Nuclei of Helium Atom: Properties & Applications
The nuclei of helium atom, also known as alpha particles, exhibit unique properties that have been pivotal in advancing fields such as nuclear physics and materials science. These particles, composed of two protons and two neutrons, are emitted during the alpha decay of heavy elements, a process extensively studied at institutions like the Lawrence Berkeley National Laboratory. Characterization of the nuclei of helium atom relies heavily on sophisticated instruments, including mass spectrometers, which precisely measure their mass and charge. Their high ionization power, stemming from their +2 charge, makes them effective in applications such as radiation therapy, where they are used to target and destroy cancerous cells with high precision.
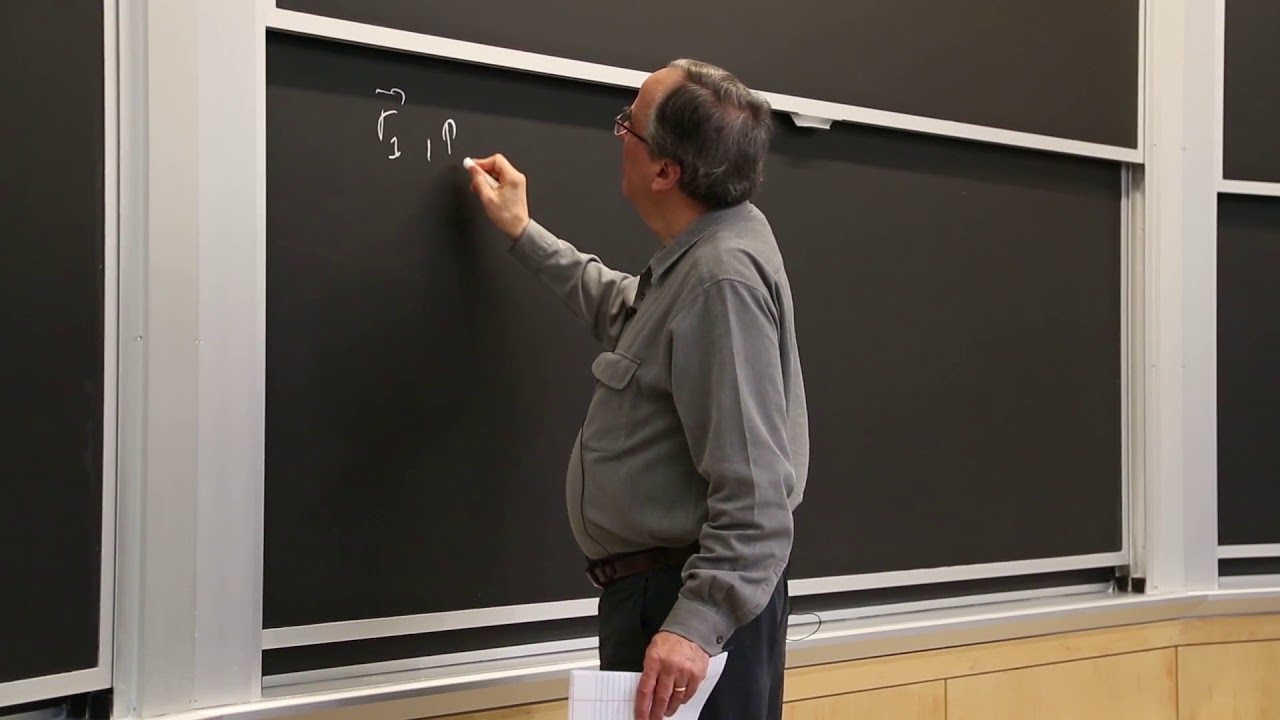
Image taken from the YouTube channel MIT OpenCourseWare , from the video titled 22. Helium Atom .
Unveiling the Alpha Particle: The Helium Nucleus
The helium-4 (⁴He) nucleus, more familiarly known as the alpha particle, represents a cornerstone in our understanding of nuclear physics. Its inherent stability and unique structure make it a subject of intense study. It is a key player in various nuclear processes.
