Respiratory Membrane: Lung Function & Gas Exchange

Hey there! Ever wondered how the air you breathe turns into the energy that powers your life? The secret lies within the lungs, where a super-thin structure called the respiratory membrane enables this critical exchange. The alveolar epithelium, an entity within the lungs, features a single-cell thickness, optimizing gas exchange at this vital interface. Diffusion, the process of molecules moving from areas of high concentration to low concentration, is the fundamental mechanism that facilitates the rapid movement of oxygen from the alveoli into the blood and carbon dioxide in the reverse direction across the respiratory membrane. Scientists at institutions like the National Institutes of Health (NIH) are continuously researching methods to enhance the efficiency of this exchange, particularly in individuals with respiratory diseases like pulmonary fibrosis, which damages the respiratory membrane. Understanding the intricacies of the respiratory membrane will let you grasp how the lungs and other structures work together to keep us alive and kicking.
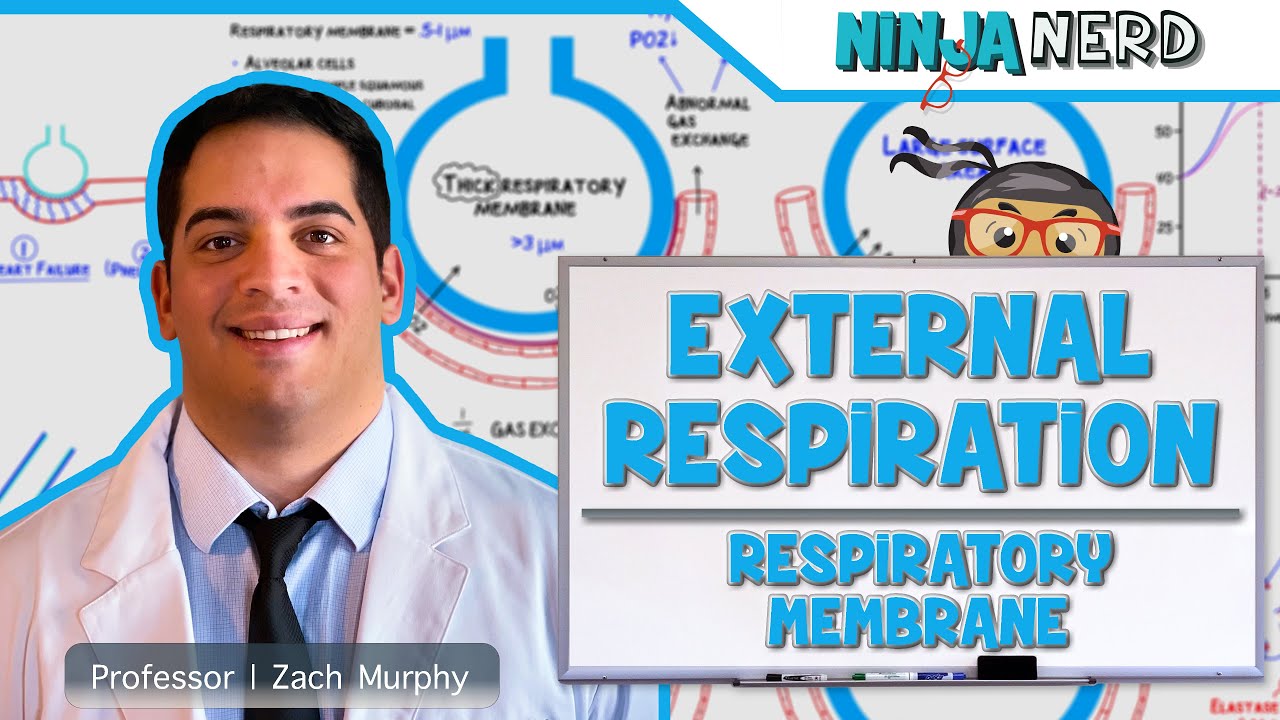
Image taken from the YouTube channel Ninja Nerd , from the video titled Respiratory | External Respiration: Thickness & Surface Area of Respiratory Membrane .
The respiratory system – it's so fundamental to our existence that we often take it for granted.
But have you ever stopped to think about the incredible, intricate processes happening every single second to keep us alive?
