Ka of HCN: Acidity, Value & Understanding
The dissociation constant, or Ka, represents a fundamental property of acids in aqueous solutions, and for hydrogen cyanide, HCN, the Ka of HCN
dictates its behavior as a weak acid. Its value is a crucial parameter when assessing the potential hazards associated with cyanide exposure. Quantitatively, the Ka of HCN
informs calculations pertinent to acid-base chemistry in diverse fields, including industrial processes and laboratory experiments, where accurate determination using tools like titration is essential for safety and precision. Linus Pauling, a pioneer in understanding chemical bonding and molecular structure, significantly contributed to the theoretical frameworks that underpin our understanding of acid dissociation constants, providing a basis for comprehending why certain molecules, like HCN, exhibit their characteristic acidic strengths.
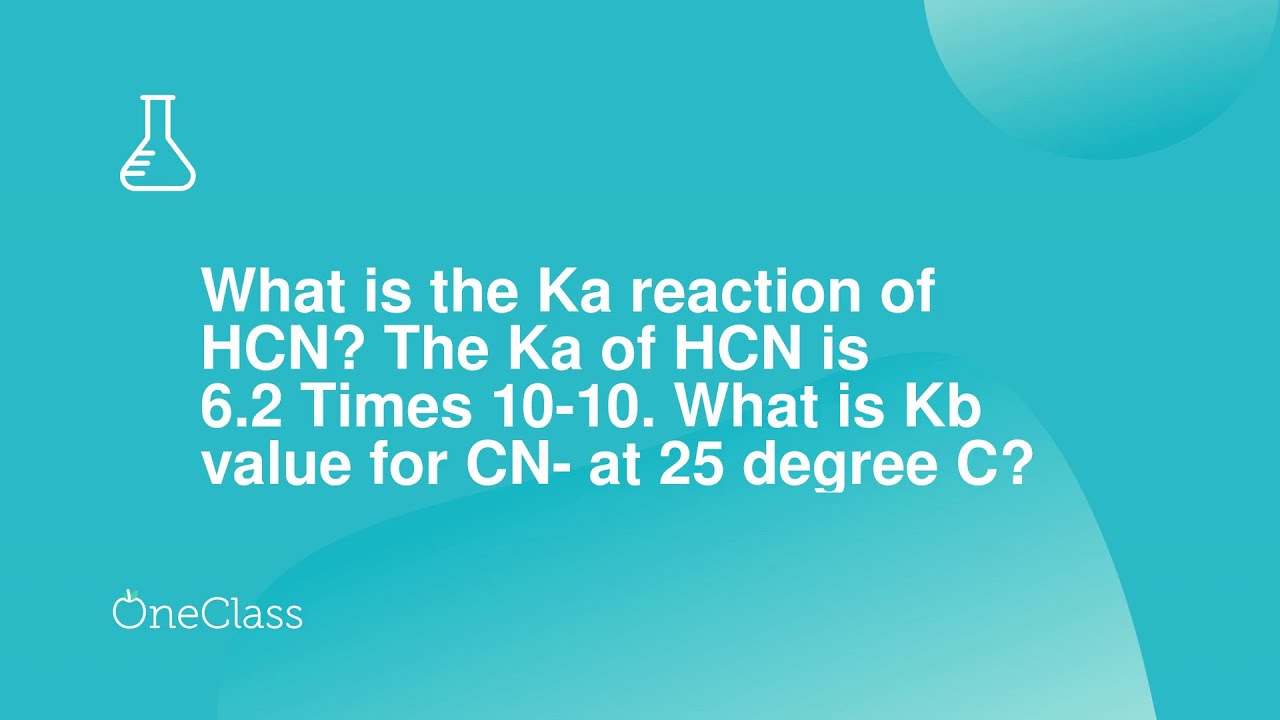
Image taken from the YouTube channel OneClass , from the video titled What is the Ka reaction of HCN? The Ka of HCN is 62 Times 10-10 What is Kb value for CN- at 25 deg .
Unveiling the Secrets of Hydrogen Cyanide's Acidity
Hydrogen Cyanide (HCN), a compound with a deceptively simple formula, holds a position of considerable importance across a diverse spectrum of scientific disciplines. From its industrial applications to its notorious toxicity, understanding its properties is paramount.