A Fundamental Building Block
The alpha particle isn't merely another entry in the table of nuclides; it occupies a position of fundamental importance. Its properties profoundly influence nuclear structure and interactions. From stellar nucleosynthesis to radioactive decay, the alpha particle's influence is pervasive.
Its composition—two protons and two neutrons—creates a particularly stable configuration. This stability influences the behavior of heavier nuclei. This leads to the emission of alpha particles during radioactive decay.
The Alpha Particle's Role in Nuclear Physics
The study of the alpha particle provides insights into the strong nuclear force. This force binds protons and neutrons together. Without it, atomic nuclei heavier than hydrogen would not exist.
The alpha particle’s relatively simple structure allows scientists to develop and test nuclear models. Understanding its behavior is crucial for advancing theoretical frameworks. This understanding helps us describe more complex nuclear systems.
Alpha Particles in Nature
Alpha particles manifest in diverse natural phenomena, most notably in alpha decay. In this process, unstable heavy nuclei eject alpha particles. This results in the formation of a more stable daughter nucleus.
This decay process is prevalent in elements such as uranium and thorium. It is a primary source of natural background radiation. The energy released during alpha decay also contributes to the Earth’s internal heat.
Significance of Understanding the Helium Nucleus
A comprehensive understanding of the helium nucleus is essential. It provides a foundation for grasping broader concepts in nuclear structure and interactions. Delving into its properties allows researchers to refine nuclear models.
This is crucial for predicting the behavior of other nuclei. Such insights are vital for advancements in nuclear energy, medicine, and materials science. The helium nucleus serves as a vital stepping stone. It helps us unlock the secrets of the nuclear world.
The Alpha Particle's Building Blocks: Composition and Structure
The helium-4 (⁴He) nucleus, more familiarly known as the alpha particle, represents a cornerstone in our understanding of nuclear physics. Its inherent stability and unique structure make it a subject of intense study. It is a key player in various nuclear processes.
A Fundamental Building Block
The alpha particle, at its core, is a tightly bound system consisting of two protons and two neutrons. These constituents, collectively termed nucleons, are confined within an incredibly small volume, approximately 1.7 femtometers in radius. This compact arrangement immediately raises a fundamental question: what force is potent enough to overcome the electrostatic repulsion between the two positively charged protons?
The answer lies in the strong nuclear force, one of the four fundamental forces of nature.
The Strong Nuclear Force: A Binding Power
The strong nuclear force operates exclusively over extremely short distances, effectively binding the nucleons together within the nucleus. This force is significantly stronger than the electromagnetic force at these scales, overpowering the proton-proton repulsion and ensuring the integrity of the helium nucleus.
Without the strong nuclear force, the helium nucleus would simply disintegrate, highlighting its absolutely crucial role in maintaining nuclear stability. The nature of the strong force also explains why larger nuclei don't spontaneously form.
Balancing Act: Overcoming Electrostatic Repulsion
The balance between the attractive strong nuclear force and the repulsive electromagnetic force is critical for nuclear existence. The strong force, mediated by the exchange of particles called gluons, acts between all nucleons, regardless of charge. However, the electromagnetic force acts only between the positively charged protons, creating a destabilizing influence.
The delicate equilibrium between these forces dictates which nuclei are stable and which are prone to radioactive decay.
Binding Energy: Quantifying Nuclear Stability
The binding energy of a nucleus provides a quantitative measure of its stability. It represents the energy required to completely disassemble the nucleus into its constituent protons and neutrons.
The helium-4 nucleus boasts a remarkably high binding energy, a testament to its inherent stability. This immense binding energy translates to a relatively low mass compared to the sum of the masses of its individual nucleons, a difference accounted for by Einstein's famous equation, E=mc².
This mass deficit is released as energy when the nucleons combine to form the nucleus. It is a striking example of the interconvertibility of mass and energy.
Helium-3: A Lighter Cousin
While helium-4 is the dominant isotope of helium, helium-3 (³He), containing two protons and one neutron, also exists. Although less abundant, helium-3 offers valuable insights into nuclear structure.
Its lower neutron count results in a lower binding energy and reduced stability compared to helium-4. This makes it a useful probe for studying nuclear reactions and quantum phenomena.
