HI Intermolecular Bonds: A Comprehensive Guide
Hydrogen iodide (HI), a diatomic molecule, exhibits intermolecular forces influenced by its molecular properties, a subject of considerable interest in physical chemistry and spectroscopy. The strength of intermolecular bonds on hydrogen iodide directly affects its physical state and reactivity. Scientists often utilize techniques like infrared spectroscopy, typically performed in laboratory settings, to probe these interactions and determine the vibrational frequencies associated with HI clusters. Linus Pauling's work on electronegativity provides a theoretical framework for understanding the polar nature of the H-I bond, which contributes significantly to the dipole-dipole interactions and, consequently, the overall intermolecular forces observed in hydrogen iodide.
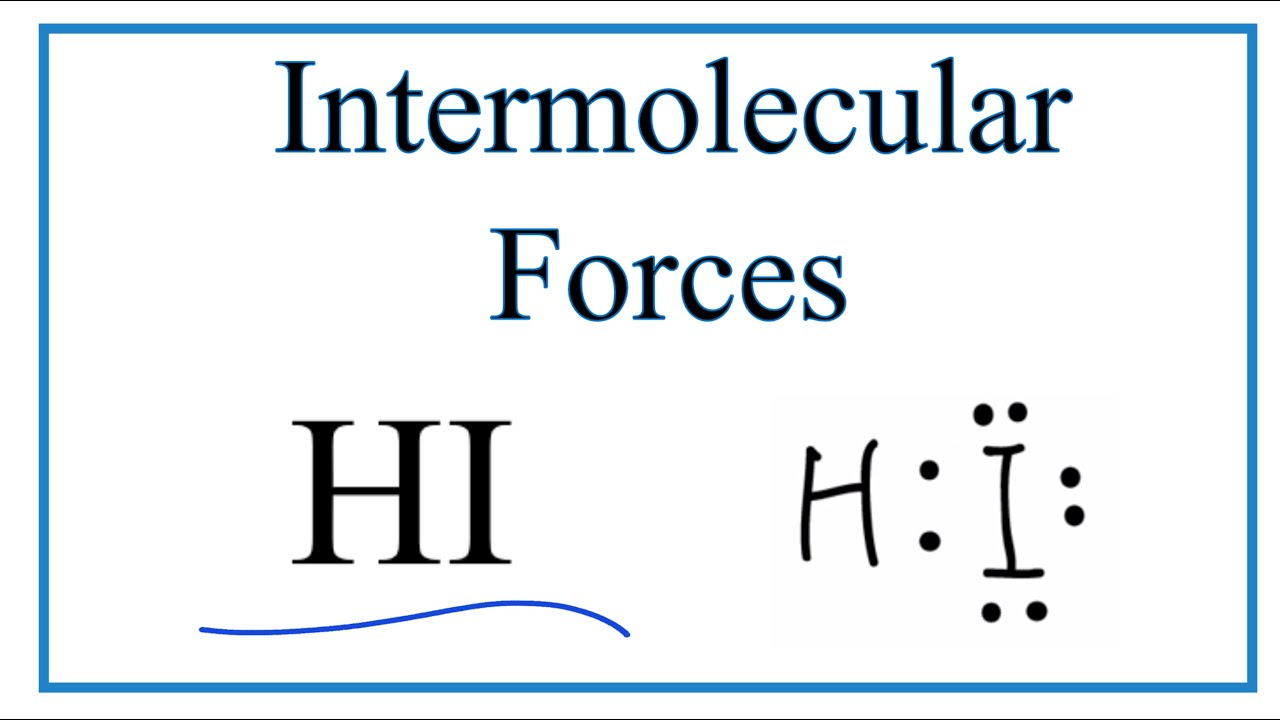
Image taken from the YouTube channel Wayne Breslyn (Dr. B.) , from the video titled Intermolecular Forces for HI (Hydrogen iodide) .
Unveiling the Secrets of Hydrogen Iodide Through Intermolecular Forces
The macroscopic properties of matter, from boiling points to solubility, are not merely intrinsic characteristics of individual molecules. They are, in fact, a direct manifestation of the intermolecular forces (IMFs) acting between them. These forces, though weaker than the intramolecular bonds holding atoms together within a molecule, dictate how molecules interact, aggregate, and ultimately behave in bulk.
Consider hydrogen iodide (HI), a seemingly simple diatomic molecule, as a compelling case study. HI's physical properties, such as its moderate boiling point and solubility characteristics, are not readily apparent from its molecular formula alone.
