Histidine Titration Curve: A Student's Guide
Histidine, an amino acid crucial for biological processes, exhibits acid-base properties that can be explored through its histidine titration curve. The histidine titration curve graphically represents the protonation states of histidine at different pH levels, a concept extensively utilized in biochemistry and molecular biology courses. Understanding this curve is essential for students studying protein chemistry, where histidine's imidazole side chain, characterized by its pKa near physiological pH, often plays a key role in enzymatic catalysis. Employing tools like the Henderson-Hasselbalch equation allows for the calculation of the ratios of protonated and deprotonated forms of histidine at various pH values, thereby facilitating a deeper understanding of the histidine titration curve and its implications.
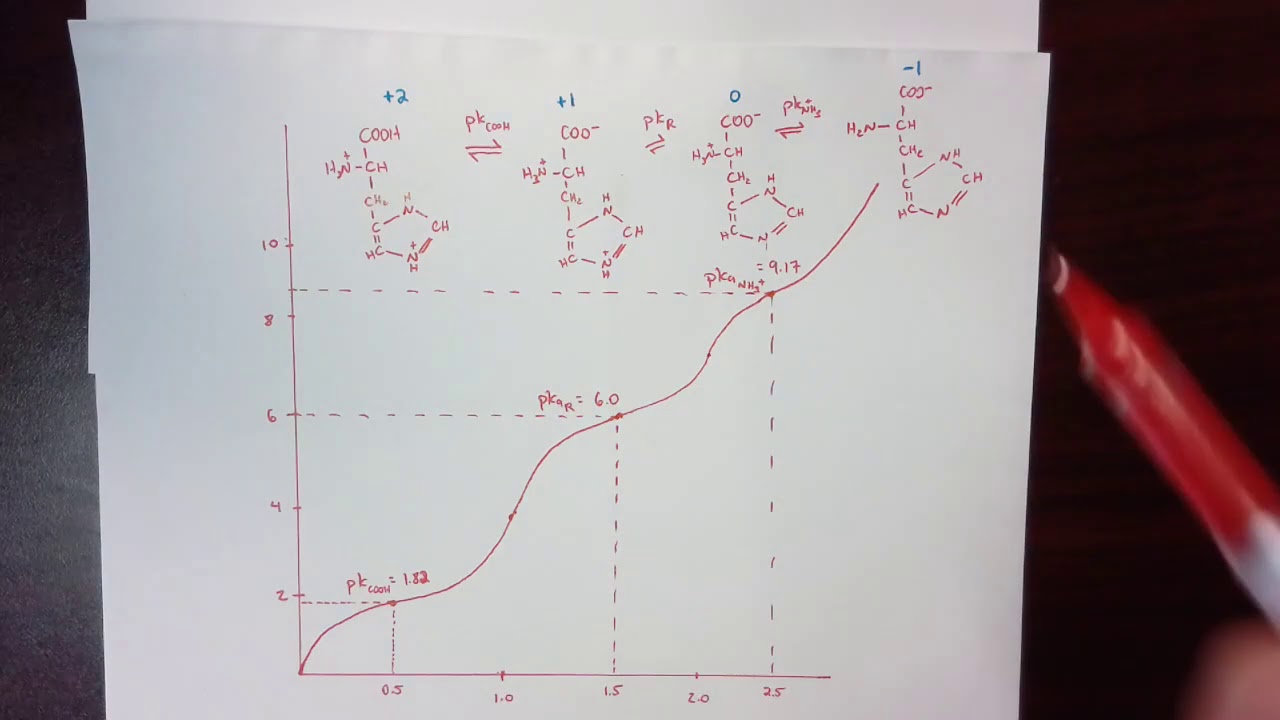
Image taken from the YouTube channel B Ferrell , from the video titled Detailed explanation of titration curve of histidine .
Unveiling Histidine Titration Curves: A Deep Dive
Titration, a cornerstone of analytical chemistry, serves as a precise quantitative method for determining the concentration of a substance. It involves the gradual addition of a titrant—a solution of known concentration—to the analyte, the substance being analyzed.
The reaction between the titrant and analyte proceeds until it reaches the equivalence point, which can be identified through various methods, including the use of indicators or electrochemical measurements. This process allows us to accurately quantify the amount of analyte present in the sample.
The Significance of Titration Curves
Titration curves are graphical representations that plot the change in pH (or another relevant property) of a solution against the volume of titrant added. These curves provide valuable insights into the chemical properties of the analyte, including its acidity, basicity, and buffering capacity.
