What is Load Resistance? Circuit Guide
Electronic circuits represent a complex, yet fascinating, field where understanding component functions is critical, and one such component is load resistance. Ohm's Law, a foundational principle, dictates the relationship between voltage, current, and resistance within a circuit, influencing how power is delivered to the load. The National Electrical Manufacturers Association (NEMA) provides standards for ensuring safe and efficient circuit design, and their guidelines often refer to optimal load matching. Electrical engineers frequently use a multimeter to measure resistance and analyze circuit behavior, which is crucial in determining if the load is functioning as intended. Therefore, a comprehensive circuit guide must address the question of what is load resistance and its practical implications for performance.
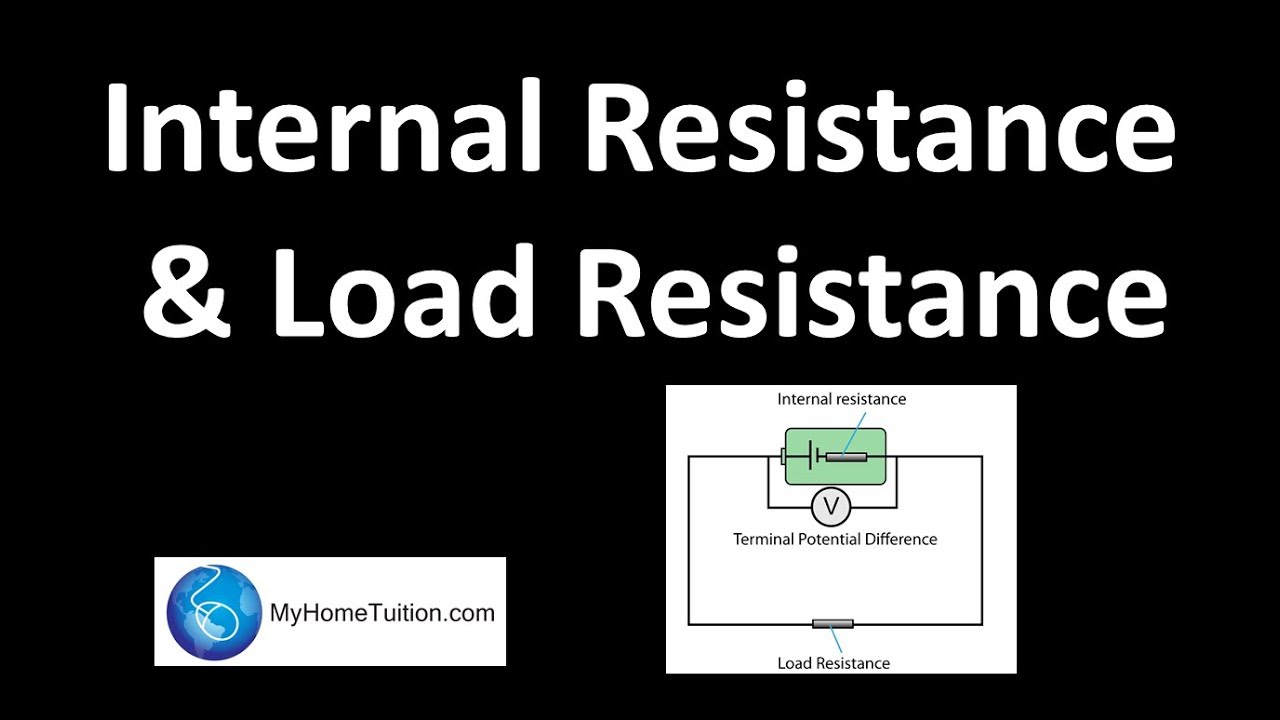
Image taken from the YouTube channel myhometuition , from the video titled Internal Resistance and Load Resistance | Electricity .
Electrical circuits are the unseen backbone of our modern world. From the smartphones in our pockets to the complex machinery that drives industries, electrical circuits are integral.
They are the unsung heroes that power our lives and enable technological advancements.