Instead, a deeper understanding of the IMFs at play is crucial to unraveling the underlying reasons for its observed behavior.
Defining Intermolecular Forces: The Glue That Holds Matter Together
Intermolecular forces (IMFs) are attractive or repulsive forces that exist between molecules. These forces are responsible for holding molecules together in the liquid and solid phases. Without IMFs, all substances would exist as gases, regardless of temperature.
The strength of these forces dictates the energy required to separate molecules, directly influencing properties like boiling point and melting point. Stronger IMFs translate to higher boiling and melting points, as more energy is needed to overcome the attractive forces holding the molecules together.
Hydrogen Iodide: A Case Study in Intermolecular Interactions
Hydrogen iodide (HI) serves as an excellent example to illustrate the connection between IMFs and macroscopic properties. At room temperature, HI is a colorless gas, but it can be readily condensed into a liquid at lower temperatures.
Its behavior in different solvents also provides clues about the nature of its intermolecular interactions. By examining HI, we can gain insights into how molecular structure and polarity influence the overall strength and type of IMFs present.
Key Physical Properties of Hydrogen Iodide
Before delving deeper into the specific IMFs in HI, it is useful to outline its key physical properties:
- Boiling Point: HI has a moderate boiling point (-35 °C), suggesting the presence of reasonably strong IMFs.
- Melting Point: Similarly, its melting point (-51 °C) indicates intermolecular attractions are significant but not exceptionally strong.
- Solubility: HI is soluble in water, forming hydroiodic acid, a strong acid. It also exhibits some solubility in nonpolar solvents, indicating the presence of both polar and nonpolar interactions.
The Primary Intermolecular Forces in HI: Dipole-Dipole and London Dispersion Forces
The intermolecular forces present in HI are primarily dipole-dipole interactions and London Dispersion Forces (LDF). The significant electronegativity difference between hydrogen and iodine atoms creates a polar molecule with a partial positive charge (δ+) on the hydrogen and a partial negative charge (δ-) on the iodine.
This polarity leads to attractive forces between the positive end of one HI molecule and the negative end of another, resulting in dipole-dipole interactions.
While dipole-dipole interactions are crucial, London Dispersion Forces (LDF), present in all molecules, also contribute to the overall intermolecular attraction in HI. LDF arise from temporary fluctuations in electron distribution, creating temporary dipoles that induce dipoles in neighboring molecules.
The relative contributions of these two forces and how they influence HI's properties will be explored in greater detail in the subsequent sections.
Foundation: Understanding Polarity and Intermolecular Forces
The macroscopic properties of matter, from boiling points to solubility, are not merely intrinsic characteristics of individual molecules. They are, in fact, a direct manifestation of the intermolecular forces (IMFs) acting between them. These forces, though weaker than the intramolecular bonds holding atoms together within a molecule, dictate how molecules interact with their neighbors. Understanding the nature and strength of these forces is crucial for predicting and explaining the behavior of substances like hydrogen iodide (HI).
Polarity: The Uneven Distribution of Electrons
The foundation of many intermolecular forces lies in the concept of polarity. Polarity arises from differences in electronegativity between atoms within a molecule. Electronegativity is a measure of an atom's ability to attract electrons in a chemical bond.
When two atoms with significantly different electronegativities bond, the electrons are not shared equally. The more electronegative atom pulls the electron density closer, resulting in a partial negative charge (δ-) on that atom and a partial positive charge (δ+) on the less electronegative atom. This uneven distribution of charge creates a polar bond.
Quantifying Polarity: The Dipole Moment
The magnitude of polarity is quantified by the dipole moment (µ), a vector quantity that points from the positive to the negative end of the polar bond. The dipole moment is calculated as the product of the magnitude of the partial charge (δ) and the distance (d) between the charges: µ = δd. The unit of dipole moment is typically the Debye (D).
For a molecule with multiple polar bonds, the overall molecular dipole moment is the vector sum of the individual bond dipole moments. Molecular geometry plays a critical role here. Even if a molecule contains polar bonds, if the geometry is symmetrical such that the bond dipoles cancel each other out, the molecule will be nonpolar overall (e.g., carbon dioxide).
Classifying Intermolecular Forces
Intermolecular forces are broadly classified as Van der Waals forces, a collective term that encompasses dipole-dipole interactions, London Dispersion Forces (LDF), and, sometimes, hydrogen bonding (though hydrogen bonding is significantly stronger than typical Van der Waals forces).