At its core, the respiratory system is all about gas exchange: bringing in the oxygen our bodies need to function and expelling the carbon dioxide that's a waste product of those functions.
Think of it as our body's personal air purification and delivery service.
The Lungs: The Hub of Gas Exchange
The lungs are, of course, the stars of the show. These spongy, vital organs are designed for maximum efficiency in gas exchange.
They're not just simple balloons; they're complex structures with a vast network of airways and tiny air sacs called alveoli (we'll dive deeper into those later).
These alveoli are where the magic happens: where oxygen enters our bloodstream and carbon dioxide leaves.
Associated structures include the diaphragm, which contracts to allow the lungs to expand and take in air, and the rib cage, which protects the lungs.
The Respiratory-Circulatory Connection: A Dynamic Duo
It's crucial to understand that the respiratory system doesn't work in isolation.
It's in constant communication and collaboration with the circulatory system.

The circulatory system, with the heart as its engine, is responsible for transporting those vital gases throughout the body.
Oxygen-rich blood travels from the lungs to the tissues, while carbon dioxide-rich blood returns to the lungs to be expelled.
It's a beautiful, tightly coupled system where each component relies on the other for survival.
Understanding the Respiratory System: A Technical Yet Accessible Explanation
The purpose of this article is to delve into the intricacies of this system, providing a technical yet accessible explanation of how it all works.
We'll explore the anatomy, the physiology, and even some of the common problems that can arise.
Our goal is to provide you with a solid understanding of the respiratory system and its vital role in maintaining health.
Anatomy and Physiology of Gas Exchange: The Alveolar-Capillary Unit
The respiratory system – it's so fundamental to our existence that we often take it for granted. But have you ever stopped to think about the incredible, intricate processes happening every single second to keep us alive? At its core, the respiratory system is all about gas exchange: bringing in the oxygen our bodies need to function and expelling carbon dioxide. Let's dive into the amazing microscopic world where this vital exchange occurs: the alveolar-capillary unit.
The Alveoli: Tiny Sacs, Huge Impact
The alveoli are the primary sites of gas exchange in the lungs. Think of them as tiny, balloon-like sacs clustered together. Their design is optimized for one thing: maximizing surface area.
This vast surface area – estimated to be around 70 square meters in humans – allows for incredibly efficient exchange of oxygen and carbon dioxide between the air we breathe and our bloodstream.
It's truly a marvel of biological engineering!
Pulmonary Capillaries: A Close Encounter
Surrounding the alveoli is a dense network of pulmonary capillaries. These tiny blood vessels are in extremely close proximity to the alveolar walls. This proximity is crucial.
It minimizes the distance that gases need to diffuse. The closer, the better when it comes to efficient gas exchange.
The capillaries act as the highway system. They transport oxygen-rich blood away from the lungs and carbon dioxide-rich blood toward them.
The Pneumocytes: Architects of the Alveoli
The alveolar walls are primarily formed by two types of cells called pneumocytes.
Type I pneumocytes, also known as Type I alveolar cells, are thin and flat. Their main job is to form the structure of the alveolar walls.
Then we have Type II pneumocytes, or Type II alveolar cells. These cells have a very special function: they secrete surfactant.
Surfactant: The Secret to Preventing Collapse
Surfactant is a complex mixture of lipids and proteins that reduces surface tension in the alveoli.
Think of it like this: without surfactant, the water molecules lining the alveoli would create a strong surface tension that would cause the tiny sacs to collapse, especially during exhalation.
Surfactant prevents this collapse, allowing the alveoli to remain open and functional. It's absolutely essential for breathing, especially in newborns.
The Interstitium: Supporting Structure
The interstitium is the space between the alveoli and capillaries. This space isn't just empty. It's composed of connective tissue that provides structural support.
This connective tissue contains fibers like collagen and elastin, which give the lungs their elasticity and resilience.
It's like the scaffolding that holds everything together.
Endothelial Cells and the Basement Membrane: Essential Layers
The capillaries are lined by endothelial cells, and these cells are supported by a basement membrane (also called the basal lamina). These structures are essential for maintaining the integrity of the capillary walls.
They provide a barrier that prevents fluid from leaking into the alveoli, which would impair gas exchange.
Red Blood Cells and Hemoglobin: Oxygen Delivery
Finally, we can't forget the red blood cells (erythrocytes) and hemoglobin.
