Meiosis Diagram: High School Biology Guide
Understanding cell division is a cornerstone of high school biology, especially when exploring the complexities of sexual reproduction. Meiosis, a type of cell division essential for genetic diversity, can be visually represented through a meiosis diagram, which clarifies each stage. Khan Academy offers resources that complement textbook explanations, providing students with valuable support as they study intricate biological processes. Visual aids, such as diagrams from educational websites, are invaluable tools for students learning about the phases of meiosis, specifically meiosis I and meiosis II. Teachers often use diagrams to help students grasp how chromosomes behave during meiosis and to improve their exam scores.
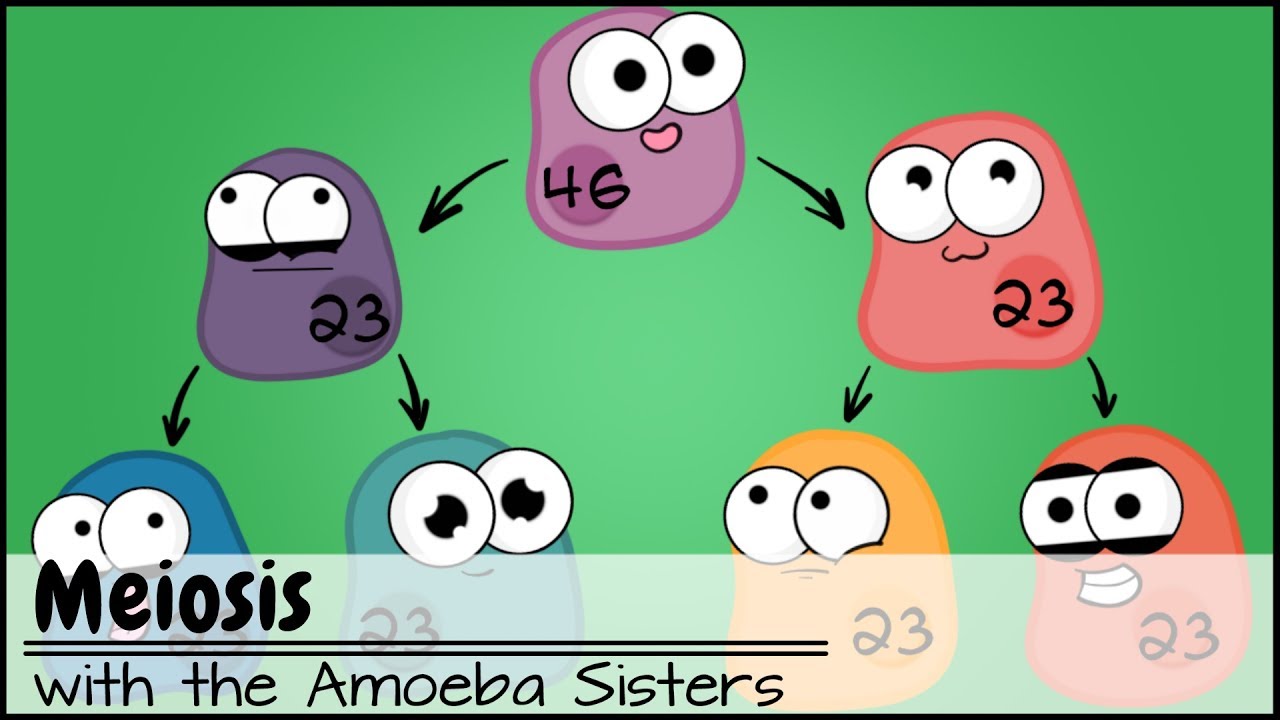
Image taken from the YouTube channel Amoeba Sisters , from the video titled Meiosis (Updated) .
Unveiling the Secrets of Meiosis: The Foundation of Sexual Reproduction
Meiosis, a fundamental process in biology, holds the key to understanding sexual reproduction and the incredible diversity of life. This cellular dance, unlike any other, ensures the continuation of species while simultaneously shuffling the genetic deck. This introduction illuminates why meiosis matters, exploring its unique mechanisms and contrasting it with its cellular cousin, mitosis.
Why Meiosis Matters: The Engine of Genetic Diversity
At its core, meiosis is essential for sexual reproduction. It's the process by which germ cells – sperm in males and eggs in females – are created. Without meiosis, the fusion of two parental cells would lead to a doubling of the chromosome number in each successive generation, a recipe for genetic chaos.