Understanding the basic principles of electrical circuits is not just for engineers and scientists. It's a valuable asset for anyone interested in electronics, DIY projects, or simply understanding how the technology around them works.
Why Learn About Electrical Circuits?
-
Ubiquitous Presence: Electrical circuits are everywhere, making a basic understanding universally beneficial.
-
Empowerment: Knowledge empowers you to troubleshoot, repair, and create your own electronic projects.
-
Foundation: A solid understanding of basic circuit principles forms the foundation for more advanced studies in electronics, engineering, and computer science.
What is an Electrical Circuit?
An electrical circuit is a closed path that allows electrical current to flow from a source to a load, and back to the source. This flow is facilitated by conductive materials, typically wires made of copper or aluminum.
The essential components of a circuit include:
-
A Power Source: Provides the electrical energy, such as a battery or power outlet.
-
Conductive Path: Wires or traces that allow the current to flow.
-
Load: A component that consumes the electrical energy, such as a light bulb, motor, or electronic device.
-
Switch (Optional): Controls the flow of current, turning the circuit on or off.
Basic Concepts We Will Cover
This section will guide you through the fundamental concepts that are essential for understanding how electrical circuits work.
We will start with the core principles of voltage, current, and resistance, and how they relate to each other through Ohm's Law.
We will explore the different ways circuits can be configured, such as series and parallel circuits, and how to calculate total resistance and current in each configuration.
We will also introduce common circuit faults like short circuits and open circuits, and how to prevent them.
Finally, we will touch on the concept of load matching and impedance considerations, and look at some essential components such as resistors, light bulbs, motors, speakers, and heaters.
Through these topics, you will gain the knowledge and skills to analyze, design, and troubleshoot basic electrical circuits.
Let's begin this exciting journey into the world of electrical circuits!
Understanding the core principles of voltage, current, and resistance is fundamental to grasping how electrical circuits function. These three concepts are the building blocks upon which all circuit analysis and design are based. This section will demystify these concepts, providing you with a solid foundation for further exploration.
Core Principles: Voltage, Current, and Resistance
Let's dive into each of these critical elements.
Voltage: The Driving Force
Voltage, often described as electrical potential difference, is the "push" or force that causes electrons to move through a circuit. It's analogous to the pressure in a water pipe that drives the flow of water. Voltage is measured in volts (V).
Think of a battery; it creates a voltage difference between its terminals, which then forces electrons to flow when a circuit is connected. Without voltage, there is no current flow. It's the prime mover of electrical activity.
Current: The Flow of Charge
Current is the rate of flow of electrical charge through a circuit. It represents the number of electrons passing a given point per unit of time. Current is measured in amperes (A), often shortened to amps.
The higher the current, the more electrons are flowing. Like the amount of water flowing through a pipe, current indicates the magnitude of electrical activity. It's directly linked to voltage and inversely linked to resistance as explained by Ohm's Law.
Resistance: Opposition to Flow
Resistance is the opposition to the flow of electric current within a circuit. It impedes the movement of electrons, converting electrical energy into other forms, such as heat. Resistance is measured in ohms (Ω).
Every material offers some degree of resistance, but components called resistors are specifically designed to provide a precise amount of resistance. These are crucial in controlling current and voltage levels within a circuit.
Factors Affecting Resistance
Several factors influence a material's resistance:
-
Material: Conductors (like copper) have low resistance, while insulators (like rubber) have high resistance.
-
Length: Longer conductors have higher resistance.
-
Cross-sectional Area: Thicker conductors have lower resistance.
-
Temperature: For most materials, resistance increases with temperature.
Types of Resistors
Resistors come in various forms, each suitable for different applications:
-
Carbon Film Resistors: Common, general-purpose resistors.
-
Wire-Wound Resistors: Used for high-power applications.
-
Surface Mount Resistors (SMD): Small resistors for modern circuit boards.
Choosing the right type of resistor is important for achieving the desired circuit performance and reliability.