London Dispersion Forces: The Ubiquitous Attraction
London Dispersion Forces (LDF), also known as induced dipole-induced dipole interactions, are present in all molecules, regardless of their polarity. They arise from temporary, instantaneous fluctuations in electron distribution.
These fluctuations create temporary dipoles, which can then induce dipoles in neighboring molecules, leading to a weak attractive force. The strength of LDF depends on the polarizability of the molecule, which is its ability to form these temporary dipoles.
Polarizability generally increases with molecular size (number of electrons) and molecular shape (more elongated molecules are more polarizable than compact ones). Therefore, larger molecules with more electrons tend to exhibit stronger LDF.
The Absence of Hydrogen Bonding in HI
Hydrogen bonding is a particularly strong type of dipole-dipole interaction that occurs when a hydrogen atom is bonded to a highly electronegative atom (nitrogen, oxygen, or fluorine) and interacts with another electronegative atom on a neighboring molecule. This interaction is much stronger than typical dipole-dipole forces.
However, hydrogen bonding does not occur in hydrogen iodide (HI). This is because iodine is not electronegative enough to create a sufficiently strong partial positive charge on the hydrogen atom. The hydrogen atom in HI does not possess the high degree of positive charge density necessary to form a strong hydrogen bond with another electronegative atom.
Implications for HI's Physical Properties
The absence of hydrogen bonding in HI has significant implications for its physical properties. Compared to molecules of similar molecular weight that can form hydrogen bonds (like water or alcohols), HI exhibits a significantly lower boiling point and melting point.
The lack of this strong intermolecular attraction means that less energy is required to overcome the forces holding HI molecules together in the liquid or solid phase, resulting in lower phase transition temperatures. Understanding why HI lacks hydrogen bonding is a critical step in appreciating the nuanced interplay of IMFs that govern its macroscopic behavior.
The Dominant Forces: Intermolecular Interactions in Hydrogen Iodide
The macroscopic properties of matter, from boiling points to solubility, are not merely intrinsic characteristics of individual molecules. They are, in fact, a direct manifestation of the intermolecular forces (IMFs) acting between them. These forces, though weaker than the intramolecular bonds holding atoms together within a molecule, dictate how molecules interact with each other, ultimately shaping the physical behavior of substances. In the case of hydrogen iodide (HI), understanding its dominant IMFs is crucial for explaining its observed properties.
Dipole-Dipole Interactions: The Primary Force in HI
Hydrogen iodide is a polar molecule due to the difference in electronegativity between hydrogen and iodine. Iodine is significantly more electronegative, pulling electron density towards itself and creating a partial negative charge (δ-) on the iodine atom and a partial positive charge (δ+) on the hydrogen atom.
This unequal distribution of charge results in a permanent dipole moment, which is a vector quantity representing the magnitude and direction of the charge separation.
The presence of this dipole moment leads to dipole-dipole interactions.
Dipole-dipole interactions occur when the positive end of one HI molecule is attracted to the negative end of another HI molecule.
These interactions are electrostatic in nature and contribute significantly to the overall intermolecular forces in HI. They are, therefore, the primary IMF in hydrogen iodide.
The Role of London Dispersion Forces
While dipole-dipole interactions are dominant, London Dispersion Forces (LDF) also contribute to the overall intermolecular attraction in HI. LDFs are temporary, fluctuating dipoles that arise from the instantaneous distribution of electrons within a molecule.
Even in nonpolar molecules, LDFs exist, and their strength generally increases with increasing molecular size and number of electrons.
Iodine is a large, highly polarizable atom, meaning its electron cloud is easily distorted. This increased polarizability results in stronger LDFs in HI compared to lighter hydrogen halides.
Therefore, while not the primary force, LDFs do play a non-negligible role in the intermolecular forces of HI, adding to its overall attraction between molecules.
Comparing Hydrogen Halides: Trends in Polarity and IMFs
Examining the hydrogen halides (HF, HCl, HBr, and HI) reveals important trends in polarity and intermolecular forces. As we move down the group from fluorine to iodine, the electronegativity of the halogen decreases.
This means the difference in electronegativity between hydrogen and the halogen decreases, leading to a reduction in the dipole moment of the molecule.
Therefore, HF has the highest dipole moment, followed by HCl, HBr, and then HI.
However, the boiling point trend does not perfectly follow this decreasing polarity. While HF has an anomalously high boiling point due to hydrogen bonding, the boiling points of HCl, HBr, and HI increase down the group.