Hemoglobin is the protein inside red blood cells that binds to oxygen. This is how oxygen is transported throughout the body.
Red blood cells are the delivery trucks, and hemoglobin is the container that carries the precious cargo of oxygen.
Similarly, Hemoglobin plays a role in transporting carbon dioxide, although the mechanism is different from oxygen transport.
Without hemoglobin, our cells would quickly run out of oxygen and we wouldn't be able to get rid of the carbon dioxide produced during metabolism.
The alveolar-capillary unit is a finely tuned system. Each component plays a crucial role in facilitating efficient gas exchange.
Understanding the anatomy and physiology of this unit is essential for comprehending how the respiratory system functions and how diseases can impair its function.
Core Respiratory Processes: The Mechanics of Breathing
Just as the intricate structure of the alveoli and capillaries is crucial for gas exchange, the underlying physiological processes are equally vital. These processes dictate how oxygen and carbon dioxide actually move between the air we breathe and our bloodstream. Let's delve into the key mechanisms that make this life-sustaining exchange possible.
Gas Exchange: A Two-Way Street
Gas exchange, in its simplest form, is the movement of oxygen from the alveoli into the blood and carbon dioxide from the blood into the alveoli. This seemingly simple process is governed by several interacting factors. Understanding these factors is essential to grasp how our lungs function effectively.
Diffusion: The Driving Force
At the heart of gas exchange lies diffusion. Diffusion is the spontaneous movement of molecules from an area of high concentration to an area of low concentration. Think of it like dropping a drop of food coloring into water; the color spreads out until it's evenly distributed.
In the lungs, oxygen concentration is higher in the alveoli than in the blood, so oxygen diffuses into the blood. Conversely, carbon dioxide concentration is higher in the blood than in the alveoli, so carbon dioxide diffuses out of the blood.
Partial Pressures: Pressure is Key
To understand diffusion in the context of respiration, it's crucial to grasp the concept of partial pressure. The partial pressure of a gas is the pressure exerted by that individual gas within a mixture of gases. Air, for example, is a mixture of nitrogen, oxygen, carbon dioxide, and other gases.
The partial pressure of oxygen (pO2) and carbon dioxide (pCO2) are key determinants of gas exchange. Oxygen moves from an area of high pO2 (alveoli) to an area of low pO2 (blood). Carbon dioxide moves from an area of high pCO2 (blood) to an area of low pCO2 (alveoli).
This pressure gradient – the difference in partial pressures – drives the movement of gases across the alveolar-capillary membrane.
Ventilation and Perfusion: A Dynamic Duo
While diffusion explains how gases move, ventilation and perfusion explain how much gas is available and how well it's delivered. Ventilation refers to the movement of air into and out of the lungs, bringing fresh oxygen and removing carbon dioxide.
Perfusion refers to the blood flow through the pulmonary capillaries. It brings carbon dioxide to the alveoli and carries oxygen away.
Effective gas exchange requires a harmonious balance between ventilation and perfusion.
V/Q Matching: Getting the Ratio Right
This balance is often described as ventilation-perfusion matching, or V/Q matching. The V/Q ratio represents the relationship between the amount of air reaching the alveoli (ventilation, V) and the amount of blood flowing through the pulmonary capillaries (perfusion, Q).
An ideal V/Q ratio ensures that each alveolus receives enough air to oxygenate the blood flowing past it. Mismatches in V/Q can lead to impaired gas exchange and reduced blood oxygen levels.
Areas with low ventilation relative to perfusion (low V/Q) mean blood passes alveoli that don't have enough oxygen. Areas with high ventilation relative to perfusion (high V/Q) means alveoli have plenty of oxygen, but not enough blood passes to pick it up.
Surface Tension and Surfactant: A Delicate Balance
Finally, let's consider the role of surface tension. The alveoli are lined with a thin film of fluid. This fluid creates surface tension, which tends to collapse the alveoli. Without a counteracting force, the small alveoli would collapse and make it difficult to breathe.
That's where surfactant comes in. Surfactant, produced by Type II alveolar cells, is a substance that reduces surface tension. By reducing surface tension, surfactant prevents alveolar collapse and makes it easier to inflate the lungs. This is crucial for normal breathing.