Ohm's Law Explained: The Cornerstone Relationship
Ohm's Law describes the relationship between voltage (V), current (I), and resistance (R). It is expressed as the equation:
V = IR
This simple equation is one of the most fundamental laws in electronics. It can be rearranged to solve for any of the three variables:
I = V/R
R = V/I
Ohm's Law allows you to calculate unknown values in a circuit if you know the other two. It's an indispensable tool for both circuit design and troubleshooting.
Practical Examples of Using Ohm's Law
Here are a couple of examples to illustrate Ohm's Law:
Example 1:
A circuit has a 12V power source and a 100Ω resistor. Calculate the current flowing through the circuit.
I = V/R = 12V / 100Ω = 0.12A or 120mA
Example 2:
A circuit has a current of 0.5A and a resistance of 20Ω. Calculate the voltage across the resistor.
V = IR = 0.5A
**20Ω = 10V
Practice Problems
Test your understanding with these problems:
-
A resistor has a voltage of 5V across it, and the current through it is 0.1A. What is the resistance value?
-
A 9V battery is connected to a 470Ω resistor. What is the current flowing through the resistor?
Solutions:
-
R = V/I = 5V / 0.1A = 50Ω
-
I = V/R = 9V / 470Ω ≈ 0.019A or 19mA
Mastering Ohm's Law is crucial for understanding more complex circuit behaviors.
Electrical Power: Calculation and Dissipation
Electrical power is the rate at which electrical energy is transferred or consumed in a circuit. It is measured in**watts (W)
**.
Understanding electrical power is essential for designing efficient and safe circuits.
Power Formulas
Power can be calculated using several formulas:
-
P = VI (Power equals Voltage times Current)
-
P = I2R (Power equals Current squared times Resistance)
-
P = V2/R (Power equals Voltage squared divided by Resistance)
These formulas are derived from Ohm's Law and provide different ways to calculate power depending on the available information.
Power Dissipation in Resistors
Resistors dissipate electrical power as heat. The amount of heat generated depends on the power rating of the resistor and the actual power dissipated. It's important to select resistors with appropriate power ratings to avoid overheating and component failure.
If a resistor dissipates more power than its rating, it can overheat, leading to altered resistance values or complete failure.
Examples of Power Calculation
Let's illustrate power calculations with examples:
Example 1:
A 100Ω resistor has a current of 0.2A flowing through it. Calculate the power dissipated by the resistor.
P = I2R = (0.2A)2** 100Ω = 4W
Example 2:
A 12V power source is connected to a 200Ω resistor. Calculate the power dissipated by the resistor.
P = V2/R = (12V)2 / 200Ω = 0.72W
Selecting Resistors with Appropriate Power Ratings
It's crucial to choose resistors with a power rating significantly higher than the expected power dissipation. A common practice is to select resistors with a power rating at least twice the calculated power dissipation. This provides a safety margin and ensures the resistor operates within its specifications.
For example, if a resistor is expected to dissipate 0.5W, selecting a 1W resistor would be a safe choice. Paying attention to this detail will improve the reliability and longevity of the circuits.
By understanding voltage, current, resistance, and Ohm's Law, you are equipped with the foundational knowledge required to analyze and design basic electrical circuits. You now have the key to unlocking the complexities of more intricate circuit designs.
Series and Parallel Circuits: Building Blocks of Complexity
Having established a firm understanding of voltage, current, and resistance, we now turn our attention to how these elements interact within circuit configurations. The two fundamental ways to connect components are in series and in parallel. These configurations dictate how current and voltage are distributed throughout the circuit, profoundly impacting its overall behavior.
Series Circuits: Current Remains Constant
In a series circuit, components are connected sequentially, forming a single, unbroken path for the electric current. Imagine a single lane road; all traffic must flow through each point along the way.
A crucial characteristic of a series circuit is that the current is the same at every point. There is no branching or alternative path for the electrons to take.
Because of this, every component within the series experiences the same current flow. Understanding this characteristic is essential for designing and troubleshooting series circuits.
Calculating Total Resistance in Series Circuits
To determine the total resistance in a series circuit, simply add the individual resistances of each component:
Rtotal = R1 + R2 + ... + Rn
Where R1, R2, and Rn represent the resistance of each individual resistor in the series. The total resistance is the sum of all resistance values.