This is because, while the dipole-dipole interactions are decreasing, the strength of London Dispersion Forces increases significantly due to the increasing size and polarizability of the halogen atoms.
In other words, for HCl, HBr, and HI, the increasing LDFs overcome the decreasing dipole-dipole interactions, resulting in an overall increase in intermolecular attraction and, thus, boiling point.
This highlights the interplay between different types of IMFs and their influence on macroscopic properties.
Properties Unveiled: Connecting Intermolecular Forces to Hydrogen Iodide's Characteristics
The macroscopic properties of matter, from boiling points to solubility, are not merely intrinsic characteristics of individual molecules. They are, in fact, a direct manifestation of the intermolecular forces (IMFs) acting between them. These forces, though weaker than the intramolecular bonds holding atoms together within a molecule, dictate how molecules interact with each other. In the case of hydrogen iodide (HI), understanding the interplay of dipole-dipole interactions and London Dispersion Forces (LDF) is crucial to explaining its observable physical properties.
Boiling Point and Melting Point
Boiling point and melting point serve as telltale signs of the magnitude of intermolecular forces within a substance. The stronger the intermolecular attractions, the greater the energy required to overcome these forces and transition from a condensed phase (solid or liquid) to a gaseous phase.
In HI, the moderate polarity of the H-I bond gives rise to dipole-dipole interactions, contributing significantly to its boiling point. Additionally, the large electron cloud surrounding the iodine atom results in substantial London Dispersion Forces. These combined effects explain why HI has a higher boiling point than hydrogen chloride (HCl), despite HCl being more polar. The more significant London Dispersion forces present in HI owing to its larger size increase the overall intermolecular attraction, which subsequently increases the energy required to break the attraction.
The influence of IMFs on melting point is more complex. While stronger IMFs generally lead to higher melting points, molecular shape and packing efficiency in the solid state also play critical roles. HI's melting point reflects the energy needed to disrupt its crystal lattice, which is influenced by both dipole-dipole interactions and LDF.
Solubility
Solubility, the ability of a substance (solute) to dissolve in a solvent, is governed by the principle of "like dissolves like." This means that polar solutes tend to dissolve in polar solvents, while nonpolar solutes dissolve in nonpolar solvents.
Solubility in Water
HI is readily soluble in water (H₂O) due to water's highly polar nature. The strong dipole-dipole interactions between HI molecules and water molecules facilitate the dissolution process.
Furthermore, HI is a strong acid, and when dissolved in water, it undergoes ionization to form hydronium ions (H₃O⁺) and iodide ions (I⁻). This ionization process further enhances its solubility in water.
Solubility in Nonpolar Solvents
In contrast, HI exhibits limited solubility in nonpolar solvents like hexane or toluene. These solvents lack the strong dipoles necessary to effectively interact with HI molecules.
While LDFs can facilitate some degree of dissolution, the relatively strong dipole-dipole interactions within HI favor its association with other polar molecules, making it less miscible with nonpolar solvents.
Phase Transitions
Phase transitions—such as melting, boiling, sublimation, and deposition—represent the physical changes of matter between solid, liquid, and gaseous states. These transitions are fundamentally controlled by the interplay between thermal energy, which encourages molecular motion and separation, and intermolecular forces, which promote molecular attraction and cohesion.
For HI, the energy required to overcome these IMFs and transition between phases is directly related to the strength of its dipole-dipole interactions and LDF.
At low temperatures, HI exists as a solid, where intermolecular forces dominate, holding the molecules in a fixed lattice structure. As temperature increases, thermal energy begins to counteract these forces.
At the melting point, the solid transitions to a liquid as molecules gain sufficient kinetic energy to overcome the lattice structure but remain in close proximity due to IMFs. Finally, at the boiling point, the liquid transitions to a gas, with molecules possessing enough energy to completely overcome intermolecular attractions and move freely.
Investigating the Invisible: Experimental and Computational Approaches
The influence of intermolecular forces, while fundamentally critical to understanding the behavior of hydrogen iodide, operates at a scale that is beyond direct observation. Thus, scientists rely on sophisticated experimental and computational methods to probe these elusive interactions. These approaches provide complementary insights, allowing for a comprehensive characterization of the forces governing HI's properties.
Spectroscopic Characterization
Spectroscopy serves as a powerful experimental window into the molecular world. By analyzing the interaction of electromagnetic radiation with a substance, it is possible to glean information about its structure, dynamics, and, crucially, the nature of its intermolecular interactions. Techniques such as Infrared (IR) and Microwave spectroscopy are particularly valuable in the study of HI.