But meiosis does more than just prevent chromosome overload. It's also a primary engine of genetic diversity. By generating unique combinations of genes, meiosis fuels evolution and adaptation, allowing populations to thrive in changing environments. This is an indispensable component of the complex tapestry of life.
Reduction Division: Halving the Chromosome Count
Meiosis is often described as a "reduction division." This refers to its unique ability to halve the chromosome number. Diploid cells (2n), containing two sets of chromosomes (one from each parent), undergo meiosis to produce haploid cells (n), which possess only one set.
This reduction is critical. When a sperm and egg fuse during fertilization, the diploid number is restored, creating a new individual with a unique blend of genetic material from both parents. The magic of sexual reproduction lies in this precise chromosomal choreography.
Genetic Variation: The Meiotic Shuffle
The true power of meiosis lies in its ability to generate genetic variation. This is achieved through two key mechanisms: crossing over and independent assortment. Crossing over involves the exchange of genetic material between homologous chromosomes, creating new combinations of genes.
Independent assortment refers to the random alignment and separation of chromosomes during meiosis, ensuring that each gamete receives a unique mix of maternal and paternal chromosomes. These processes lead to offspring that are genetically distinct from their parents and from each other.
Meiosis vs. Mitosis: A Tale of Two Divisions
While both meiosis and mitosis are forms of cell division, they serve fundamentally different purposes. Mitosis is responsible for growth, repair, and asexual reproduction. It produces two identical daughter cells, each with the same chromosome number as the parent cell.
In contrast, meiosis is solely dedicated to sexual reproduction. It generates four genetically distinct daughter cells, each with half the chromosome number of the parent cell. While mitosis preserves genetic consistency, meiosis embraces genetic diversity. The subsequent sections will fully explore the intricacies of meiosis, stage by stage.
Preparing for Meiosis: Setting the Cellular Stage
Before the intricate choreography of meiosis can commence, the cellular stage must be meticulously prepared. This preparatory phase lays the groundwork for the accurate segregation of chromosomes and the creation of genetically diverse gametes. Let's examine the critical components necessary for this remarkable cellular event to unfold.
Diploid vs. Haploid: Understanding Chromosome Number
One of the most fundamental concepts to grasp before diving into the mechanics of meiosis is the distinction between diploid and haploid cells. Diploid cells, denoted as 2n, contain two complete sets of chromosomes, one inherited from each parent.
In contrast, haploid cells, denoted as n, possess only one set of chromosomes. Meiosis is the process that transforms diploid cells into haploid gametes (sperm and egg cells). This reduction in chromosome number is essential.
This ensures that when fertilization occurs – the fusion of sperm and egg – the resulting zygote will have the correct diploid number of chromosomes. Without this halving, each subsequent generation would have double the number of chromosomes.
Chromosomes: The Carriers of Heredity
At the heart of meiosis lie the chromosomes. These thread-like structures are composed of DNA tightly wound around proteins called histones. They carry the genetic information that determines an organism's traits.
Think of chromosomes as the instruction manuals for building and operating a cell, and ultimately, an entire organism. Each chromosome contains numerous genes, the specific sequences of DNA that code for particular proteins.
Prior to meiosis, each chromosome undergoes replication, resulting in two identical sister chromatids joined together at a region called the centromere. This replication ensures that each daughter cell resulting from meiosis receives a complete copy of the genetic information.
Understanding chromosome structure and behavior is crucial for comprehending the intricacies of meiosis.
The Nucleus: The Meiotic Arena
The entire process of meiosis takes place within the nucleus, the control center of the cell. This membrane-bound organelle houses the chromosomes and provides the necessary environment for the complex molecular events of meiosis to occur.
Within the nucleus, specialized proteins orchestrate the replication, pairing, and separation of chromosomes. The nuclear envelope, the membrane surrounding the nucleus, breaks down during certain stages of meiosis to allow for the movement of chromosomes.
The nucleus truly serves as the meiotic arena, the stage upon which this essential drama unfolds, ensuring the accurate transmission of genetic information from one generation to the next.
Meiosis I: The First Division - Separating Homologous Chromosomes
Before the actual division can happen, DNA synthesis must occur in the S phase of interphase, which results in each chromosome being composed of two identical sister chromatids. The cell also grows in preparation for the division process, so all the proteins required for meiosis are made in interphase as well.
With the cellular stage primed, Meiosis I takes center stage. This initial division is a profound event, distinct from mitosis. It orchestrates the separation of homologous chromosomes, setting the ploidy reduction and genetic recombination that define meiosis. Let’s dissect each phase of this pivotal process.