The total resistance determines the total current in a series circuit. With the total voltage, using Ohm's Law becomes straightforward.
Calculating Total Current in Series Circuits
Once the total resistance (Rtotal) is known, the total current (Itotal) can be calculated using Ohm's Law:
Itotal = Vtotal / Rtotal
Where Vtotal is the total voltage applied across the series circuit. Remember, the current remains constant throughout the entire series circuit.
Practical Examples of Series Circuits
Consider traditional Christmas lights. In older sets, if one bulb burns out, the entire string goes dark. This is because the bulbs are wired in series; the broken filament creates an open circuit, stopping current flow through the whole string.
Another practical example is a voltage divider. A voltage divider uses multiple resistors in series to create a specific voltage at a particular point in the circuit. This is a common technique in electronics to provide different voltage levels from a single voltage source.
Parallel Circuits: Voltage Remains Constant
In a parallel circuit, components are connected side-by-side, providing multiple paths for the current to flow. Think of a multi-lane highway; vehicles can choose different lanes to reach their destination.
The defining characteristic of a parallel circuit is that the voltage across each component is the same. Each component is directly connected to the voltage source.
Components in a parallel circuit share the same voltage. This principle is fundamental in understanding and designing parallel circuits.
Calculating Total Resistance in Parallel Circuits
Calculating total resistance in a parallel circuit is a bit more involved. The reciprocal of the total resistance is equal to the sum of the reciprocals of the individual resistances:
1/Rtotal = 1/R1 + 1/R2 + ... + 1/Rn
After calculating the reciprocal of the total resistance, remember to take the reciprocal of the result to find the actual Rtotal value. Many find this step to be a common area of error.
This is because total resistance in a parallel circuit is always less than the smallest individual resistance.
Calculating Total Current in Parallel Circuits
In a parallel circuit, the total current (Itotal) is the sum of the currents flowing through each branch:
Itotal = I1 + I2 + ... + In
Where I1, I2, and In are the currents through each individual branch. Each current can be calculated using Ohm's Law (I = V/R), where V is the constant voltage across all branches.
Practical Examples of Parallel Circuits
Household wiring is a prime example of a parallel circuit. All appliances and lights are connected in parallel, ensuring that each receives the standard household voltage (e.g., 120V in the US).
If one appliance is switched off (breaking its branch), the other appliances continue to function because the voltage across them remains unaffected. Another example is an LED array, where LEDs are often connected in parallel (with current-limiting resistors) to ensure each receives the necessary voltage.
Calculating Total Resistance and Current: Practical Application
To solidify your understanding, let's work through a few examples.
Example 1: Series Circuit
A series circuit consists of a 100Ω resistor, a 220Ω resistor, and a 330Ω resistor connected to a 9V battery. Calculate the total resistance and total current.
Rtotal = 100Ω + 220Ω + 330Ω = 650Ω
Itotal = 9V / 650Ω ≈ 0.0138A or 13.8mA
Example 2: Parallel Circuit
A parallel circuit consists of a 470Ω resistor and a 1kΩ resistor connected to a 5V power supply. Calculate the total resistance and the total current.
1/Rtotal = 1/470Ω + 1/1000Ω ≈ 0.00313
Rtotal ≈ 1 / 0.00313 ≈ 319.5Ω
I1 = 5V / 470Ω ≈ 0.0106A
I2 = 5V / 1000Ω = 0.005A
Itotal = 0.0106A + 0.005A = 0.0156A or 15.6mA
Combined Series-Parallel Circuits
Many real-world circuits combine both series and parallel elements. Analyzing these requires breaking down the circuit into smaller, manageable sections.
First, identify series and parallel combinations and simplify them by calculating equivalent resistances. Then, redraw the circuit with these simplifications and repeat the process until you have a single equivalent resistance.
From there, you can use Ohm's Law to determine the total current and then work backward to find the voltage and current at various points in the original circuit.
Mastering series and parallel circuit analysis is critical for understanding more complex electronic systems. These fundamental concepts are the foundation upon which more advanced circuit designs are built.
