Geographic Isolation Definition Biology: Guide
Geographic isolation, a crucial mechanism of evolution, describes a scenario where a population of organisms becomes separated by physical barriers. These barriers, often categorized under the umbrella of biogeography, can range from imposing mountain ranges, which significantly limit species mobility and interaction, to expansive oceans, effectively creating discrete habitats. This separation impedes gene flow, a concept thoroughly explored by population geneticists such as Sewall Wright, and sets the stage for divergent evolutionary pathways, leading to speciation. Understanding the intricacies of the geographic isolation definition biology reveals how environmental factors mold biodiversity.
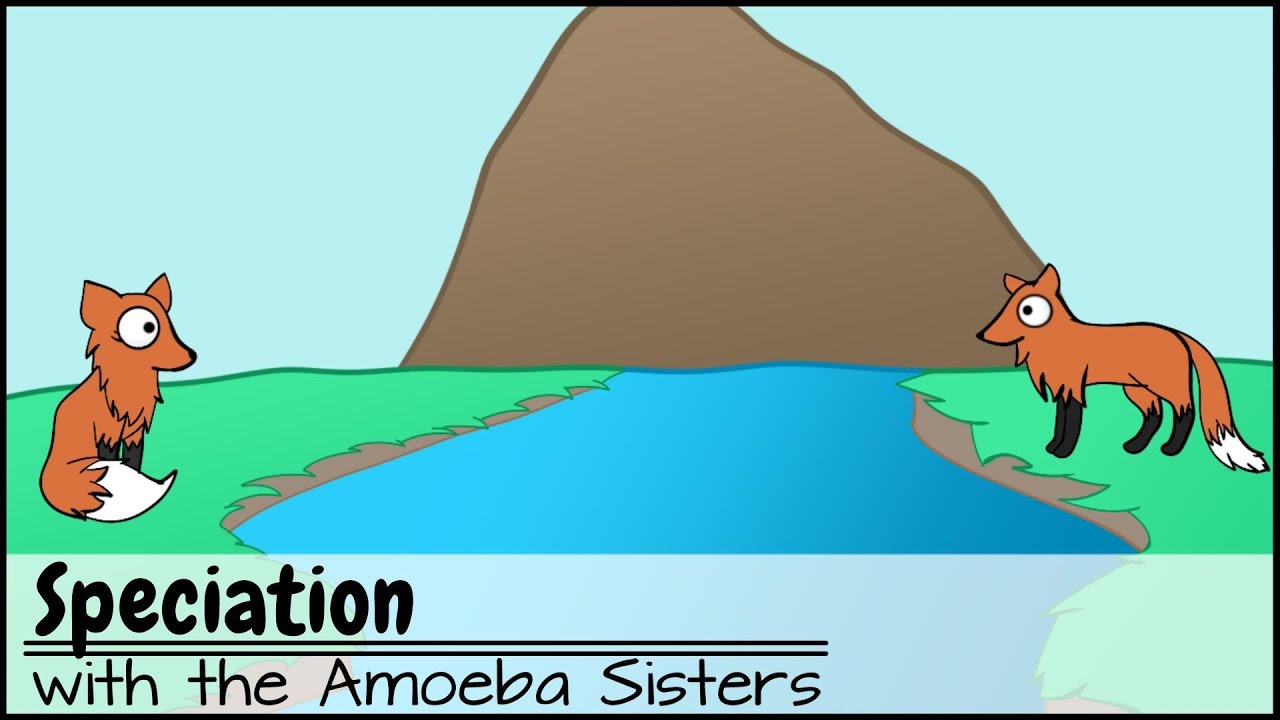
Image taken from the YouTube channel Amoeba Sisters , from the video titled Speciation .
Geographic isolation stands as a cornerstone concept in evolutionary biology, explaining how new species arise through physical separation. This introduction will explore the fundamental role of geographic isolation in the grand narrative of speciation. We will define the term, emphasize allopatric speciation as the most prominent outcome, and briefly touch upon other modes of speciation to provide a comprehensive context.
Defining Geographic Isolation
At its core, geographic isolation refers to the physical separation of populations, effectively preventing gene flow between them. This separation can arise from various factors, such as the formation of mountain ranges, the creation of new islands, or even something as simple as a river changing course. The critical element is that the physical barrier hinders or completely stops the exchange of genetic material, setting the stage for independent evolutionary trajectories.
Allopatric Speciation: The Primary Outcome
Geographic isolation is most directly linked to allopatric speciation, often considered the most common mode of species formation. Allopatric speciation unfolds when populations are geographically separated, leading to independent evolution in response to differing environmental conditions and genetic drift.