Key Physiological Properties: Lung Compliance and Its Significance
Core Respiratory Processes: The Mechanics of Breathing
Just as the intricate structure of the alveoli and capillaries is crucial for gas exchange, the underlying physiological processes are equally vital. These processes dictate how oxygen and carbon dioxide actually move between the air we breathe and our bloodstream. Let's delve into the key mechanics of breathing, including a critical factor: lung compliance.
What exactly is lung compliance, and why should you care? Well, it's all about how easily your lungs stretch and expand. Think of it like this: a brand-new balloon is harder to inflate than one that's been blown up a few times. That initial resistance is similar to what we're talking about with lung compliance.
Defining Lung Compliance: The Stretchability Factor
Lung compliance, in the simplest terms, refers to the elasticity of your lungs and chest wall. It's a measure of how much your lung volume changes for a given change in pressure.
A highly compliant lung expands easily with minimal pressure, while a lung with low compliance requires more effort (more pressure) to achieve the same expansion.
Compliance = Change in Volume / Change in Pressure
Basically, it's how much "give" your lungs have.
Pressure-Volume Relationship: Inflation Dynamics
The relationship between pressure and volume is fundamental to understanding lung compliance. When you inhale, your respiratory muscles (diaphragm and intercostal muscles) contract, creating negative pressure within the chest cavity.
This negative pressure "sucks" air into your lungs, causing them to expand.
The amount your lungs expand depends directly on their compliance.
If your lungs are highly compliant, even a small change in pressure will result in a significant increase in volume. If compliance is low, you'll need a much larger pressure change to get the same volume of air in.
Factors Affecting Lung Compliance
Several factors can influence lung compliance:
-
Elasticity of Lung Tissue: The inherent elastic properties of the lung tissue itself play a crucial role. Diseases like emphysema, which destroy lung tissue, can increase compliance (but in a bad way, as the lungs lose their recoil).
-
Surface Tension: The surface tension created by the fluid lining the alveoli tends to collapse the alveoli. Surfactant, a substance produced by the lungs, reduces this surface tension, increasing compliance.
-
Chest Wall Compliance: The stiffness of the chest wall also contributes to overall lung compliance. Conditions like scoliosis or obesity can decrease chest wall compliance, making it harder to breathe.
-
Diseases: Conditions such as pulmonary fibrosis decrease compliance because scarring and stiffening of the lung tissue make it harder for the lungs to expand.
Clinical Significance: Why Compliance Matters
Lung compliance isn't just a theoretical concept; it has significant clinical implications. Doctors measure compliance to assess lung function and diagnose respiratory diseases.
For example, decreased lung compliance is a hallmark of conditions like pulmonary fibrosis and acute respiratory distress syndrome (ARDS). Patients with these conditions often require mechanical ventilation to assist their breathing.
On the other hand, increased lung compliance, as seen in emphysema, can lead to air trapping and reduced gas exchange efficiency.
Understanding lung compliance is crucial for managing respiratory patients and optimizing their treatment. It helps healthcare professionals tailor ventilation strategies and monitor the effectiveness of interventions aimed at improving lung function.
So, next time you take a deep breath, remember the fascinating interplay between pressure, volume, and lung compliance – the "stretchability factor" that keeps you breathing easy.
Pathological Conditions Affecting Gas Exchange: A Clinical Overview
Just as the intricate structure of the alveoli and capillaries is crucial for gas exchange, disruptions to this delicate system can lead to a cascade of respiratory problems. Understanding these pathological conditions is essential for effective diagnosis and treatment.
Let's dive into some common respiratory diseases and explore how they compromise the essential process of gas exchange.
Hypoxia and Hypercapnia: Imbalances in Blood Gases
These are, in essence, the cardinal signs of respiratory distress.
Hypoxia refers to a state of low oxygen levels in the blood and tissues. This can result from various factors:
- Insufficient oxygen intake: Such as at high altitudes or in enclosed spaces.
- Impaired lung function: Caused by diseases that affect gas exchange.
- Circulatory problems: Which prevent oxygen from reaching tissues.
- Anemia: Which impairs oxygen transport.
Hypercapnia, on the other hand, is characterized by elevated carbon dioxide levels in the blood. Its common causes include:
- Inadequate ventilation: Due to lung disease or respiratory muscle weakness.