This exploration begins with a foundational understanding of HCN itself, followed by a necessary clarification of acidity and the pivotal role of the Acid Dissociation Constant, Ka.
Hydrogen Cyanide: A Chemical Profile
Hydrogen Cyanide, also known as Hydrocyanic Acid in its aqueous form, is chemically represented as HCN. At room temperature, it presents as a colorless or pale blue liquid with a characteristic bitter almond odor, although not everyone can detect this scent due to genetic variations.
Its significance stems from its utility as a precursor in the production of various chemical compounds, including polymers and fumigants. However, its high toxicity necessitates stringent safety protocols in its handling and use.
HCN's impact extends into the realms of toxicology and environmental science, primarily due to the acute toxicity associated with cyanide exposure. Even low concentrations can have devastating effects on biological systems.
Acidity Defined: The Quantification of Proton Donation
Acidity, in its most fundamental sense, describes the propensity of a chemical species to donate protons (H+). This ability to release hydrogen ions is a key determinant of a compound’s behavior in aqueous solutions.
The Acid Dissociation Constant, Ka, provides a quantitative measure of an acid's strength. Ka is defined as the equilibrium constant for the dissociation of an acid in water.
It represents the ratio of the concentrations of the products (hydrogen ions and the conjugate base) to the concentration of the undissociated acid at equilibrium.
A higher Ka value indicates a stronger acid, signifying a greater extent of dissociation in solution. Conversely, a lower Ka signifies a weaker acid, implying a lesser degree of proton donation.
Ka is, therefore, a crucial parameter for characterizing the acidic properties of a given compound.
Thesis Statement: Deciphering HCN's Acidity
The core objective of this examination is to provide a comprehensive analysis of the Acid Dissociation Constant (Ka) of Hydrogen Cyanide (HCN).
This analysis will encompass an in-depth exploration of its significance, the various factors that influence its value, the experimental methodologies employed for its determination, and its overarching relevance across multiple scientific and technological domains. By elucidating these aspects, we aim to provide a thorough understanding of the chemical behavior of HCN.
Fundamentals of Acid-Base Chemistry: Setting the Stage
Understanding the acid dissociation constant (Ka) of hydrogen cyanide (HCN) requires a firm grasp of fundamental acid-base chemistry principles. We will explore the dynamic interplay of acid dissociation, equilibrium, and the characteristics that define HCN as a weak acid. This will lead us to a discussion of the pKa value and its significance in quantifying acidity.
Acid Dissociation: HCN in Action
Acid dissociation is the cornerstone of understanding acidity. In aqueous solutions, Hydrogen Cyanide (HCN) undergoes dissociation, releasing a proton (H+) and forming a cyanide ion (CN-).
This process is represented by the following equilibrium reaction:
HCN ⇌ H+ + CN-
This seemingly simple equation encapsulates the essence of HCN's acidic behavior, illustrating its ability to donate a proton and contribute to the acidity of the solution.
Equilibrium and the Equilibrium Constant: Ka's Foundation
The Dynamic Nature of Equilibrium
Chemical equilibrium is not a static state. Rather, it is a dynamic condition where the rates of the forward and reverse reactions are equal. The forward reaction represents the dissociation of HCN, while the reverse reaction represents the association of H+ and CN- to reform HCN.
At equilibrium, the concentrations of HCN, H+, and CN- remain constant, although the reactions continue to occur.
Ka: Quantifying Equilibrium
The acid dissociation constant, Ka, is a specific type of equilibrium constant that provides a quantitative measure of the extent to which an acid dissociates in solution.
It is defined as the ratio of the concentrations of the products (H+ and CN-) to the concentration of the reactant (HCN) at equilibrium:
Ka = [H+][CN-]/[HCN]
A larger Ka value indicates a stronger acid, signifying a greater degree of dissociation.
HCN: A Weak Acid Characterization
Unlike strong acids, which dissociate completely in solution, HCN is classified as a weak acid. This means that it only partially dissociates, resulting in a lower concentration of H+ ions compared to a strong acid of the same concentration.
For example, hydrochloric acid (HCl) and sulfuric acid (H2SO4) are strong acids that dissociate almost entirely.