Common Circuit Faults: Short Circuits and Open Circuits
Electrical circuits, while incredibly useful, are not without their vulnerabilities. Among the most common issues encountered are short circuits and open circuits, both of which can disrupt the intended operation and, in some cases, pose significant safety risks. Understanding these faults, their causes, and preventative measures is paramount for anyone working with electrical systems.
Understanding Short Circuits: The Path of Least Resistance
A short circuit occurs when an unintended path of very low resistance is created in a circuit. This bypasses the intended circuit components, allowing current to flow freely along this new, easier route.
Since resistance is inversely proportional to current (Ohm's Law), the result is a large, uncontrolled current flow. This surge of current can have several detrimental consequences.
The Dangers of Uncontrolled Current
The dangers of short circuits cannot be overstated. The excessive current generates heat, which can lead to:
- Overheating: Components, wires, and insulation can overheat, potentially melting or igniting.
- Component Damage: Sensitive electronic components are often destroyed by the excessive current.
- Fire Hazards: Overheated insulation or flammable materials in close proximity can easily ignite, leading to a fire.
- Electrical Shock: Short circuits can expose conductive surfaces, increasing the risk of electrical shock if touched.
Understanding Open Circuits: A Disconnected Path
In contrast to a short circuit, an open circuit represents a break in the intended circuit path. This break prevents the flow of current altogether.
Imagine a severed wire; the circuit is incomplete, and electricity cannot flow. While often less immediately dangerous than short circuits, open circuits can still have significant impacts.
Consequences of Interrupted Current
The primary consequence of an open circuit is a non-functional circuit. The intended operation is disrupted, and the connected device or system will fail to work.
This can range from a light bulb not turning on to a more complex electronic system shutting down entirely. Furthermore, in systems with multiple interconnected circuits, an open circuit in one area can indirectly affect the operation of other parts.
Preventing Circuit Faults: Proactive Measures
Fortunately, both short circuits and open circuits can be prevented through careful design, installation, and maintenance practices.
Proactive prevention is always better than reactive troubleshooting.
Best Practices for Avoiding Issues
Several key strategies can significantly reduce the risk of these common circuit faults:
- Proper Wiring Techniques: Ensure all connections are secure, wires are properly insulated, and wiring practices adhere to established standards.
- Fuses and Circuit Breakers: These devices are designed to interrupt the circuit when an overcurrent condition (like a short circuit) is detected.
- Regular Inspections: Periodically inspect wiring, connections, and components for signs of wear, damage, or loose connections.
- Avoid Overloading Circuits: Do not exceed the rated current capacity of circuits or outlets, as this can lead to overheating and potential short circuits.
The Role of Overcurrent Protection
Fuses and circuit breakers are essential safety devices that protect circuits from overcurrent conditions. They work by interrupting the flow of electricity when the current exceeds a predetermined limit.
- Fuses: These contain a thin wire that melts and breaks the circuit when the current is too high. Fuses are single-use devices and must be replaced after they blow.
- Circuit Breakers: These use an electromechanical mechanism to trip and break the circuit. Circuit breakers can be reset and reused, making them more convenient than fuses in many applications.
By understanding the nature of short circuits and open circuits, and by implementing preventative measures, it's possible to significantly reduce the risk of these common circuit faults, ensuring the safe and reliable operation of electrical systems.
Load Matching: Optimizing Power Transfer
In the realm of electrical engineering, maximizing efficiency is often a primary goal. One crucial aspect of achieving this is through load matching, a technique that ensures the most effective transfer of power from a source to its load. Understanding load matching is essential for anyone seeking to optimize the performance of electronic systems.
Defining Load Matching: Aligning Impedances
Load matching, at its core, is the process of equating the impedance of the load to the internal impedance of the source.
Think of it as tuning a musical instrument to resonate perfectly; when impedances are matched, the power transfer reaches its peak efficiency.
The Role of Impedance
While resistance is a familiar concept, impedance is a more encompassing term. It includes not only resistance but also reactance, which arises from capacitive and inductive effects.