- Increased carbon dioxide production: Such as during intense exercise or fever.
- Rebreathing exhaled air: As can happen with faulty respiratory equipment.
Both hypoxia and hypercapnia can have severe consequences, affecting multiple organ systems and potentially leading to life-threatening complications.
Infections: The Impact of Pneumonia
Pneumonia, an inflammation of the lungs, often results from bacterial, viral, or fungal infections. The inflammatory response causes:
- Fluid and pus accumulation in the alveoli: Which hinders gas exchange.
- Thickening of the alveolar walls: Further impairing diffusion.
The consequences can be dire, particularly for vulnerable populations like the elderly and immunocompromised individuals.
Pulmonary Edema: The Danger of Fluid Overload
Pulmonary edema occurs when excess fluid accumulates in the air spaces and interstitium of the lungs. This fluid build-up increases the diffusion distance for gases, making it harder for oxygen to reach the bloodstream and for carbon dioxide to be removed.
Common causes include:
- Heart failure: Where the heart's inability to pump effectively leads to fluid backing up into the lungs.
- Kidney failure: Where fluid balance is disrupted.
- Lung injury: From infections or toxins.
COPD and Emphysema: Trapped Air and Alveolar Destruction
Chronic Obstructive Pulmonary Disease (COPD) is an umbrella term for progressive lung diseases, most notably emphysema and chronic bronchitis.
Emphysema is characterized by the destruction of the alveolar walls. This reduces the surface area available for gas exchange.
This destruction also leads to:
- Air trapping: Making it difficult to exhale fully.
- Hyperinflation of the lungs: A hallmark of the disease.
COPD is most often associated with long-term exposure to irritants, primarily cigarette smoke.
Pulmonary Fibrosis: Scarring and Stiffening
Pulmonary fibrosis involves the scarring and thickening of lung tissue. The thickened, stiffened tissue impairs gas exchange by:
- Increasing the diffusion distance: Making it harder for gases to cross the alveolar-capillary membrane.
- Reducing lung compliance: Making it difficult to expand the lungs.
The cause of pulmonary fibrosis is often unknown (idiopathic pulmonary fibrosis), but it can also be associated with autoimmune diseases, environmental exposures, and certain medications.
ARDS: A Syndrome of Acute Lung Injury
Acute Respiratory Distress Syndrome (ARDS) is a severe form of lung injury characterized by widespread inflammation and fluid leakage into the alveoli. This leads to:
- Severe hypoxemia: Despite supplemental oxygen.
- Stiffening of the lungs: Making it difficult to ventilate.
ARDS can be triggered by various insults, including:
- Severe infections (sepsis)
- Trauma
- Aspiration of gastric contents
Asthma: Airway Obstruction and Inflammation
Asthma is a chronic inflammatory disease of the airways characterized by:
- Bronchospasm (constriction of the airways): Which limits airflow.
- Inflammation and mucus production: Which further obstructs airflow.
These factors lead to:
- Wheezing
- Shortness of breath
- Chest tightness
Asthma attacks can be triggered by allergens, irritants, exercise, or respiratory infections.
COVID-19: The Impact of SARS-CoV-2 on the Lungs
The SARS-CoV-2 virus, responsible for COVID-19, can cause significant lung damage, leading to:
- Pneumonia
- ARDS
- Pulmonary fibrosis in some cases
The virus damages the alveolar cells. This triggers an inflammatory response that impairs gas exchange and can lead to long-term respiratory complications.
ILD: A Diverse Group of Lung Disorders
Interstitial Lung Disease (ILD) encompasses a diverse group of lung disorders characterized by:
- Inflammation
- Scarring of the lung tissue
This affects the interstitium, the space between the air sacs. ILD can be caused by a variety of factors, including:
- Autoimmune diseases
- Environmental exposures
- Certain medications
Pulmonary Hypertension: High Blood Pressure in the Lungs
Pulmonary hypertension is a condition characterized by abnormally high blood pressure in the pulmonary arteries. This increased pressure makes it harder for the right side of the heart to pump blood to the lungs.
This leads to:
- Reduced blood flow through the lungs
- Impaired gas exchange
It can result from various factors, including:
- Heart or lung disease
- Blood clots in the lungs
- Genetic factors
NRDS: A Challenge for Premature Infants
Neonatal Respiratory Distress Syndrome (NRDS) primarily affects premature infants. It is caused by a deficiency of surfactant, a substance that reduces surface tension in the alveoli and prevents them from collapsing.