HCN, however, exists primarily in its undissociated form in solution, reflecting its weak acid nature.
pKa: A Convenient Scale for Acidity
Defining pKa
To facilitate easier comparison of acid strengths, the pKa value is used. The pKa is defined as the negative base-10 logarithm of the Ka:
pKa = -log10(Ka)
Significance of pKa
The pKa provides a convenient scale for expressing acidity. A lower pKa value indicates a stronger acid, which is inversely related to the Ka.
This logarithmic scale allows for easier comparison of the acidities of different compounds, making it an indispensable tool in acid-base chemistry.
By understanding these fundamental principles, we can better appreciate the significance of the Ka value of HCN and its implications in various chemical and biological systems.
Experimental Determination of Ka for HCN: Methods and Techniques
[Fundamentals of Acid-Base Chemistry: Setting the Stage Understanding the acid dissociation constant (Ka) of hydrogen cyanide (HCN) requires a firm grasp of fundamental acid-base chemistry principles. We will explore the dynamic interplay of acid dissociation, equilibrium, and the characteristics that define HCN as a weak acid. This will lead us to...] an exploration of the experimental methodologies employed to ascertain the Ka value of HCN, with a focus on titration and pH measurement. These techniques offer invaluable insights into how we can translate experimental observations into a quantifiable measure of acid strength.
Titration: Unveiling Ka Through Controlled Neutralization
Acid-base titration stands as a cornerstone analytical technique in chemistry, allowing for the precise determination of an acid's concentration. The method involves the gradual addition of a base of known concentration (the titrant) to an acid solution until the reaction reaches its equivalence point.
This point signifies that the acid has been completely neutralized by the base. Monitoring the pH throughout the titration process is critical.
For HCN, titrating with a strong base like NaOH enables us to track the formation of cyanide ions (CN-) as the acid is neutralized. The data obtained during titration provide the necessary information to calculate the Ka value.
Deriving Ka from Titration Curves
The titration curve, a plot of pH versus the volume of titrant added, provides valuable information for Ka determination. At the half-equivalence point, where exactly half of the HCN has been neutralized, the concentration of HCN equals the concentration of CN-.
Crucially, at this point, the pH of the solution is equal to the pKa of HCN. This stems from the Henderson-Hasselbalch equation:
pH = pKa + log([CN-]/[HCN])
Since [CN-] = [HCN] at the half-equivalence point, the log term becomes zero, leaving pH = pKa. By identifying the half-equivalence point on the titration curve, one can directly read the pKa value and subsequently calculate Ka (Ka = 10^-pKa).
Practical Considerations in Titration
Several factors can influence the accuracy of Ka determination via titration. Temperature control is essential, as temperature variations can shift the equilibrium and alter the Ka value.
Additionally, the ionic strength of the solution should be carefully considered, as it can affect the activity coefficients of the ions involved. Precise measurement of the titrant volume and accurate pH readings are paramount.
pH Measurement: A Direct Probe into Acidity
Measuring the pH of an HCN solution offers a direct approach to determining its Ka value. Since HCN is a weak acid, it only partially dissociates in water, establishing an equilibrium between HCN, H+, and CN-.
By carefully measuring the pH of a solution with a known concentration of HCN, one can calculate the concentrations of H+ and CN- at equilibrium.
Utilizing the Equilibrium Expression
The acid dissociation constant (Ka) is defined by the following equilibrium expression:
Ka = [H+][CN-] / [HCN]
By measuring the pH, we directly determine the [H+]. Since each HCN molecule that dissociates produces one H+ and one CN-, we can assume that [H+] = [CN-] at equilibrium (assuming negligible H+ from water autoionization).
Knowing the initial concentration of HCN and the equilibrium concentration of H+, we can calculate the equilibrium concentration of HCN and, subsequently, the Ka value.
The Precision of pH Measurements
The accuracy of Ka determination via pH measurement hinges on the precision of the pH meter and the control of experimental conditions. The pH meter must be properly calibrated using standard buffer solutions to ensure reliable readings.
Temperature control is also crucial, as temperature affects both the pH meter's performance and the equilibrium of the acid dissociation. The purity of the water used to prepare the HCN solution is important to prevent contamination that might affect the pH.
Factors Influencing the Ka Value of HCN: A Dynamic Equilibrium
Experimental determination provides a snapshot of the Ka value for HCN under specific conditions. However, this value isn't static. Several factors can dynamically influence the equilibrium, and consequently, the measured Ka. Understanding these influences is critical for accurately predicting HCN behavior across various chemical environments.