The contrast between helium-4 and helium-3 showcases how even subtle variations in nucleon composition can drastically alter nuclear properties, providing invaluable data for refining nuclear models and understanding the nuances of the strong nuclear force.
Theoretical Models: Describing the Helium Nucleus
[The Alpha Particle's Building Blocks: Composition and Structure The helium-4 (⁴He) nucleus, more familiarly known as the alpha particle, represents a cornerstone in our understanding of nuclear physics. Its inherent stability and unique structure make it a subject of intense study. It is a key player in various nuclear processes. A Fundamental Buil...]
Understanding the intricacies of the helium nucleus requires the application of sophisticated theoretical models. These models provide a framework for explaining the behavior of nucleons within the nucleus and predicting its properties. They are built upon the foundation of quantum mechanics and incorporate various approximations to tackle the complexities of nuclear interactions.
Quantum Mechanics: The Foundation
Quantum mechanics is indispensable for describing the behavior of nucleons within the helium nucleus. Unlike classical mechanics, which fails at the atomic and subatomic scales, quantum mechanics accurately captures the wave-particle duality of matter and the probabilistic nature of particle interactions.
The Schrödinger equation, a central equation in quantum mechanics, can be used to calculate the energy levels and wave functions of the nucleons within the helium nucleus. These wave functions provide information about the probability of finding a nucleon at a particular location within the nucleus.
Solving the Schrödinger equation for the helium nucleus is a complex task. Due to the strong interactions between the nucleons, approximations must be employed. Nevertheless, these approximations allow us to gain valuable insights into the structure and properties of the helium nucleus.
The Nuclear Shell Model: Orbitals Within the Nucleus
The Nuclear Shell Model offers an intuitive way to understand the energy levels of nucleons within the nucleus. Analogous to the electron shells in atoms, the nuclear shell model postulates that nucleons occupy discrete energy levels or "shells" within the nucleus. These shells are determined by the strong nuclear force and the spin-orbit interaction.
Energy Levels and Nuclear Properties
The Nuclear Shell Model predicts that certain numbers of nucleons lead to particularly stable nuclei. These numbers, known as magic numbers, correspond to completely filled shells. The helium nucleus, with its two protons and two neutrons, is a doubly magic nucleus, explaining its exceptional stability.
Furthermore, the Nuclear Shell Model provides insights into the spin and parity of nuclei. The spin and parity are quantum numbers that characterize the angular momentum and symmetry of the nucleus.
By knowing the configuration of nucleons within the shells, we can predict the spin and parity of the nucleus. This is invaluable for understanding nuclear reactions and decays.
The Liquid Drop Model: A Macroscopic Perspective
The Liquid Drop Model offers a complementary perspective to the Nuclear Shell Model. This model treats the nucleus as a drop of incompressible nuclear fluid. The model accounts for various factors, including the volume energy, surface energy, Coulomb energy, asymmetry energy, and pairing energy.
While the Liquid Drop Model doesn't provide the detailed information about individual nucleon energy levels that the Shell Model offers, it is useful for understanding the overall stability of nuclei and predicting nuclear masses.
Spin and Isospin: Intrinsic Quantum Numbers
Beyond spatial coordinates, nucleons possess intrinsic quantum numbers: spin and isospin. Spin is the intrinsic angular momentum of a particle. Nucleons have a spin of 1/2. Isospin is a quantum number related to the strong interaction. It describes the similarity between protons and neutrons. Protons and neutrons are considered two states of the same particle (the nucleon) with different isospin projections.
These quantum numbers play a significant role in determining the interactions between nucleons and the overall properties of the helium nucleus. The Pauli exclusion principle dictates that no two identical fermions (like nucleons) can occupy the same quantum state. This principle directly affects nuclear stability.
The Uncertainty Principle
Werner Heisenberg's Uncertainty Principle fundamentally limits the precision with which we can simultaneously know a particle's position and momentum. This principle has profound implications for understanding nucleon behavior within the helium nucleus. Because the nucleons are confined within a small space, their momentum (and therefore energy) exhibits a corresponding uncertainty.
This uncertainty contributes to the dynamic nature of the nucleus and makes it impossible to precisely predict the instantaneous position and momentum of each nucleon. The nucleus should be viewed as a probabilistic entity, in constant flux rather than a static arrangement of particles.