Prophase I: The Longest Phase and the Beginning of Genetic Diversity
Prophase I stands out as the most extended and complex phase of meiosis. It is within this phase that the magic of genetic recombination truly begins. Chromosomes condense and become visible, but more importantly, homologous chromosomes pair up in a process called synapsis.
Synapsis: The Intimate Pairing of Homologous Chromosomes
Synapsis is a pivotal step in Prophase I. Homologous chromosomes, each bearing genes for the same traits, come together in a precise alignment. This intimate pairing allows for the next crucial event, crossing over, which is central to increasing genetic diversity.
Tetrad Formation: A Visual Manifestation of Synapsis
As homologous chromosomes synapse, they form a structure known as a tetrad, also referred to as a bivalent. Envision four chromatids – two from each homologous chromosome – intertwined. This physical arrangement allows for the exchange of genetic information between non-sister chromatids.
Crossing Over (Recombination): Shuffling the Genetic Deck
Crossing over, also known as genetic recombination, is the hallmark event of Prophase I. It's a process where non-sister chromatids exchange genetic material. This exchange occurs at specific points called chiasmata. Imagine two strands of DNA, intertwined, breaking, and then rejoining with each other, swapping segments in the process. This seemingly small act has profound implications for the genetic makeup of the resulting gametes.
This shuffling of genetic information is a cornerstone of genetic diversity. It ensures that each gamete receives a unique combination of genes from both parents. Without crossing over, the genetic variation passed on to offspring would be significantly limited.
Metaphase I: Aligning for Separation
Following the intricate events of Prophase I, the cell transitions into Metaphase I. In this phase, the tetrads migrate toward the middle of the cell. They align along the metaphase plate, ready for the separation of homologous chromosomes.
Independent Assortment: Randomly Arranging Chromosomes
One of the most important aspects of Metaphase I is the random orientation of homologous chromosome pairs along the metaphase plate. This phenomenon is known as independent assortment. Imagine each tetrad as a pair of magnets. The way these magnets align is completely random. Each arrangement results in a different combination of maternal and paternal chromosomes being inherited.
Independent assortment significantly increases the potential for genetic diversity. It ensures that the resulting gametes will possess a diverse assortment of genetic material.
Spindle Fibers (Microtubules): The Cellular Cables
The alignment and subsequent separation of chromosomes relies on the precise action of spindle fibers, which are constructed from microtubules. These fibers extend from the centrosomes to the kinetochores. These are protein structures located at the centromeres of the chromosomes. The spindle fibers attach to the kinetochores, guiding the chromosomes into alignment and preparing them for separation.
Anaphase I: Pulling Apart
Anaphase I marks the separation of homologous chromosomes. The spindle fibers shorten, pulling the homologous chromosomes toward opposite poles of the cell. It’s important to note that the sister chromatids remain attached at their centromeres, unlike in mitosis. The key here is that it is the homologous pairs that separate, not the sister chromatids.
Telophase I and Cytokinesis: Division Begins
Telophase I marks the arrival of the homologous chromosomes at opposite poles of the cell. The chromosomes may decondense to some extent, and a nuclear envelope reforms around each set of chromosomes.
The cell then undergoes cytokinesis, physically dividing into two daughter cells. Each daughter cell now contains a haploid (n) set of chromosomes, meaning they have half the number of chromosomes as the original diploid cell. Though each chromosome still consists of two sister chromatids, the ploidy has been reduced, setting the stage for Meiosis II.
Before the actual division can happen, DNA synthesis must occur in the S phase of interphase, which results in each chromosome being composed of two identical sister chromatids. The cell also grows in preparation for the division process, so all the proteins required for meiosis are...
Meiosis II: The Second Division - Separating Sister Chromatids
Meiosis II represents the final stage in the meiotic process, closely mirroring the events of mitosis. However, it’s vital to remember that Meiosis II starts with haploid cells, each containing a single set of chromosomes, unlike the diploid cells that undergo mitosis. The primary goal of Meiosis II is the separation of sister chromatids, resulting in the creation of four genetically distinct haploid cells, the gametes necessary for sexual reproduction.
Prophase II: Preparing for the Second Division
Prophase II marks the start of the second meiotic division. If the chromosomes have decondensed after Telophase I, they will now condense again. This phase is relatively brief, as the cell doesn't need to undergo the same extensive preparation seen in Prophase I.
The nuclear envelope, if reformed, breaks down again.
Spindle fibers begin to form in preparation for the separation of sister chromatids. The centrosomes migrate towards opposite poles of the cell, setting the stage for Metaphase II.