Temperature Effects: Shifting the Equilibrium
Temperature plays a pivotal role in determining the equilibrium constant, including Ka. As temperature increases, the equilibrium will shift to favor the endothermic reaction, according to Le Chatelier's principle. In the case of acid dissociation, the dissociation of HCN is typically endothermic.
This means that increasing the temperature will generally lead to a higher degree of dissociation and a larger Ka value.
The Van't Hoff Equation
The quantitative relationship between temperature and the equilibrium constant is described by the Van't Hoff equation:
d(lnK)/dT = ΔH°/RT²
Where:
- K is the equilibrium constant (Ka in this case).
- ΔH° is the standard enthalpy change of the reaction.
- R is the ideal gas constant.
- T is the absolute temperature.
This equation indicates that a positive ΔH° (endothermic reaction) will result in an increase in Ka as temperature increases. It allows for the prediction of Ka at different temperatures, given the enthalpy change of the dissociation reaction. It highlights that temperature control is crucial for accurate and reproducible Ka measurements.
Ionic Strength: The Impact of Ions in Solution
The presence of other ions in the solution, quantified as ionic strength, significantly impacts the activity of the reacting species (HCN, H+, and CN-). Ionic strength affects the activity coefficients of these ions, which in turn alters the effective concentrations influencing the Ka value.
Higher ionic strength generally leads to a decrease in the activity coefficients.
This effectively stabilizes the ionic species (H+ and CN-) relative to the neutral HCN, promoting dissociation and increasing the apparent Ka value.
Debye-Hückel Theory
The Debye-Hückel theory provides a framework for estimating activity coefficients based on ionic strength. This theory helps to predict how the Ka value of HCN will change in solutions with varying concentrations of other ions.
While the Debye-Hückel theory has limitations, particularly at high ionic strengths, it offers a valuable approximation for understanding the behavior of weak acids like HCN in complex solutions.
Ignoring ionic strength effects can lead to significant errors in Ka determination and predictions.
Solvent Effects: The Medium Matters
The solvent in which HCN is dissolved significantly affects its dissociation. Different solvents have different polarities and hydrogen-bonding capabilities, which influence the stability of the reactants (HCN) and products (H+ and CN-).
Solvent Polarity
Highly polar solvents, like water, tend to stabilize ions more effectively than nonpolar solvents. This is due to the favorable interactions between the ions and the solvent dipoles. Therefore, HCN will generally dissociate more readily in polar solvents, resulting in a higher Ka value compared to nonpolar solvents.
Hydrogen Bonding
Solvents capable of hydrogen bonding, such as water and alcohols, can also influence the dissociation of HCN. Hydrogen bonds can stabilize both HCN and its ions, but the extent of stabilization may differ. For instance, water can effectively solvate the cyanide ion (CN-) through hydrogen bonding, further promoting the dissociation of HCN.
The dielectric constant of the solvent is another important parameter. Solvents with higher dielectric constants reduce the electrostatic attraction between H+ and CN-, favoring dissociation.
The choice of solvent is not merely a matter of convenience. It is a critical parameter that directly influences the acid-base properties of HCN and must be carefully considered in experimental design and theoretical calculations.
Implications and Applications: HCN's Role in Science and Beyond
Experimental determination provides a snapshot of the Ka value for HCN under specific conditions. However, this value isn't static. Several factors can dynamically influence the equilibrium, and consequently, the measured Ka. Understanding these influences is critical for accurately predicting HCN's behavior across various scientific applications. This understanding unlocks insights into pH calculations, buffer systems, and the environmental and toxicological impact of this compound.
Ka in pH Calculations: Predicting HCN Behavior
The Ka value of HCN is not merely an academic curiosity. It serves as a critical tool for predicting the behavior of HCN in aqueous solutions.
By understanding its dissociation constant, scientists can accurately calculate the pH of solutions containing HCN, a capability that has far-reaching implications.
Utilizing Ka to Determine pH
The calculation of pH in HCN solutions involves considering the equilibrium between HCN and its dissociated ions, H+ and CN-. The Ka expression allows for the determination of the hydrogen ion concentration ([H+]) at equilibrium.
This concentration is directly related to pH through the formula: pH = -log[H+]. Accurate determination of pH is particularly important in scenarios where precise control of acidity is required.
