the YouTube channel Chad's Prep , from the video titled 11.2 Phase Diagrams | General Chemistry .
Methane, a molecule composed of one carbon and four hydrogen atoms (CH4), is far more than just a simple chemical compound. Its role is pivotal and multifaceted, extending across the realms of energy production, environmental science, and various industrial processes.
As the primary component of natural gas, it serves as a crucial fuel source, powering homes and industries worldwide. Methane's influence, however, extends beyond its energy-generating capabilities.
Its potency as a greenhouse gas also makes it a significant player in climate change, necessitating a comprehensive understanding of its behavior in different environments. Further, it serves as a feedstock for manufacturing of a vast range of chemicals.
The Significance of Understanding Methane's Behavior
Methane's properties and behavior are highly sensitive to changes in temperature and pressure. Understanding how methane behaves under different conditions is paramount for numerous reasons.
In the energy sector, predicting methane's phase (solid, liquid, or gas) is critical for optimizing extraction, transportation, and storage processes. The economics of natural gas rely heavily on this.
Environmentally, comprehending the conditions under which methane hydrates form and decompose is crucial for assessing the risks associated with methane release from thawing permafrost and marine sediments.
This is of great importance when trying to determine environmental impact.
Industrially, controlling methane's phase allows for the development of innovative technologies, such as methane-based fuel cells and advanced materials. Therefore, a strong understanding of this is needed for innovation.
Phase Diagrams: A Roadmap to Methane's Many Forms
To accurately predict and control methane's behavior, scientists and engineers rely on a powerful tool: the phase diagram.
A phase diagram is a graphical representation that illustrates the thermodynamically stable phases of a substance under different conditions of temperature and pressure.
For methane, the phase diagram reveals the specific conditions under which it exists as a solid, liquid, or gas, as well as the boundaries between these phases. It is therefore extremely important for determining appropriate action.
By consulting the methane phase diagram, we can determine whether methane will exist as a gas, a liquid, or a solid under specific conditions. This knowledge is critical for a multitude of applications.
Article Goal: A Comprehensive Exploration
This article aims to provide a clear and comprehensive explanation of the methane phase diagram. We will delve into the key features of the diagram, including the triple point, the critical point, and the phase boundaries.
By understanding these concepts, readers will gain the ability to interpret the methane phase diagram effectively and apply this knowledge to real-world scenarios.
Phase Diagrams: A Quick Guide
Understanding methane's behavior in different conditions necessitates a grasp of phase diagrams. These graphical representations are the roadmaps that delineate the stable states of a substance under varying conditions of temperature and pressure. Before diving into the specifics of methane's phase diagram, it is essential to establish a firm understanding of what phase diagrams are, how to read them, and the significance of their key features.
What is a Phase Diagram?
A phase diagram is a graphical representation illustrating the physical states (phases) of a substance under different conditions of temperature and pressure.
It serves as a powerful tool in materials science, chemistry, and engineering, providing critical information for predicting and controlling the behavior of substances in various applications.
Phase diagrams are not substance-specific. Each pure substance will have a unique phase diagram to represent its specific characteristics.
Deciphering the Axes: Pressure and Temperature
The foundation of any phase diagram lies in its axes.
Typically, the x-axis represents temperature, increasing from left to right.
The y-axis represents pressure, increasing from bottom to top.
These axes define the environmental conditions under which a substance exists in a particular phase. By pinpointing a specific temperature and pressure on the diagram, we can determine whether the substance will be a solid, liquid, or gas.
The Three Primary Phases: Solid, Liquid, and Gas
Within the boundaries defined by the pressure and temperature axes, a phase diagram delineates the regions corresponding to the three primary phases of matter: solid, liquid, and gas.
The solid phase typically occupies the lower-left region of the diagram, indicative of lower temperatures and higher pressures favoring the formation of a rigid structure.
As temperature increases and/or pressure decreases, the substance may transition to the liquid phase.
At even higher temperatures and/or lower pressures, the substance exists as a gas.
Phase Transition Lines: Boundaries of Change
The lines or curves on a phase diagram are not merely decorative; they are crucial indicators of phase transitions.
Each line represents the set of temperature and pressure conditions under which two phases can coexist in equilibrium.
For example, the line separating the solid and liquid phases represents the melting point at different pressures. Similarly, the line between the liquid and gas phases represents the boiling point at different pressures.
These lines are vital for predicting the conditions required to induce a phase change.
Critical Points and Triple Points: Defining Substance Behavior
Two particularly significant points on a phase diagram are the triple point and the critical point.
The triple point is the unique set of temperature and pressure conditions at which all three phases – solid, liquid, and gas – can coexist in equilibrium. This point is invariant for a pure substance and serves as a fixed reference point.