IR spectroscopy, for instance, probes the vibrational modes of molecules. The frequencies at which a molecule absorbs infrared radiation are sensitive to the strength and nature of the chemical bonds present, as well as to the intermolecular environment. Shifts and broadenings in the IR spectrum of HI in different phases or solutions can therefore provide direct evidence of the presence and strength of intermolecular forces.
Microwave spectroscopy, on the other hand, examines the rotational transitions of molecules. These transitions are highly dependent on the molecular geometry and dipole moment, thus yielding valuable information regarding the polarity and shape of HI molecules.
Unveiling Molecular Secrets Through Spectral Analysis
By carefully analyzing the spectral data obtained from IR and microwave experiments, researchers can:
-
Quantify the strength of dipole-dipole interactions between HI molecules.
-
Assess the contribution of London Dispersion Forces (LDF) by examining the broadening of spectral lines.
-
Determine the extent to which HI molecules are influenced by their surrounding environment.
In short, spectroscopic characterization provides empirical evidence of the IMFs governing HI's behavior, thereby bridging the gap between theoretical understanding and experimental observation.
Computational Studies: Modeling Intermolecular Interactions
While experimental techniques offer direct insights, computational methods provide a complementary approach. Computational chemistry allows scientists to create theoretical models of HI molecules and their interactions, enabling the prediction of properties and behaviors that may be difficult or impossible to measure experimentally.
These methods typically involve solving the Schrödinger equation for the system of interest, using approximations such as Density Functional Theory (DFT) or Hartree-Fock theory. These calculations can provide detailed information about the electronic structure of HI, including its dipole moment, polarizability, and the energies of its various electronic states.
More sophisticated simulations, such as Molecular Dynamics (MD) simulations, can be used to study the behavior of HI molecules over time. These simulations involve numerically solving the equations of motion for a collection of molecules, allowing researchers to observe how they interact with each other under different conditions.
Synergistic Power of Simulation and Experimentation
By comparing the results of computational studies with experimental data, scientists can:
-
Validate the accuracy of their theoretical models.
-
Gain a deeper understanding of the underlying physical principles governing intermolecular interactions.
-
Predict the properties of HI under conditions that are difficult or impossible to access experimentally (e.g., at extremely high pressures or temperatures).
In essence, computational studies serve as a powerful tool for complementing and extending experimental findings, facilitating a more comprehensive and nuanced understanding of the IMFs that shape the behavior of hydrogen iodide. The synergy between experimental observation and computational modeling is essential for advancing our knowledge of the molecular world.
Video: HI Intermolecular Bonds: A Comprehensive Guide
FAQs: HI Intermolecular Bonds
What type of intermolecular forces are most significant in liquid HI?
Dipole-dipole interactions are the most significant intermolecular forces on hydrogen iodide. While London dispersion forces are always present, the relatively high electronegativity difference between hydrogen and iodine creates a substantial dipole moment. This results in stronger dipole-dipole attractions compared to dispersion forces alone.
Why doesn't HI form strong hydrogen bonds like HF, even though it contains hydrogen?
Hydrogen bonding requires a very electronegative atom (fluorine, oxygen, or nitrogen) directly bonded to hydrogen. Iodine, although electronegative, is not electronegative enough to create the strong, polarized bond needed for significant hydrogen bonding. Therefore, intermolecular bonds on hydrogen iodide are primarily dipole-dipole.
How do the intermolecular forces in HI affect its boiling point compared to other hydrogen halides?
HI has a higher boiling point than HCl and HBr primarily due to stronger dipole-dipole interactions and larger London dispersion forces. The larger size of the iodine atom contributes to stronger dispersion forces, while the significant dipole moment due to electronegativity differences enhances dipole-dipole interactions. This combination elevates the energy needed to overcome intermolecular bonds on hydrogen iodide during boiling.
How do intermolecular forces of hydrogen iodide influence its solubility in different solvents?
HI is more soluble in polar solvents due to favorable dipole-dipole interactions between HI and the solvent molecules. Nonpolar solvents interact primarily through weaker London dispersion forces, leading to lower solubility. Stronger intermolecular bonds on hydrogen iodide will need stronger interactions to be broken by the solvent.
So, next time you're thinking about interactions at the molecular level, don't forget the crucial role of HI intermolecular bonds. They might seem complex at first, but understanding them opens up a whole new world of chemical behavior. Hopefully, this guide has given you a solid foundation – now go forth and explore the fascinating world of intermolecular forces!