The applications of titration curves span across numerous scientific disciplines. In environmental science, they're used to assess water quality and monitor pollution levels.
In the pharmaceutical industry, they play a crucial role in drug development and quality control. Furthermore, titration curves are indispensable in biochemistry for studying the behavior of proteins, amino acids, and other biomolecules.
Histidine: A Unique Amino Acid
Among the twenty standard amino acids, histidine stands out due to its unique structure and functional properties. Its most distinguishing feature is the imidazole ring in its side chain.
This aromatic heterocycle contains two nitrogen atoms, one of which can be protonated under physiological conditions. This characteristic gives histidine a pKa close to physiological pH, making it a crucial player in many biological processes.
The imidazole ring allows histidine to act as both a proton donor and acceptor, enabling it to play a significant role in enzyme catalysis and buffering within biological systems. Its presence in the active sites of many enzymes underscores its importance in biochemical reactions.
Understanding histidine's behavior through titration curves is essential for comprehending its role in protein structure, function, and biological regulation. The titration curve reveals key information about its protonation states and buffering capacity.
Foundational Concepts: pH, pKa, and Protonation States
Before embarking on the specifics of histidine titration, it is crucial to solidify our understanding of the underlying chemical principles that govern its behavior. These concepts include pH, pKa, protonation states, and the indispensable Henderson-Hasselbalch equation. A firm grasp of these fundamentals is essential for accurately interpreting the titration curve and understanding histidine's role in biological systems.
Understanding pH: A Measure of Acidity
pH, a logarithmic scale, quantifies the acidity or alkalinity of a solution. It represents the negative logarithm (base 10) of the hydrogen ion concentration ([H+]).
-
A pH value of 7 indicates neutrality.
-
Values below 7 signify acidity, with lower values indicating stronger acids.
-
Values above 7 signify alkalinity (or basicity), with higher values denoting stronger bases.
Understanding pH is fundamental because the protonation state of histidine, and thus its charge, is highly dependent on the surrounding pH.
pKa: Quantifying Acid Strength
pKa is a critical concept closely related to pH. It represents the acid dissociation constant, Ka, expressed on a logarithmic scale (pKa = -log10Ka).
It essentially tells us the propensity of a molecule to donate a proton at a particular pH.
The lower the pKa value, the stronger the acid, meaning it is more likely to donate a proton. Each ionizable group in histidine has a specific pKa value, and these values are crucial for understanding the shape of the titration curve.
Protonation and Deprotonation: The Dance of Protons
Protonation refers to the addition of a proton (H+) to a molecule, while deprotonation refers to the removal of a proton. These processes alter the charge of the molecule.
Histidine, with its multiple ionizable groups, can exist in various protonation states depending on the surrounding pH.
At low pH (acidic conditions), histidine will be fully protonated and carry a positive charge. As the pH increases, the molecule will undergo sequential deprotonation, losing protons from its different functional groups, each characterized by a distinct pKa.
The charge state of histidine significantly impacts its interactions with other molecules, especially within proteins.
The Henderson-Hasselbalch Equation: A Predictive Tool
The Henderson-Hasselbalch equation provides a quantitative relationship between pH, pKa, and the relative concentrations of an acid and its conjugate base:
pH = pKa + log10([A-]/[HA])
Where:
- [A-] is the concentration of the conjugate base.
- [HA] is the concentration of the acid.
This equation is invaluable for predicting the protonation state of histidine at a given pH. When the pH equals the pKa, the concentrations of the acid and its conjugate base are equal.
Furthermore, the Henderson-Hasselbalch equation serves as the foundation for understanding buffering capacity. Solutions are most effective as buffers at pH values close to their pKa. By understanding these core concepts, we can accurately predict histidine's behavior under varying conditions. This knowledge is essential for effectively performing and interpreting a histidine titration.
Histidine: Structure, Properties, and Buffer Action
Having established the fundamental principles of pH, pKa, and protonation states, we now turn our attention to histidine itself. Understanding the intricate relationship between histidine’s structure, properties, and buffering capabilities is key to interpreting its titration curve. This section will explore the unique structural aspects of histidine and how they dictate its behavior in solution, particularly its remarkable ability to act as a buffer.
Histidine's Unique Chemical Structure
Histidine is distinguished from other common amino acids by the presence of an imidazole ring in its side chain. The basic structure of histidine, like all amino acids, includes a central carbon atom bonded to an amino group (-NH2), a carboxyl group (-COOH), a hydrogen atom, and a distinctive R-group (the imidazole ring in this case).