The critical point, on the other hand, represents the temperature and pressure beyond which a distinct liquid phase does not exist. Above the critical temperature and pressure, the substance exists as a supercritical fluid, exhibiting properties of both a liquid and a gas.
Understanding the triple and critical points provides invaluable insights into the fundamental behavior of a substance and its limitations. They are essential for predicting the state of matter under extreme conditions.
The Methane Phase Diagram: A Deep Dive
Having established a fundamental understanding of phase diagrams and their components, we can now turn our attention to the specific case of methane. The methane phase diagram is our key to unlocking the secrets of this molecule's behavior under varying pressures and temperatures.
It provides a visual roadmap for predicting whether methane will exist as a solid, liquid, or gas under given conditions, which is crucial for applications ranging from natural gas processing to understanding the dynamics of methane hydrates in permafrost.
The following sections will dissect the methane phase diagram, highlighting its key features, defining data points, and thoroughly explaining the phase boundaries. This knowledge empowers one to interpret the diagram effectively and apply it to real-world scenarios.
Unveiling the Methane Phase Diagram
Below, we present the Methane Phase Diagram. Note that visual representations are crucial for understanding. The graph plots pressure (typically in Pascals or atmospheres) against temperature (typically in Kelvin or Celsius).
[Include Visual Representation of the Methane Phase Diagram here]
The diagram clearly displays the regions corresponding to solid, liquid, and gaseous methane, separated by distinct curves representing phase transitions.
Key Features and Data Points
Certain points on the phase diagram are especially significant, providing valuable insights into methane's properties. These are the triple point and the critical point. They represent unique conditions where methane exhibits particular behaviors.
The Triple Point of Methane
The triple point is the unique temperature and pressure at which all three phases – solid, liquid, and gas – coexist in equilibrium. For methane, the triple point occurs at:
- Temperature: 90.68 K (-182.47 °C)
- Pressure: 0.0117 MPa (0.115 atm)
At this specific condition, one could theoretically observe solid, liquid, and gaseous methane simultaneously. This point is fundamental for calibrating scientific instruments and defining thermodynamic scales.
The Critical Point of Methane
The critical point represents the temperature and pressure beyond which distinct liquid and gas phases do not exist. Above this point, methane exists as a supercritical fluid, exhibiting properties of both a liquid and a gas. For methane, the critical point occurs at:
- Temperature: 190.6 K (-82.55 °C)
- Pressure: 4.599 MPa (45.4 atm)
Understanding the critical point is vital for optimizing processes like natural gas liquefaction. Supercritical methane possesses unique solvent capabilities and transport properties that are exploited in various industrial applications.
Phase Boundaries Explained
The lines or curves on the phase diagram delineate the boundaries between different phases. Each line represents the set of temperature and pressure conditions under which two phases can coexist in equilibrium. Understanding these boundaries is crucial for predicting phase transitions.
The Sublimation Curve
The sublimation curve represents the transition between solid methane and gaseous methane (sublimation).
This curve is located at the lower-left portion of the phase diagram, representing relatively low temperatures and pressures.
The sublimation curve indicates the specific pressure at which solid methane will directly transform into gas at a given temperature, skipping the liquid phase entirely.
This is particularly relevant in environments like outer space, where low temperatures and pressures prevail.
The Melting Curve
The melting curve represents the transition between solid methane and liquid methane (melting or fusion). It slopes upwards and to the right, indicating that higher pressures generally require higher temperatures for melting to occur.
The slope of the melting curve is positive for most substances, including methane, although there are exceptions like water.
Understanding the melting curve is essential for processes involving the handling and storage of cryogenic methane.
The Vaporization Curve
The vaporization curve represents the transition between liquid methane and gaseous methane (vaporization or boiling).
This curve terminates at the critical point.
The vaporization curve is crucial for understanding the behavior of methane in natural gas processing, transportation, and storage.
The pressure at a given temperature on this curve represents the vapor pressure of methane at that temperature. It dictates how readily liquid methane will vaporize into gas.
Methane Phase Transitions and Equations of State
Having thoroughly examined the landscape of the methane phase diagram, pinpointing its key features and notable data points, it's time to delve deeper into the mathematical frameworks that govern methane's phase transitions. This section explores how equations of state, such as the Van der Waals equation and the Clapeyron equation, model the intricate relationships between pressure, temperature, and volume during these critical transformations.
Understanding Methane Phase Transitions
Methane, like any substance, undergoes distinct phase transitions depending on temperature and pressure.
-
Sublimation: Direct transition from solid to gas.
-
Melting (Fusion): Solid to liquid transition.
-
Vaporization: Liquid to gas transition.
Each transition occurs along a specific curve on the phase diagram, representing the equilibrium conditions where two phases coexist. These transitions are not instantaneous; they involve energy input (or release) as the methane molecules overcome intermolecular forces.