Impedance, denoted by 'Z', is a measure of the opposition to alternating current (AC) flow. It encompasses resistance, inductive reactance (from inductors), and capacitive reactance (from capacitors).
Consequences of Impedance Mismatch
When the load's impedance doesn't align with the source's internal impedance, several undesirable effects can occur.
- Reduced Power Transfer: A significant portion of the available power is lost, resulting in inefficiency.
- Signal Reflections: Energy bounces back towards the source, causing distortion and potential damage.
- Distortion: The signal waveform can be altered, leading to inaccurate or unreliable operation.
- Potential Damage: In some cases, the mismatch can cause overheating or other forms of damage to the source or load.
Applications of Load Matching
Load matching is not merely a theoretical concept; it has widespread practical applications across various electronic devices.
Audio Amplifiers
In audio systems, matching the output impedance of an amplifier to the impedance of the speakers is crucial. This ensures that the speakers receive the maximum power, resulting in optimal sound reproduction.
Typically, audio amplifiers are designed to drive speakers with specific impedance ratings (e.g., 4 ohms, 8 ohms).
Radio Transmitters
Radio transmitters rely heavily on load matching to efficiently transmit signals.
The antenna's impedance must be carefully matched to the transmitter's output impedance to prevent signal reflections and maximize the radiated power.
Techniques like antenna tuning and impedance matching networks are commonly employed.
Data Transmission Lines
In high-speed data communication, impedance matching prevents signal reflections that can corrupt data.
Transmission lines, such as coaxial cables, are designed with a characteristic impedance (e.g., 50 ohms, 75 ohms). Terminating the line with a matching impedance at the receiving end minimizes reflections and ensures reliable data transfer.
Essential Components: Building Blocks of Electrical Circuits
Electrical circuits, at their heart, are composed of individual components working in harmony. Understanding the function and variety of these building blocks is fundamental to grasping how circuits operate.
Let's delve into some essential components: resistors, light bulbs, motors, speakers, and heaters, exploring their unique roles and applications within the electrical landscape.
Resistors: Masters of Current Control
Resistors are arguably the most fundamental passive components in electronics. Their primary role is to control the flow of electric current within a circuit.
They introduce a specific amount of resistance, measured in ohms (Ω), which opposes the current and limits its magnitude. This control is essential for protecting other components and shaping circuit behavior.
The Resistor Color Code: Unlocking Resistance Values
The resistor color code is a standardized system for indicating a resistor's resistance value and tolerance using colored bands.
Each color represents a specific numerical digit or multiplier. Learning to decode the color bands enables you to quickly identify a resistor's properties.
Tolerance indicates the acceptable range of variation from the stated resistance value. A resistor with a 5% tolerance, for example, may have an actual resistance that deviates by up to 5% from its nominal value.
Types of Resistors and Their Applications
Resistors come in a variety of types, each suited for specific applications. Carbon film resistors are common, general-purpose resistors used in many electronic circuits.
Wire-wound resistors offer higher power ratings and are used in applications requiring greater heat dissipation. Potentiometers, also known as variable resistors, allow you to adjust the resistance value, making them ideal for volume controls or adjustable voltage dividers.
Light Bulbs: Illumination Through Resistance
Light bulbs, in their essence, are resistive loads that convert electrical energy into light. When current flows through the bulb's filament, the filament heats up to a high temperature, emitting light.
However, light bulbs do more than just light up a room. They also act as components within a circuit.
A light bulb will drop the voltage, which is especially important to consider when troubleshooting circuits.
Voltage Dropping and Current Limiting
As current flows through the bulb, the light bulb limits the amount of current based on the properties of the filament. Without enough current, the bulb will not reach its maximum light output.
A Spectrum of Light Bulb Technologies
The world of light bulbs has evolved significantly over the years. Traditional incandescent bulbs are known for their warm light but are energy-inefficient.
LEDs (light-emitting diodes) are highly efficient and long-lasting, making them the preferred choice for many applications. Fluorescent bulbs offer better energy efficiency than incandescent bulbs, and have traditionally been used in commercial spaces.