The imidazole ring is a five-membered heterocyclic ring containing two nitrogen atoms. One nitrogen atom is protonated at physiological pH, bearing a positive charge, while the other remains unprotonated. This unique arrangement plays a crucial role in histidine's acid-base properties and its function in biological systems.
Zwitterion Formation and pH Dependence
In aqueous solutions, amino acids like histidine exist predominantly as zwitterions. A zwitterion is a molecule with both positive and negative electrical charges, resulting from the protonation of the amino group and deprotonation of the carboxyl group.
The formation and stability of the zwitterionic form are highly dependent on the pH of the surrounding environment. At low pH (acidic conditions), the amino group is protonated (-NH3+), and the carboxyl group remains protonated (-COOH), resulting in a net positive charge. As the pH increases, the carboxyl group loses a proton (-COO-), leading to the zwitterionic form with no net charge. Further increases in pH cause the imidazole ring and then the amino group to deprotonate, resulting in a net negative charge.
Histidine as a Biological Buffer
One of the most significant properties of histidine is its ability to act as a buffer. Buffers are solutions that resist changes in pH upon the addition of small amounts of acid or base.
Histidine's buffering capacity stems from the presence of the imidazole ring, which has a pKa value close to physiological pH (around 6.0). This means that at physiological pH, the imidazole ring can readily accept or donate protons, thereby neutralizing added acid or base and maintaining a relatively stable pH.
Defining Buffer Capacity
Buffer capacity refers to the amount of acid or base a buffer can neutralize before the pH begins to change significantly. Histidine's buffer capacity is optimal near its pKa value, where equal concentrations of the protonated and deprotonated forms of the imidazole ring are present. This allows it to effectively absorb changes in pH within a narrow range.
In biological systems, histidine residues in proteins contribute significantly to the overall buffering capacity of cells and tissues. This is especially important in maintaining optimal conditions for enzymatic reactions and other biochemical processes.
Influence of the Imidazole Ring on pKa Values
The imidazole ring of histidine has a profound effect on its pKa values. Histidine has three ionizable groups: the carboxyl group, the amino group, and the imidazole ring. Each group has a characteristic pKa value that reflects its tendency to donate or accept protons.
- The pKa of the carboxyl group is typically around 2.0.
- The pKa of the amino group is around 9.0.
- The pKa of the imidazole ring is approximately 6.0.
The proximity of the imidazole ring to the amino and carboxyl groups influences their respective pKa values. The presence of the slightly positive imidazole at physiological pH increases the acidity of the carboxyl group and decreases the acidity of the amino group. The relatively low pKa of the imidazole ring is what makes histidine a particularly effective buffer at physiological pH.
Setting Up and Performing Histidine Titration
Having established the fundamental principles of pH, pKa, and protonation states, we now turn our attention to histidine itself. Understanding the intricate relationship between histidine’s structure, properties, and buffering capabilities is key to interpreting its titration curve. This section will guide you through the practical aspects of setting up and performing a histidine titration experiment, ensuring you can gather the data necessary for meaningful analysis.
Materials Required for Histidine Titration
Before embarking on the titration, it's crucial to assemble the necessary materials. Precision and accuracy are paramount to obtaining reliable results. The essential items include:
-
pH Meter: A calibrated pH meter with a resolution of at least 0.01 pH units is essential for accurate pH measurements throughout the titration. The electrode should be properly maintained and stored according to the manufacturer's instructions.
-
Buret: A buret (typically 25 mL or 50 mL) is used to dispense the titrant accurately and precisely. Ensure the buret is clean and free of air bubbles before use.
-
Titrant: A standardized solution of a strong acid (e.g., 0.1 M HCl) or a strong base (e.g., 0.1 M NaOH) is needed as the titrant. The concentration of the titrant must be accurately known.
-
Histidine Solution: Prepare a known concentration of histidine solution. This will be the analyte being titrated.
-
Beaker or Titration Vessel: A clean beaker or titration vessel to contain the histidine solution during the titration.
-
Magnetic Stirrer and Stir Bar: A magnetic stirrer ensures thorough mixing of the solution during the titration, promoting rapid equilibrium.
-
Distilled Water: Use distilled water for preparing solutions and rinsing equipment.
Step-by-Step Titration Procedure
Executing the titration requires a methodical approach.