Without sufficient surfactant, the alveoli collapse, making it difficult for the infant to breathe. This leads to:
- Hypoxia
- Respiratory distress
Understanding these pathological conditions is crucial for healthcare professionals to diagnose, treat, and manage respiratory diseases effectively. By targeting the underlying causes and mechanisms of these conditions, we can improve patient outcomes and enhance their quality of life.
Surfactant Replacement Therapy: A Treatment for NRDS
Pathological Conditions Affecting Gas Exchange: A Clinical Overview Just as the intricate structure of the alveoli and capillaries is crucial for gas exchange, disruptions to this delicate system can lead to a cascade of respiratory problems. Understanding these pathological conditions is essential for effective diagnosis and treatment. Let's dive...
Neonatal Respiratory Distress Syndrome (NRDS) is a serious condition that primarily affects premature infants.
These tiny babies often lack a crucial substance in their lungs called surfactant.
Without enough surfactant, their lungs struggle to inflate, making it incredibly difficult to breathe.
Fortunately, there's a treatment called Surfactant Replacement Therapy (SRT) that can make a life-saving difference.
What is Surfactant Replacement Therapy?
SRT is exactly what it sounds like: a way to give babies the surfactant they're missing.
It involves administering a specially formulated surfactant directly into the infant's lungs.
This helps to reduce surface tension in the alveoli, making it easier for the lungs to expand with each breath.
Think of it like this: Imagine trying to inflate a balloon.
If the balloon is dry and sticky, it takes a lot of effort to get it started.
But if you add a little bit of lubricant, it becomes much easier.
Surfactant does the same thing for the lungs.
Why is Surfactant So Important?
Surfactant is a complex mixture of lipids and proteins that is naturally produced in the lungs.
It lines the alveoli, the tiny air sacs where gas exchange occurs.
By reducing surface tension, surfactant prevents the alveoli from collapsing at the end of each breath.
Without it, the lungs become stiff and difficult to inflate, leading to respiratory distress.
How is Surfactant Replacement Therapy Administered?
The procedure is relatively straightforward.
A thin tube called an endotracheal tube is inserted into the infant's trachea (windpipe).
The surfactant is then administered through the tube directly into the lungs.
The baby is closely monitored throughout the procedure to ensure they are tolerating the treatment well.
Multiple doses may be necessary, depending on the infant's response.
Benefits of Surfactant Replacement Therapy
The benefits of SRT are significant and well-documented.
It improves lung function, reduces the severity of respiratory distress, and decreases the need for mechanical ventilation.
SRT also reduces the risk of complications associated with NRDS, such as pneumothorax (collapsed lung) and bronchopulmonary dysplasia (chronic lung disease).
Overall, SRT has dramatically improved the survival rates of premature infants with NRDS.
Potential Risks and Side Effects
While SRT is generally safe, like any medical procedure, it does carry some potential risks.
These can include:
- Temporary drop in oxygen saturation
- Slow heart rate
- Airway obstruction
It's important to note that these side effects are usually mild and transient.
The benefits of SRT far outweigh the risks for most infants with NRDS.
Types of Surfactant Used in SRT
There are two main types of surfactant used in SRT:
- Natural surfactant: Derived from animal lungs (usually cows or pigs). These contain a variety of lipids and proteins, closely resembling human surfactant.
- Synthetic surfactant: Manufactured in the laboratory. These typically contain a mixture of synthetic lipids and proteins.
Both types of surfactant have been shown to be effective in treating NRDS.
The choice of surfactant may depend on availability, cost, and the individual needs of the infant.
Surfactant Replacement Therapy is a cornerstone of treatment for Neonatal Respiratory Distress Syndrome.
By providing the crucial substance that premature infants lack, SRT significantly improves lung function, reduces complications, and increases survival rates.
While potential risks exist, they are generally outweighed by the profound benefits this therapy offers to vulnerable newborns.
Modeling the Respiratory System: Using Mathematical Tools
Just as understanding the structure and function of the respiratory system is vital, mathematical models provide a powerful way to simulate its complex processes and predict its behavior under various conditions. These models are increasingly important in respiratory research and clinical practice.