Pioneers of Discovery: Key Figures in Helium Nucleus Research
Our modern understanding of the helium nucleus, and indeed the broader field of nuclear physics, rests on the shoulders of giants. This section pays homage to the pioneering scientists whose meticulous experiments and groundbreaking insights unveiled the secrets of the alpha particle. From scattering experiments to the discovery of the neutron, their contributions have shaped our comprehension of the fundamental building blocks of matter.
Rutherford's Alpha Scattering: Unveiling the Nuclear Atom
Ernest Rutherford stands as a towering figure in the history of physics, renowned for his revolutionary work on radioactivity and the structure of the atom. His alpha scattering experiments, conducted in the early 20th century, irrevocably altered the prevailing "plum pudding" model of the atom.
Rutherford directed a beam of alpha particles at a thin gold foil. The results defied expectations. While most alpha particles passed straight through, a small fraction were deflected at large angles, and some even bounced back.
This startling observation led Rutherford to propose a new atomic model. This model envisioned a tiny, dense, positively charged nucleus at the center of the atom, surrounded by orbiting electrons. The Rutherford model remains a cornerstone of atomic theory.
Geiger and Marsden: The Art of Careful Observation
Hans Geiger and Ernest Marsden, under Rutherford's guidance, played crucial roles in the execution and interpretation of the alpha scattering experiments. Their meticulous work in observing and quantifying the scattering patterns provided the essential data that underpinned Rutherford's conclusions.
Geiger and Marsden dedicated themselves to the tedious task of counting scintillations produced when alpha particles struck a screen. Their work required immense patience and careful attention to detail. Without their contributions, Rutherford's theoretical leap would have lacked the empirical foundation necessary for acceptance. Their collaboration highlights the importance of experimental precision in scientific discovery.
Chadwick's Neutron: Completing the Picture
The discovery of the neutron by James Chadwick in 1932 was a watershed moment in nuclear physics. Prior to Chadwick's work, the composition of the nucleus remained a puzzle. Scientists knew that nuclei contained protons, but the atomic mass of many elements exceeded what could be accounted for by protons alone.
Chadwick, building upon earlier observations of unusual radiation emitted from beryllium when bombarded with alpha particles, hypothesized the existence of a neutral particle with a mass similar to that of the proton. Through a series of elegant experiments, he demonstrated the existence of this particle, which he named the neutron.
The discovery of the neutron completed our understanding of the nucleus as being composed of protons and neutrons. It paved the way for further advances in nuclear physics, including the development of nuclear energy and nuclear weapons.
Marie Curie: Radioactivity and the Alpha Particle's Source
Marie Curie's pioneering research on radioactivity laid the groundwork for our understanding of alpha decay and the properties of alpha particles. Her painstaking work in isolating radioactive elements, such as radium and polonium, revealed the phenomenon of spontaneous emission of radiation.
Curie's investigations demonstrated that alpha particles were emitted during the radioactive decay of certain elements. She identified that these particles were positively charged and relatively heavy, eventually leading to their identification as helium nuclei.
Her contributions not only advanced our knowledge of radioactivity but also had a profound impact on medicine and other fields. Curie's legacy extends far beyond the laboratory, inspiring generations of scientists and solidifying her place as one of the most important figures in scientific history.
Radioactive Decay: Alpha Emission from Unstable Nuclei
Having explored the fundamental structure and theoretical models of the helium nucleus, it is essential to consider one of its most significant roles in nature: its emission as an alpha particle during radioactive decay. Alpha decay represents a primary pathway for unstable, heavy nuclei to achieve a more stable configuration. This section will delve into the mechanics of alpha decay, the energetics involved, and the resulting nuclear transformations, offering a comprehensive understanding of this ubiquitous phenomenon.
The Process of Alpha Decay
Alpha decay is a type of radioactive decay in which an atomic nucleus emits an alpha particle (⁴He nucleus) and thereby transforms or decays into a different atomic nucleus, with a mass number reduced by 4 and an atomic number reduced by 2. This process is governed by the fundamental laws of physics and represents a crucial mechanism for heavy, unstable nuclei to shed excess energy and nucleons.