Relevance in Biological and Environmental Systems
The ability to predict pH is crucial in biological systems, where enzymatic reactions and protein stability are highly pH-dependent. Consider how this precision applies to enzyme kinetics and protein folding, both of which are critically sensitive to pH levels.
Similarly, in environmental science, understanding the pH of HCN-contaminated water sources is essential for assessing potential risks to aquatic life and devising remediation strategies. Accurate pH determination also aids in the study of acid rain and its impact on ecosystems.
HCN as a Buffer Component: Maintaining pH Stability
Beyond its acidic properties, HCN exhibits buffering capabilities when paired with its conjugate base, cyanide (CN-). This combination forms a buffer solution that can resist drastic changes in pH upon the addition of acids or bases.
The Buffering Mechanism
A buffer solution works by neutralizing small amounts of added acid or base, thereby maintaining a relatively stable pH.
In the case of the HCN/CN- buffer, the HCN component neutralizes added base (OH-) by reacting to form water and CN-. Conversely, the CN- component neutralizes added acid (H+) by forming HCN. This dual-action ensures pH stability.
Importance of Buffer Capacity
The buffer capacity refers to the amount of acid or base that a buffer solution can neutralize before its pH changes significantly. The effectiveness of the HCN/CN- buffer depends on the concentrations of both HCN and CN-.
Higher concentrations provide a greater buffer capacity, allowing the solution to resist larger pH changes. This buffering capacity is crucial in industrial processes. Specifically, it is a critical aspect of chemical synthesis. Further, it can stabilize solutions in laboratory settings.
Environmental Science and Toxicology: Understanding HCN's Impact
HCN and cyanide compounds are significant environmental pollutants and potent toxins. Understanding the Ka of HCN provides crucial insights into its behavior and impact on living organisms.
HCN and Cyanide as Environmental Pollutants
Cyanide compounds can enter the environment through various industrial processes, including mining, electroplating, and chemical manufacturing.
The presence of HCN in water sources can pose a significant threat to aquatic life, as even low concentrations can be toxic to fish and other organisms.
The persistence and transport of cyanide compounds in the environment are influenced by factors such as pH, temperature, and the presence of other pollutants.
Toxicity Mechanisms
The toxicity of HCN stems from its ability to inhibit cellular respiration.
It binds to cytochrome c oxidase, an enzyme essential for the electron transport chain in mitochondria. This binding prevents cells from utilizing oxygen, leading to cellular asphyxiation and ultimately death.
Understanding the dose-response relationship of HCN is crucial in assessing the risks associated with exposure. Moreover, it can help develop effective treatment strategies for cyanide poisoning. This knowledge is important for determining the severity of exposure and developing an appropriate therapeutic strategy.
Video: Ka of HCN: Acidity, Value & Understanding
FAQs: Ka of HCN: Acidity, Value & Understanding
What does the Ka of HCN tell us about its strength as an acid?
The Ka of HCN (hydrogen cyanide) is a measure of its acid strength in solution. A smaller Ka value indicates a weaker acid. HCN is considered a weak acid because its Ka of hcn value is relatively low, meaning it doesn't readily donate protons (H+) in water.
What is the approximate value of the Ka of HCN, and what does it imply?
The Ka of HCN is approximately 4.9 x 10^-10. This very small value signifies that HCN only partially dissociates in water, meaning only a small fraction of HCN molecules release a proton. Consequently, it behaves as a weak acid in aqueous solutions.
How does the Ka of HCN relate to the pH of a cyanide solution?
The Ka of HCN helps determine the concentration of hydrogen ions (H+) in a solution containing cyanide ions (CN-). Since HCN is the conjugate acid of CN-, knowing the Ka of HCN, along with the concentration of cyanide, allows us to calculate the pH of the solution using equilibrium expressions.
Is HCN a strong acid based on its Ka value?
No, HCN is not a strong acid. Strong acids completely dissociate in water, which would result in a much larger Ka value. Because the Ka of hcn is very small, it indicates that HCN remains mostly undissociated in solution, characteristic of a weak acid.
So, next time you're thinking about acids and bases, remember that even seemingly simple molecules like HCN have a story to tell. Understanding the Ka of HCN, even though it's a relatively small number, can really unlock a deeper appreciation for chemical equilibrium and the subtle dance of protons in solution. Keep exploring!