The energy required for melting is the latent heat of fusion, while the energy for vaporization is the latent heat of vaporization.
The Van der Waals Equation of State
The Van der Waals equation is a modification of the ideal gas law that accounts for the non-ideal behavior of real gases like methane.
It introduces two correction terms: one for the finite size of the molecules and another for the attractive forces between them.
The equation is expressed as:
(P + a(n/V)^2) (V - nb) = nRT
Where:
- P is pressure.
- V is volume.
- n is the number of moles.
- T is temperature.
- R is the ideal gas constant.
- a accounts for intermolecular forces.
- b accounts for the volume occupied by the molecules.
The a and b parameters are specific to each gas and are determined experimentally.
The Van der Waals equation provides a more accurate representation of methane's behavior than the ideal gas law, particularly at higher pressures and lower temperatures where intermolecular forces become significant.
The Clapeyron Equation and Phase Equilibrium
The Clapeyron equation is a powerful tool for determining the slope of the phase boundaries on the phase diagram.
It relates the change in pressure with temperature to the enthalpy and volume changes associated with a phase transition.
The Clapeyron equation is given by:
dP/dT = ΔH / (TΔV)
Where:
- dP/dT is the slope of the phase boundary.
- ΔH is the enthalpy change (latent heat) of the transition.
- T is the temperature.
- ΔV is the change in volume during the transition.
This equation is crucial for predicting how the equilibrium temperature between two phases changes with pressure. For instance, it can be used to determine how the melting point of methane changes as pressure increases. It directly links thermodynamic properties (enthalpy and volume) to the phase behavior observed on the phase diagram.
Limitations of Equations of State
While the Van der Waals and Clapeyron equations provide valuable insights into methane's phase behavior, they have limitations, especially under extreme conditions.
The Van der Waals equation is a cubic equation of state, which can lead to inaccuracies near the critical point.
More sophisticated equations of state, such as the Peng-Robinson equation or more complex models based on molecular simulation, are often required for accurate predictions at very high pressures or low temperatures.
Furthermore, these equations assume a homogeneous system and may not accurately represent methane behavior in complex mixtures or in the presence of other substances.
Therefore, it’s crucial to recognize the limitations of any equation of state and validate predictions with experimental data whenever possible. The complexity of intermolecular interactions and quantum effects at extreme conditions makes accurate modeling a significant challenge.
Having explored the equations governing methane's behavior, it’s natural to wonder where this knowledge finds practical application. The theoretical understanding of methane's phases translates directly into crucial processes and environmental considerations, impacting industries and our planet's climate.
Real-World Applications of the Methane Phase Diagram
The methane phase diagram isn't just an academic exercise; it's a vital tool shaping how we handle and understand this ubiquitous molecule in the real world. Its principles are indispensable in optimizing natural gas processing and in comprehending the behavior of methane hydrates, which hold significant implications for environmental science.
Natural Gas Processing: Liquefaction and Optimization
Natural gas, primarily composed of methane, is a critical energy source globally. However, its gaseous state poses challenges for long-distance transportation. This is where understanding the methane phase diagram becomes essential.
The Role of Liquefaction
Liquefaction, the process of converting natural gas into its liquid form (LNG), drastically reduces its volume, enabling efficient shipping and storage. The methane phase diagram dictates the precise temperature and pressure conditions required to achieve this phase change.
Cooling methane below its critical temperature (-82.6 °C) and applying appropriate pressure forces it to condense into a liquid. Operating efficiently in this zone of the phase diagram minimizes energy consumption and maximizes the volume reduction.
Without precise control informed by the phase diagram, liquefaction becomes inefficient, costly, or even impossible. This underscores the diagram's fundamental role in the LNG supply chain.
Optimization Based on Phase Behavior
Beyond simple liquefaction, the phase diagram enables optimization of the entire natural gas processing workflow. From extraction to transportation, maintaining conditions within specific regions of the phase diagram ensures the gas remains in the desired state, preventing unwanted phase transitions that could damage equipment or reduce efficiency.
For instance, pipelines are often operated at pressures and temperatures that keep methane in a supercritical fluid state. This dense phase offers a balance between liquid-like density and gas-like flow properties, minimizing pumping costs and maximizing throughput. The diagram allows operators to identify and maintain these optimal conditions.
Environmental Science: Methane Hydrates and Climate Change
Methane hydrates, also known as methane clathrates, are ice-like solids where methane molecules are trapped within a crystal structure of water. These formations are abundant in permafrost regions and on the ocean floor. Their stability is heavily dependent on temperature and pressure, as defined by the methane phase diagram.
Formation Conditions and the Phase Diagram
Methane hydrates form under specific conditions of low temperature and high pressure. The phase diagram illustrates the precise range of these conditions, showing where the hydrate phase is stable.