Attention to detail and careful recording of data are critical. Follow these steps for a successful histidine titration:
-
Preparation: Begin by accurately measuring a known volume of the histidine solution into the titration vessel. Add a stir bar and place the vessel on the magnetic stirrer.
-
Initial pH Measurement: Immerse the pH meter electrode into the histidine solution and allow the reading to stabilize. Record the initial pH value.
-
Titrant Addition: Carefully add the titrant (either acid or base, depending on the starting pH of the histidine solution) from the buret in small increments (e.g., 0.1 mL to 0.5 mL). The initial additions should be smaller (0.1 mL) for greater accuracy.
-
pH Monitoring: After each addition of titrant, allow the solution to mix thoroughly using the magnetic stirrer. Wait for the pH reading on the pH meter to stabilize before recording the pH value and the corresponding volume of titrant added.
-
Buffering Region Considerations: As you approach the expected buffering regions (around the pKa values), reduce the volume of titrant added per increment (e.g., 0.05 mL or even 0.01 mL) to capture the buffering effect with greater precision.
-
Equivalence Point Determination: Continue adding titrant and monitoring the pH until you have passed all expected equivalence points. Observe for rapid changes in pH with small additions of titrant.
-
Data Recording: Meticulously record the volume of titrant added and the corresponding pH value in a table or spreadsheet. This data will be used to generate the titration curve.
Generating the Titration Curve
The titration curve is a graphical representation of the titration data. It is generated by plotting the pH values on the y-axis against the volume of titrant added on the x-axis.
-
Plotting the Data: Use a graphing software program (e.g., Microsoft Excel, Google Sheets, or specialized scientific plotting software) to create the titration curve.
-
Curve Analysis: The resulting curve will display characteristic features, including buffering regions (plateaus) and equivalence points (steep changes in pH).
Analyzing this curve will allow determination of the pKa values for histidine’s ionizable groups, as well as its isoelectric point (pI). Accurate data is essential for a meaningful and accurate interpretation of the buffering capabilities of histidine.
Interpreting the Histidine Titration Curve
Having set up and performed the histidine titration, the next critical step is to analyze the resulting data, which is graphically represented as a titration curve. The histidine titration curve provides a wealth of information about the amino acid's behavior in solution, revealing its ionization states, buffering capacity, and isoelectric point. Decoding this curve is crucial for understanding how histidine functions in biological systems and proteins.
Identifying the Three Stages of the Titration Curve
The histidine titration curve exhibits three distinct stages, each corresponding to the deprotonation of one of its three ionizable groups: the carboxyl group (COOH), the amino group (NH3+), and the imidazole side chain. These stages appear as plateaus or buffering regions on the curve, separated by relatively sharp transitions.
-
Stage 1: Carboxyl Group Deprotonation: At low pH, all three groups are protonated. As the pH increases, the carboxyl group (COOH) loses its proton first. This corresponds to the first buffering region and equivalence point.
-
Stage 2: Imidazole Side Chain Deprotonation: Next, the imidazole side chain of histidine loses its proton. This stage is particularly important because the imidazole group's pKa is close to physiological pH, making histidine an effective buffer in biological systems.
-
Stage 3: Amino Group Deprotonation: Finally, at higher pH values, the amino group (NH3+) is deprotonated, marking the third and final stage of the titration.
Determining pKa Values from the Titration Curve
The pKa value represents the pH at which half of the molecules of a particular species are protonated, and half are deprotonated. On the titration curve, the pKa values can be determined at the midpoint of each buffering region, also known as the half-equivalence point.
At each of these midpoints, the pH is equal to the pKa of the corresponding ionizable group. Accurately identifying these points on the curve is essential for characterizing the acid-base properties of histidine.
Buffering Regions and Buffer Capacity
Buffering regions are the relatively flat portions of the titration curve where the pH changes slowly upon the addition of acid or base. These regions occur near the pKa values of each ionizable group. Histidine exhibits maximum buffering capacity at pH values close to its pKa values.
Buffer capacity refers to the ability of a solution to resist changes in pH upon the addition of an acid or a base. The wider the buffering region, the greater the buffer capacity. Histidine's imidazole side chain, with a pKa near physiological pH, makes it an important buffer in many biological systems.
Understanding Buffer Capacity
Buffer capacity is influenced by the concentration of the buffer and the proximity of the pH to the pKa.
Calculating the Isoelectric Point (pI)
The isoelectric point (pI) is the pH at which the molecule carries no net electrical charge. For histidine, which has three ionizable groups, the pI is calculated as the average of the two pKa values that surround the neutral species.