Motors: Harnessing Electrical Energy for Motion
Motors are electromechanical devices that convert electrical energy into mechanical motion. They are the driving force behind countless machines, from household appliances to industrial equipment.
Driving Loads and Generating Torque
Motors connect directly into circuits, receiving electrical power and transforming it into rotational force, or torque.
This torque is used to drive various loads, such as fans, pumps, and machinery.
A Motor Menagerie: Different Types for Different Needs
Motors come in various types, each suited for specific applications. DC motors are commonly used in battery-powered devices and offer good speed control.
AC motors are the workhorses of industrial applications, known for their reliability and high power output. Stepper motors provide precise positional control, making them ideal for robotics and automation.
Speakers: Transforming Electrical Signals into Sound
Speakers are transducers that convert electrical energy into audible sound waves. They are integral components in audio systems, reproducing music, speech, and other audio signals.
Amplifying and Reproducing Audio Signals
Speakers function as transducers, which means that they convert audio signals through the electromagnetic coil, membrane, and into sound waves that our ears can understand.
Amplifiers have a big role to play in producing signals through these speakers.
Dynamic vs. Electrostatic Speakers
Speakers can be different sizes, but they also contain different technologies as well. Two popular technologies are: dynamic and electrostatic speakers.
Both have their own quirks, and use cases that depend on the listener.
Heaters: Providing Warmth and Generating Heat
Heaters are resistive loads that convert electrical energy into heat. They are widely used for space heating, industrial processes, and various other applications requiring controlled heat generation.
Generating Heat for Diverse Applications
Heaters serve a variety of purposes, from keeping homes warm during the winter to providing heat for industrial processes.
There are many different types of heaters to suit varying needs and requirements.
Resistive vs. Ceramic Heaters
Two common types of heaters include resistive heaters and ceramic heaters. Resistive heaters use a resistive element, such as a wire or coil, to generate heat.
Ceramic heaters utilize ceramic materials, which offer good heat conductivity and thermal stability. Each type has its own set of advantages and disadvantages.
Measurements and Applications: Bringing Theory to Life
The knowledge of electrical circuits, while intellectually stimulating, truly comes alive when applied in the real world. This involves not only understanding the underlying principles but also mastering the art of measurement and analysis. This section explores how to use multimeters for practical measurements and how to apply circuit analysis techniques to solve real-world problems.
Mastering the Multimeter: Your Window into the Circuit
The multimeter is an indispensable tool for anyone working with electrical circuits. It allows you to measure voltage, current, and resistance, providing crucial insights into circuit behavior.
Let's explore how to use this versatile instrument effectively and safely.
Measuring Voltage: Understanding Potential Difference
Voltage, measured in volts (V), represents the potential difference between two points in a circuit. To measure voltage, the multimeter must be connected in parallel with the component or section of the circuit you wish to analyze.
- Set the multimeter to the voltage (V) setting. Ensure you select the appropriate voltage type (DC or AC) based on the circuit you're measuring.
- Connect the black (negative) probe to the ground or common reference point in the circuit.
- Connect the red (positive) probe to the point where you want to measure the voltage.
- Read the voltage value displayed on the multimeter.
Measuring Current: Tracking the Flow of Charge
Current, measured in amperes (A), represents the flow of electric charge through a circuit. To measure current, the multimeter must be connected in series with the circuit, meaning you need to break the circuit and insert the multimeter in the path of the current flow.
- Turn off the power to the circuit.
- Set the multimeter to the current (A) setting. Make sure to select the appropriate current range (mA or A) and current type (DC or AC).
- Break the circuit at the point where you want to measure the current.
- Connect the black (negative) probe to one end of the break and the red (positive) probe to the other end, completing the circuit through the multimeter.
- Turn the power back on to the circuit.
- Read the current value displayed on the multimeter.
Measuring Resistance: Quantifying Opposition to Flow
Resistance, measured in ohms (Ω), quantifies the opposition to current flow in a component or circuit. To measure resistance, the component must be isolated from the rest of the circuit, as other components can affect the reading.