This spontaneous emission is a quantum mechanical tunneling phenomenon, where the alpha particle, despite being bound by the strong nuclear force, has a non-zero probability of escaping the potential well of the nucleus.
The parent nucleus (the original nucleus) transforms into a daughter nucleus (the new nucleus) and an alpha particle. This transformation can be represented by the following general equation:
XA -> A-4Y + ⁴He
Z Z-2 2
Where:
- X is the parent nucleus.
- Y is the daughter nucleus.
- A is the mass number.
- Z is the atomic number.
- ⁴He is the alpha particle. 2
Energy Release and Nuclear Transformation
The release of an alpha particle during radioactive decay is accompanied by the release of energy, often referred to as the Q-value of the decay.
This energy manifests as the kinetic energy of the alpha particle and the recoiling daughter nucleus. The Q-value is determined by the difference in mass between the parent nucleus and the combined mass of the daughter nucleus and the alpha particle, as dictated by Einstein's famous equation, E=mc².
A positive Q-value indicates that the decay is energetically favorable and can occur spontaneously. The kinetic energy is partitioned between the alpha particle and the daughter nucleus, with the lighter alpha particle receiving a greater share due to conservation of momentum.
The emission of an alpha particle results in a significant nuclear transformation.
Specifically, the daughter nucleus has a mass number four units lower and an atomic number two units lower than the parent nucleus. This change in atomic number signifies the transmutation of one element into another.
For instance, uranium-238 (²³⁸U) decays via alpha emission into thorium-234 (²³⁴Th).
Alpha Particle Sources and Applications
Substances that undergo alpha decay are known as alpha particle sources. These sources have various applications in research, industry, and medicine, though their use is often carefully regulated due to the ionizing nature of alpha particles.
One notable application is in smoke detectors, where a small amount of americium-241 (²⁴¹Am) emits alpha particles. These particles ionize the air within the detector, creating a current.
When smoke enters the detector, it disrupts this current, triggering the alarm.
In scientific research, alpha particle sources are used in Rutherford backscattering spectrometry (RBS), a technique for analyzing the composition and structure of materials. Alpha particles are directed at a sample, and the energy and angle of the backscattered particles provide information about the elements present and their depth distribution.
Moreover, alpha emitters are being explored in targeted cancer therapy. Alpha particles are highly effective at killing cancer cells due to their high linear energy transfer (LET), meaning they deposit a large amount of energy over a short distance. This localized energy deposition minimizes damage to surrounding healthy tissue.
However, the challenge lies in delivering the alpha emitter specifically to the tumor site. Research is focused on developing targeting molecules that selectively bind to cancer cells, delivering the alpha emitter directly where it is needed.
Practical Applications: Harnessing the Power of Helium Nuclei
Having explored the fundamental structure and theoretical models of the helium nucleus, it is essential to consider one of its most significant roles in nature: its emission as an alpha particle during radioactive decay. Beyond this, the helium nucleus, in its various isotopic forms, presents a spectrum of practical applications, ranging from energy production to medical treatments and fundamental research. This section explores several of these key applications, revealing the diverse and impactful ways in which we are learning to harness the power of helium nuclei.
Helium-3 Fusion: A Potential Future Energy Source
Helium-3 (³He) fusion represents a tantalizing prospect for future energy production.
Unlike conventional nuclear fusion reactions involving deuterium and tritium, ³He fusion offers the potential for aneutronic reactions.
These reactions release substantially less energy in the form of neutrons, significantly reducing the challenges associated with neutron activation of reactor materials and the handling of radioactive waste.
The primary reaction of interest is: ³He + D → ⁴He + p + 18.3 MeV.
This reaction produces helium-4 (⁴He) and a proton (p), releasing a substantial amount of energy.
The challenge, however, lies in the scarcity of ³He on Earth.
The Moon has been identified as a potentially rich source of ³He, deposited by solar wind over billions of years.
Extracting and transporting this lunar ³He would require significant technological advancements and investment, but the potential rewards of a clean, abundant energy source are considerable.
While ³He fusion remains a long-term prospect, ongoing research and development efforts are gradually bringing this technology closer to realization.