Metaphase II: Aligning on the Metaphase Plate
In Metaphase II, the sister chromatids — still attached at the centromere — align along the metaphase plate. This alignment is crucial for ensuring that each daughter cell receives a complete set of genetic information.
The spindle fibers, originating from opposite poles, attach to the kinetochores of each sister chromatid.
This connection ensures that when the time comes, each chromatid will be pulled towards opposite ends of the cell. The organization at the metaphase plate is critical for the accurate segregation of genetic material.
Anaphase II: Separating Sister Chromatids
Anaphase II is characterized by the separation of the sister chromatids. The centromeres divide, and the sister chromatids — now considered individual chromosomes — are pulled apart by the shortening spindle fibers.
Each chromosome migrates towards opposite poles of the cell.
This separation ensures that each resulting daughter cell will receive a complete, single set of chromosomes. This is a critical step in creating haploid gametes.
Telophase II and Cytokinesis: The Final Division
Telophase II is the culmination of meiosis. The chromosomes arrive at the poles of the cell and begin to decondense.
Nuclear envelopes reform around each set of chromosomes, creating four distinct nuclei. Cytokinesis then occurs, dividing the cytoplasm and physically separating the two cells into four individual haploid cells.
The End Result: Four Haploid Gametes
Each of these four cells now contains a haploid (n) number of chromosomes. In animals, these cells will mature into gametes (sperm or egg cells).
In plants, they may develop into spores that can eventually lead to the production of gametes. The successful completion of Meiosis II provides the genetic diversity necessary for evolution and adaptation, securing the continuation of life.
Before the actual division can happen, DNA synthesis must occur in the S phase of interphase, which results in each chromosome being composed of two identical sister chromatids. The cell also grows in preparation for the division process, so all the proteins required for meiosis are...
The Significance of Meiosis: Why It Matters Biologically
Meiosis is far more than just a cellular process; it's a cornerstone of sexual reproduction and genetic diversity. The profound impact of meiosis on the continuation and evolution of life cannot be overstated. Let's explore why this intricate dance of chromosomes is so fundamentally important.
The Genesis of Gametes: Sperm and Egg Cells
At its core, meiosis is the mechanism by which organisms produce gametes - the sperm and egg cells essential for sexual reproduction. These specialized cells are unique in that they carry only half the genetic information of a normal somatic (body) cell. This halving of the chromosome number is crucial.
Without meiosis, the fusion of two somatic cells would result in offspring with double the number of chromosomes. Meiosis ensures that each gamete receives a haploid (n) set of chromosomes, poised to unite with another haploid gamete during fertilization.
Fertilization: Restoring the Diploid State
Fertilization, the union of a sperm and an egg, is the culmination of the meiotic process. This event restores the diploid (2n) chromosome number. This critical step creates a zygote - the first cell of a new organism, carrying a complete set of chromosomes, half from each parent.
The zygote then embarks on a journey of mitotic divisions, eventually developing into a fully formed organism. The brilliance lies in the fact that meiosis and fertilization work in tandem.
Meiosis halves the chromosome number to create gametes. Fertilization restores it for the next generation.
Genetic Variation: The Engine of Evolution
Perhaps the most significant contribution of meiosis is the generation of genetic variation. This is not a simple copying process; it's a dynamic reshuffling of genetic material, creating a unique combination of traits in each offspring. The source of this genetic diversity is found in two key processes during meiosis I: crossing over and independent assortment.
Crossing Over (Recombination): Shuffling the Deck
During prophase I, homologous chromosomes pair up in a process called synapsis. While paired, they can exchange genetic material in a process known as crossing over or recombination.
Imagine shuffling a deck of cards – crossing over is akin to swapping sections of two chromosomes, creating new combinations of genes that were not present in either parent. This swapping occurs at specific points called chiasmata. These crossovers result in recombinant chromosomes, carrying a mosaic of genetic information.
This recombination dramatically increases the genetic diversity within a population, providing raw material for natural selection to act upon.
Independent Assortment: Random Arrangement
Metaphase I introduces another layer of genetic variation through independent assortment. During this phase, homologous chromosome pairs line up randomly at the metaphase plate.
The orientation of each pair is independent of the others. This means that the maternal and paternal chromosomes are randomly distributed to the daughter cells. With 23 pairs of chromosomes in humans, the number of possible combinations is staggering, estimated to be around 8.4 million!
This independent assortment, combined with crossing over, generates a vast array of genetic possibilities in each gamete.