- Turn off the power to the circuit.
- Remove the component you want to measure from the circuit.
- Set the multimeter to the resistance (Ω) setting.
- Connect the probes to the two ends of the component.
- Read the resistance value displayed on the multimeter.
Essential Safety Precautions: Prioritizing Your Well-being
Working with electrical circuits can be hazardous if proper safety precautions are not followed. Always exercise caution and adhere to the following guidelines:
-
Never work on live circuits unless absolutely necessary. If you must work on a live circuit, use extreme caution and wear appropriate personal protective equipment (PPE), such as insulated gloves and eye protection.
-
Avoid high-voltage circuits. High voltages can be lethal. If you are not experienced with high-voltage circuits, seek assistance from a qualified electrician.
-
Use the correct settings and probes on the multimeter. Using the wrong settings or probes can damage the multimeter or lead to inaccurate readings.
-
Be aware of your surroundings. Make sure you have adequate lighting and a clear workspace. Avoid working in wet or damp environments.
Practical Circuit Analysis: Applying Knowledge to Solve Problems
Understanding circuit analysis techniques allows you to predict circuit behavior, design circuits for specific applications, and troubleshoot problems effectively.
Analyzing Simple Circuits: Ohm's Law in Action
Ohm's Law (V = IR) is the cornerstone of circuit analysis. It allows you to calculate the voltage, current, or resistance in a circuit if you know the other two values.
For example, if you have a 12V power supply connected to a 100Ω resistor, you can use Ohm's Law to calculate the current flowing through the resistor: I = V/R = 12V / 100Ω = 0.12A.
Designing Basic Circuits: Building Functional Systems
Circuit analysis techniques can be used to design circuits for specific applications. For example, you can design a voltage divider to create a lower voltage from a higher voltage source. This is commonly used to power sensitive electronic components that require a lower voltage than the main power supply.
Troubleshooting Common Problems: Diagnosing and Repairing
Multimeters and circuit analysis techniques are invaluable for troubleshooting circuit problems. By measuring voltages, currents, and resistances at different points in the circuit, you can identify open circuits, short circuits, and component failures.
For example, if a circuit is not working, you can use a multimeter to check for voltage at different points in the circuit. If there is no voltage, there may be an open circuit. If the voltage is too low, there may be a short circuit or a faulty component.
The ability to measure and analyze electrical circuits is essential for anyone seeking to apply their knowledge in practical settings. By mastering the use of multimeters and understanding circuit analysis techniques, you can unlock a world of possibilities, from designing custom circuits to troubleshooting complex electronic systems. The journey from theoretical knowledge to practical application is a rewarding one, empowering you to bring your ideas to life and solve real-world problems.
Video: What is Load Resistance? Circuit Guide
Frequently Asked Questions About Load Resistance
What exactly is load resistance in an electrical circuit?
Load resistance refers to the electrical resistance presented by the component or circuit that receives power from a source. It determines how much current flows through the circuit for a given voltage. Essentially, what is load resistance dictates how hard the power source has to work.
Why is understanding load resistance important when designing circuits?
Knowing the load resistance is critical for proper circuit design because it affects power consumption, voltage levels, and component selection. A mismatch between the source and load resistance can lead to inefficiencies or even damage to circuit components.
How does changing the load resistance affect the current in a circuit?
According to Ohm's Law, the current in a circuit is inversely proportional to the resistance. So, if you decrease the load resistance, the current will increase, assuming the voltage remains constant. What is load resistance is therefore very influential on circuit behavior.
Can a load resistance be too high or too low, and what happens if it is?
Yes, a load resistance can be too high or too low. If it's too high, the current will be very low, potentially preventing the load from functioning correctly. If what is load resistance is too low, excessive current can flow, leading to overheating and potential damage to the power source or other components.
So, there you have it! Hopefully, you've now got a solid grasp on what is load resistance and how it plays a vital role in circuit behavior. It might seem a little daunting at first, but with a bit of practice, you'll be optimizing power transfer like a pro in no time. Happy circuit building!