Helium Nuclei in Nuclear Medicine: Targeted Cancer Treatments
Alpha particles, due to their high energy and short range in matter, hold promise for targeted cancer therapies.
Alpha particles can deliver a highly localized dose of radiation to cancer cells, minimizing damage to surrounding healthy tissues.
This precision is particularly valuable in treating small, localized tumors or cancer cells that have spread microscopically.
Targeted Alpha Therapy (TAT)
Targeted Alpha Therapy (TAT) involves attaching alpha-emitting radionuclides to targeting molecules, such as antibodies or peptides, that selectively bind to cancer cells.
These targeting molecules act as delivery vehicles, bringing the alpha particles directly to the tumor.
Upon decay, the alpha particles deposit their energy within a short range, typically only a few cell diameters, effectively destroying the cancer cells while sparing nearby healthy tissue.
Actinium-225 and Thorium-227 are commonly used alpha-emitting radionuclides in TAT research and clinical trials.
TAT is showing promising results in treating various cancers, including leukemia, lymphoma, and prostate cancer.
As research progresses and new targeting molecules are developed, TAT is poised to become an increasingly important tool in the fight against cancer.
Alpha Particle Sources: Industrial and Scientific Applications
Alpha particles, emitted from radioactive sources, have diverse industrial and scientific applications.
These applications leverage the unique properties of alpha particles, such as their high ionization power and short range, for various purposes.
Smoke Detectors
One of the most common applications is in smoke detectors.
These devices use a small amount of americium-241, which emits alpha particles.
The alpha particles ionize the air within the detector, creating a small electric current.
When smoke enters the detector, it disrupts the ionization process, causing a decrease in the current and triggering an alarm.
Gauging and Thickness Measurement
Alpha particles can also be used for gauging and thickness measurement in various industries.
By measuring the amount of alpha particles that pass through a material, it is possible to determine its thickness or density.
This technique is employed in the production of paper, plastics, and metal foils, allowing for precise control of product quality.
Role in High-Energy Physics Experiments
Helium nuclei play a crucial role in high-energy physics experiments, serving as both projectiles and targets in nuclear reactions.
Heavy Ion Collisions
Accelerated beams of helium nuclei, or alpha particles, are used in heavy-ion colliders.
These colliders smash beams of heavy ions together at extremely high energies, creating conditions similar to those that existed in the early universe.
By studying the resulting particles and interactions, physicists can gain insights into the fundamental forces of nature and the structure of matter.
Nuclear Structure Studies
Helium nuclei can also be used as projectiles in nuclear scattering experiments.
By bombarding target nuclei with alpha particles and analyzing the scattered particles, physicists can probe the structure of the target nuclei and learn about the distribution of protons and neutrons within them.
These experiments provide valuable data for testing and refining theoretical models of nuclear structure.
Video: Nuclei of Helium Atom: Properties & Applications
FAQs About Helium Nuclei
What is a helium nucleus composed of?
A helium nucleus, often called an alpha particle, is composed of two protons and two neutrons tightly bound together. This makes it a very stable particle.
Why are helium nuclei used in some types of radiotherapy?
The high mass and charge of helium nuclei make them effective at depositing energy over a short range. This localized energy deposition is useful for targeting cancer cells while minimizing damage to surrounding healthy tissue.
How does the binding energy of a helium nucleus affect its stability?
Helium nuclei have a very high binding energy per nucleon. This strong binding energy contributes to their exceptional stability, making them less likely to undergo nuclear reactions or decay compared to some other nuclei.
What role do helium nuclei play in nuclear fusion reactions in stars?
Helium nuclei are a fundamental product of nuclear fusion reactions, specifically the proton-proton chain and the CNO cycle, which power stars like our Sun. These reactions involve lighter elements fusing to form nuclei of helium atom, releasing tremendous amounts of energy.
So, that's the story of helium nuclei! From their surprising stability to their versatile applications in fields like nuclear physics and medicine, these tiny particles pack a serious punch. Hopefully, this gave you a better understanding of the remarkable properties and uses of nuclei of helium atom, and maybe even sparked a little curiosity about the amazing world of nuclear science!