Specifically, the pI is calculated using the formula: pI = (pKa2 + pKa3)/2
where pKa2 and pKa3 represent the pKa values for the imidazole side chain and the amino group, respectively. The pI is an important characteristic of amino acids and proteins, influencing their solubility and behavior in different environments.
Histidine's Significance and Applications: From Physiology to Proteins
Having deciphered the intricacies of the histidine titration curve, it's time to explore the broader implications of histidine's unique properties in biological systems. Histidine's distinctive structure and buffering capacity render it a vital player in various physiological processes and protein functions.
Histidine as a Physiological Buffer
Histidine's imidazole side chain, with a pKa value near physiological pH, makes it an effective buffer in biological systems. This buffering capacity is crucial for maintaining pH homeostasis, which is essential for the proper functioning of cells and tissues.
Variations in pH can disrupt enzyme activity, protein folding, and other critical cellular processes. Histidine helps mitigate these fluctuations, particularly in intracellular environments and within specific organelles.
The imidazole ring can accept or donate protons, allowing histidine to act as a proton shuttle. This is particularly important in situations where rapid changes in pH occur, such as during muscle contraction or in response to metabolic changes.
Histidine in Enzyme Active Sites
Beyond its role as a general buffer, histidine frequently participates directly in enzyme catalysis. Its imidazole ring can act as both a proton donor and a proton acceptor, making it a versatile residue in enzyme active sites.
Catalytic Mechanisms
Histidine residues are often found in the active sites of enzymes where they facilitate reactions by acting as general acid-base catalysts. In these mechanisms, histidine can either donate a proton to activate a substrate or accept a proton to stabilize a transition state.
This is particularly evident in enzymes like chymotrypsin, where a histidine residue is part of the catalytic triad. The imidazole side chain facilitates the hydrolysis of peptide bonds.
Substrate Binding
In addition to its catalytic role, histidine can contribute to substrate binding through hydrogen bonding or electrostatic interactions. Its positive charge at certain pH levels can attract negatively charged substrates or stabilize interactions with other residues in the binding pocket.
The Legacy of Henderson and Hasselbalch
The understanding of histidine's buffering capacity and its role in biological systems is deeply rooted in the work of Lawrence Henderson and Karl Albert Hasselbalch. Their contributions laid the foundation for understanding acid-base equilibria.
The Henderson-Hasselbalch Equation
The Henderson-Hasselbalch equation, derived from the acid dissociation constant, provides a quantitative relationship between pH, pKa, and the ratio of protonated and deprotonated forms of a molecule.
This equation is invaluable for predicting the ionization state of histidine at a given pH. It also helps to understand its buffering behavior. This equation is essential for researchers and clinicians alike in fields ranging from biochemistry to medicine.
Implications for Biological Research
The principles established by Henderson and Hasselbalch are fundamental to understanding numerous biological processes. This ranges from enzyme kinetics to drug design. Their work allows for the prediction and manipulation of molecular interactions in biological systems, furthering our understanding of life at the molecular level.
Video: Histidine Titration Curve: A Student's Guide
FAQs: Histidine Titration Curve: A Student's Guide
What does the histidine titration curve show?
The histidine titration curve visually represents how the charge of histidine changes as you add a strong base (like NaOH). It plots pH against the equivalents of base added, illustrating the protonation and deprotonation of histidine's functional groups. This helps understand histidine's buffering capacity at different pH values.
Why does histidine have three pKa values?
Histidine has three ionizable groups: the α-carboxyl group, the α-amino group, and the imidazole side chain. Each group has a characteristic pKa value representing the pH at which it's 50% protonated. The histidine titration curve demonstrates these three distinct buffering regions related to those pKa values.
What does the plateau region on the histidine titration curve signify?
The plateau regions on the histidine titration curve indicate buffering capacity. At these pH values, histidine is most effective at resisting changes in pH when acid or base is added. This occurs because the histidine is present in approximately equal concentrations of its protonated and deprotonated forms at each pKa.
How can I determine the pI of histidine from its titration curve?
The pI (isoelectric point) of histidine is the pH at which the molecule has no net charge. It can be estimated from the histidine titration curve by averaging the two pKa values that surround the pH where the molecule is predominantly zwitterionic (neutral). This is typically between the α-carboxyl and imidazole pKa values.
So, there you have it! Hopefully, this guide demystified the histidine titration curve a bit. Don't be intimidated – take it step by step, remember those pKa values, and you'll be titrating like a pro in no time. Good luck with your studies!