Before the actual division can happen, DNA synthesis must occur in the S phase of interphase, which results in each chromosome being composed of two identical sister chromatids. The cell also grows in preparation for the division process, so all the proteins required for meiosis are...
Potential Errors in Meiosis: When Things Go Wrong
Meiosis, while a remarkably precise process, is not immune to error. These errors can have significant consequences, leading to genetic imbalances and potential developmental disorders. Understanding how these errors arise is crucial for comprehending the complexities of genetics and human health. Let’s delve into the potential pitfalls of meiosis and the tools we have to detect them.
Nondisjunction: The Root of the Problem
At the heart of many meiotic errors lies nondisjunction. This occurs when homologous chromosomes (in Meiosis I) or sister chromatids (in Meiosis II) fail to separate correctly.
Imagine the chromosomes as perfectly choreographed dancers who miss their cue. Instead of moving to opposite sides of the stage (the cell), they stick together.
This failure results in one daughter cell receiving an extra copy of the chromosome, while the other daughter cell is missing that chromosome entirely.
Aneuploidy: An Imbalance of Chromosomes
Nondisjunction directly leads to aneuploidy, a condition in which cells have an abnormal number of chromosomes. A normal human cell has 46 chromosomes, arranged in 23 pairs.
Aneuploidy can manifest as either a gain or a loss of chromosomes. Trisomy refers to the presence of an extra chromosome (2n+1), while monosomy indicates the absence of one chromosome (2n-1).
These imbalances disrupt the delicate balance of genes required for proper development and function.
Specific Examples of Aneuploidy: Understanding the Impact
Down Syndrome (Trisomy 21)
One of the most well-known examples of aneuploidy is Down syndrome, also known as Trisomy 21. This condition arises when an individual has three copies of chromosome 21 instead of the usual two.
This extra chromosome leads to a range of characteristic physical features, developmental delays, and health challenges.
While the severity can vary, individuals with Down syndrome often experience intellectual disability, heart defects, and other health complications.
Other Aneuploidies
While Trisomy 21 is relatively common, other aneuploidies can occur, though many are not compatible with life.
- Trisomy 18 (Edwards syndrome) and Trisomy 13 (Patau syndrome) are two other examples, but these conditions are often associated with severe health problems and a short lifespan.
Sex chromosome aneuploidies, such as Turner syndrome (monosomy X) and Klinefelter syndrome (XXY), also occur and can lead to a range of developmental and reproductive issues.
Karyotypes: Visualizing Chromosomes to Detect Abnormalities
So, how do we detect these chromosomal abnormalities? The answer lies in a technique called karyotyping.
A karyotype is essentially a photograph of an individual's chromosomes arranged in a standardized format.
Cells are arrested during metaphase, when chromosomes are most condensed and visible. Then, they are stained and arranged according to size and banding patterns.
By examining a karyotype, trained professionals can identify any missing, extra, or structurally abnormal chromosomes.
Karyotyping is a valuable tool for diagnosing genetic disorders, providing crucial information for prenatal screening, family planning, and understanding the genetic basis of certain diseases.
The ability to visualize and analyze chromosomes has revolutionized our understanding of genetics and allowed us to diagnose and manage conditions stemming from errors in meiosis.
Video: Meiosis Diagram: High School Biology Guide
FAQs: Meiosis Diagram
What are the main differences between Meiosis I and Meiosis II?
Meiosis I involves the separation of homologous chromosome pairs, reducing the chromosome number by half. The result is two haploid cells with duplicated chromosomes. Meiosis II then separates the sister chromatids of each chromosome, similar to mitosis. A meiosis diagram illustrates these distinct stages.
How does crossing over contribute to genetic diversity?
During prophase I of meiosis, homologous chromosomes exchange genetic material through a process called crossing over. This creates new combinations of alleles on the chromosomes. The meiosis diagram often highlights this crucial step that leads to unique offspring.
What does the meiosis diagram show about chromosome number?
A meiosis diagram shows the chromosome number being halved. Starting with a diploid cell (2n), meiosis produces four haploid cells (n). This ensures that when gametes fuse during fertilization, the offspring will have the correct diploid number of chromosomes.
What are the key stages labeled on a meiosis diagram?
A typical meiosis diagram will clearly label prophase I, metaphase I, anaphase I, telophase I, prophase II, metaphase II, anaphase II, and telophase II. It's important to understand what's happening to the chromosomes in each of these phases.
So, there you have it! Hopefully, this guide helps you wrap your head around meiosis. Don't sweat it if the meiosis diagram still seems a little daunting – just keep practicing, and you'll be a pro in no time. Good luck with your biology studies!