As temperatures rise or pressure decreases, these hydrates can dissociate, releasing trapped methane into the atmosphere. The phase diagram provides a framework for predicting and understanding this dissociation process.
Environmental Implications of Methane Release
Methane is a potent greenhouse gas, with a global warming potential significantly higher than carbon dioxide over a shorter timeframe. The release of methane from thawing hydrates represents a significant threat to the climate.
Understanding the phase diagram allows scientists to model and predict the rate and extent of methane release from hydrate deposits under various climate change scenarios. This information is crucial for developing strategies to mitigate this risk.
Further research and monitoring are necessary to better understand the complex interplay between climate change, hydrate stability, and methane release, and the phase diagram serves as a critical tool in this ongoing investigation.
Tools and Resources for Further Exploration
The journey to understanding the methane phase diagram doesn't end with a single explanation. For those eager to delve deeper and apply this knowledge, a wealth of resources is available. These range from comprehensive thermodynamic databases to specialized software tools, all designed to enhance understanding and facilitate practical application.
NIST Chemistry WebBook: A Thermodynamic Data Goldmine
The National Institute of Standards and Technology (NIST) Chemistry WebBook stands as a cornerstone for accurate thermodynamic data. It's an invaluable resource for anyone working with methane or other chemical substances.
Within this online compendium, you'll find meticulously compiled data on methane's:
- Phase behavior.
- Thermodynamic properties (enthalpy, entropy, heat capacity).
- Transport properties (viscosity, thermal conductivity).
This information is critical for:
- Validating calculations.
- Designing processes.
- Making informed decisions across various applications.
The WebBook's user-friendly interface allows for easy searching and retrieval of data. This makes it accessible to both seasoned researchers and those new to the field. It is often considered the go-to reference for reliable thermochemical information.
Software and Databases for Phase Diagram Calculations
Manually constructing and interpreting phase diagrams can be time-consuming. Luckily, several software packages and databases are designed to streamline this process. These tools leverage advanced algorithms and extensive data sets to:
- Calculate phase equilibria.
- Simulate material behavior under different conditions.
- Generate phase diagrams for complex systems.
Popular options include:
- Thermo-Calc: A powerful software package widely used in academia and industry for thermodynamic calculations and phase diagram prediction.
- Pandat: Another leading software for multi-component phase diagram calculation, offering a user-friendly interface and extensive database support.
- Materials Project: An open-source database that provides calculated materials properties and phase diagrams, promoting collaborative research and data sharing.
These tools allow for detailed exploration of methane's phase behavior under a wide range of conditions. They facilitate tasks such as:
- Predicting the formation of methane hydrates.
- Optimizing gas separation processes.
- Designing high-pressure experiments.
They offer significant advantages in terms of efficiency and accuracy, enabling researchers and engineers to tackle complex challenges with greater confidence.
Navigating Research Articles and Publications
Staying abreast of the latest research is crucial for a comprehensive understanding of methane phase behavior. Scientific journals and publications offer a wealth of information on cutting-edge developments, novel experimental techniques, and advanced modeling approaches.
Search engines like:
- Google Scholar.
- Web of Science.
- Scopus.
These are excellent starting points for discovering relevant research articles.
Keywords such as:
- "Methane phase diagram".
- "Methane hydrates".
- "Equation of state methane".
These will help you refine your search and uncover specific topics of interest.
Additionally, professional organizations such as:
- The American Institute of Chemical Engineers (AIChE).
- The American Physical Society (APS).
They often host conferences and publish journals featuring the latest research on thermodynamics and phase behavior. Engaging with this literature provides valuable insights into the ongoing efforts to better understand and utilize methane.
Video: Unlock the Secrets: Methane Phase Diagram Explained!
FAQs: Understanding the Methane Phase Diagram
Here are some frequently asked questions about the methane phase diagram to help clarify its key aspects.
What does the methane phase diagram show?
The methane phase diagram illustrates the different physical states (solid, liquid, gas) of methane under varying temperatures and pressures. It maps out the conditions under which methane will exist in each phase.
What are the key points on the methane phase diagram?
Key points include the triple point (where all three phases coexist) and the critical point (beyond which distinct liquid and gas phases no longer exist). These points define the boundaries of the different phases on the methane phase diagram.
Why is the methane phase diagram important?
Understanding the methane phase diagram is crucial in various applications, including natural gas processing, cryogenics, and understanding geological formations where methane is present. It predicts methane behavior under different conditions.
How does pressure affect the phase of methane?
Increasing the pressure on methane can cause it to transition from a gas to a liquid and eventually to a solid, even without changing the temperature. The methane phase diagram visually represents these pressure-temperature relationships.