The Role of Mathematical Models in Respiratory Research
Mathematical models are invaluable tools in respiratory research. They allow scientists to explore the complex interactions within the respiratory system in a way that would be difficult or impossible with experimental methods alone.
Simulating Complex Interactions
These models can simulate gas exchange, airflow dynamics, and the mechanical properties of the lungs. This helps researchers understand how these factors interact to determine overall respiratory function.
Exploring "What-If" Scenarios
Models allow researchers to test hypotheses and explore "what-if" scenarios. This can provide insights into the effects of diseases, treatments, and environmental factors on the respiratory system.
Simulating Gas Exchange: A Deeper Dive
One of the primary uses of mathematical models is to simulate the process of gas exchange in the lungs.
Modeling Alveolar Gas Exchange
Models can represent the diffusion of oxygen and carbon dioxide across the alveolar-capillary membrane. They take into account factors such as partial pressures, membrane thickness, and surface area.
Predicting Blood Gas Levels
By simulating gas exchange, these models can predict how changes in ventilation, perfusion, or membrane properties will affect arterial blood gas levels. This information is critical for understanding the pathophysiology of respiratory diseases.
Predicting the Effects of Various Factors on Respiratory Function
Mathematical models can also be used to predict how various factors, such as lung disease, altitude, or exercise, affect respiratory function.
Simulating the Effects of Lung Diseases
Models can simulate the effects of diseases like emphysema, pulmonary fibrosis, and asthma on lung mechanics and gas exchange. This can help researchers understand how these diseases impair respiratory function.
Modeling Responses to Exercise and Altitude
Mathematical models are useful tools for simulating the physiological responses to exercise and altitude. For example, they can predict the changes in ventilation, blood flow, and oxygen uptake that occur during exercise. They can also predict the effects of altitude on oxygen saturation and ventilation.
Advantages of Using Mathematical Models
There are several advantages to using mathematical models in respiratory research:
- They can provide insights into complex physiological processes.
- They can be used to test hypotheses and explore "what-if" scenarios.
- They can reduce the need for animal experiments.
- They can be used to develop new diagnostic and therapeutic strategies.
Limitations of Mathematical Models
While mathematical models are powerful tools, they also have limitations:
- They are only as good as the data and assumptions on which they are based.
- They may not capture all of the complexity of the respiratory system.
- They require expertise in mathematical modeling and respiratory physiology.
Despite these limitations, mathematical models are becoming increasingly important in respiratory research and clinical practice. As models become more sophisticated and data become more readily available, they will play an even greater role in advancing our understanding of respiratory function and disease.
Video: Respiratory Membrane: Lung Function & Gas Exchange
FAQs: Respiratory Membrane, Lung Function & Gas Exchange
What is the respiratory membrane and why is it important?
The respiratory membrane is the extremely thin structure in the lungs where gas exchange occurs. It's made of the alveolar and capillary walls.
Oxygen passes from the alveoli into the blood, and carbon dioxide moves from the blood into the alveoli, all across this membrane. Its thinness is crucial for efficient diffusion.
How does the respiratory membrane facilitate gas exchange?
The respiratory membrane's thinness allows for simple diffusion of gases. Gases move from areas of high concentration to areas of low concentration.
This concentration gradient, combined with the respiratory membrane's large surface area, drives efficient oxygen uptake and carbon dioxide removal.
What factors affect the efficiency of gas exchange at the respiratory membrane?
Several factors impact efficiency, including membrane thickness (thickening reduces efficiency), surface area (reduced area lessens gas exchange), and ventilation-perfusion matching (mismatch hinders gas exchange).
Lung diseases like emphysema can damage the respiratory membrane, affecting its surface area and the body's ability to absorb oxygen.
What are the main components of the respiratory membrane?
The respiratory membrane mainly consists of the alveolar epithelium (lining of the alveoli) and the capillary endothelium (lining of the blood capillaries).
These two very thin layers, along with their fused basement membrane, create the barrier across which oxygen and carbon dioxide must diffuse. The respiratory membrane structure is essential for healthy lung function.
So, next time you take a deep breath, give a little nod to your amazing respiratory membrane. It's quietly working away, making sure that vital oxygen gets to where it needs to go and that carbon dioxide gets out. Pretty cool, right?