Over time, these isolated populations accumulate genetic differences. Natural selection favors traits advantageous in their respective environments. This divergence, unchecked by gene flow, eventually leads to reproductive isolation.
When the separated populations eventually come into contact again, they can no longer interbreed successfully. They are, at that point, distinct species.
Other Speciation Modes in Context
While allopatric speciation is the focus due to its direct reliance on geographic separation, it's essential to acknowledge other modes of speciation for a comprehensive view. Sympatric speciation occurs within the same geographic area, often driven by strong selection pressures or genetic mutations that lead to reproductive isolation despite the lack of physical barriers.
Parapatric speciation, on the other hand, occurs when populations are adjacent to each other, with limited gene flow in a narrow contact zone. In this case, strong selection pressures across the environmental gradient can drive divergence and ultimately speciation, despite the absence of a complete physical barrier. Understanding these different modes provides a more complete picture of the complex processes driving the evolution of biodiversity.
Having established the concept of geographic isolation and its role in speciation, it is now crucial to delve into the specific mechanisms by which populations become physically separated. Understanding these mechanisms is essential for comprehending the initiation of evolutionary divergence. We will explore vicariance, dispersal, and the diverse array of barriers that impede gene flow, providing concrete examples to illustrate each process.
The Mechanisms of Separation: How Populations Become Isolated
Geographic isolation, the cornerstone of allopatric speciation, doesn't occur spontaneously. Specific mechanisms drive the physical separation of populations, setting the stage for independent evolutionary trajectories. These mechanisms can be broadly categorized into vicariance, dispersal, and the presence of diverse barriers to gene flow, each playing a unique role in shaping biodiversity.
Vicariance: Separation by Newly Formed Barriers
Vicariance, derived from the Latin "vicarius" meaning substitute, refers to the separation of an ancestral population due to the emergence of a new physical barrier. This barrier effectively divides the population, preventing gene flow and allowing each subpopulation to evolve independently.
Defining Vicariance
At its core, vicariance involves the fragmentation of a previously continuous population’s range. This fragmentation is not due to the population's active movement, but rather to geological or environmental events that create a divide.
The key is that the barrier arises within the existing range of the species, splitting it into two or more isolated groups.
The Isthmus of Panama: A Classic Example
The formation of the Isthmus of Panama, approximately 3 million years ago, provides a compelling example of vicariance. This land bridge connected North and South America, simultaneously creating a barrier that separated the Caribbean Sea from the Pacific Ocean.
The consequences were profound. Marine species, such as snapping shrimp, were divided into Atlantic and Pacific populations. These populations, now geographically isolated, began to diverge genetically due to differing environmental conditions and genetic drift.
Today, many sister species exist on either side of the isthmus, representing the direct result of vicariant speciation. This event also facilitated the Great American Interchange, with significant faunal exchange between the two continents.
Continental Drift: A Grand Scale Example
On a much larger timescale, continental drift has played a pivotal role in vicariance. The breakup of supercontinents like Gondwana led to the separation of numerous plant and animal populations.
The evolutionary history of flightless birds (ratites) provides strong evidence for this. Species like ostriches in Africa, rheas in South America, and emus and cassowaries in Australia are thought to have evolved from a common ancestor before the continents drifted apart. Their current distribution reflects the ancient vicariance event caused by continental drift.
Dispersal: Movement to New Isolated Areas
In contrast to vicariance, which involves a static population being divided, dispersal involves the active movement of a subset of a population to a new, previously uninhabited area.
This movement establishes a new, isolated population that can then evolve independently from the source population.
Defining Dispersal
Dispersal occurs when a few individuals from a larger population migrate to a new location, often across a significant barrier. This new location must be sufficiently isolated to prevent ongoing gene flow with the original population.
The success of dispersal depends on the ability of the organisms to overcome the challenges of the journey and establish themselves in the new environment.
The Founder Effect: A Consequence of Dispersal
A crucial aspect of dispersal is the founder effect. This phenomenon occurs when the small founding population does not carry the full genetic diversity of the original population.
Certain alleles may be overrepresented, while others may be entirely absent. This altered allele frequency in the founding population can lead to rapid evolutionary divergence from the parent population.
Island colonizations are prime examples. The genetic makeup of island populations often reflects the founder effect, leading to unique evolutionary trajectories and the emergence of endemic species.
Barriers to Gene Flow: Impeding Genetic Exchange
Barriers to gene flow are obstacles that prevent or significantly reduce the exchange of genetic material between populations. These barriers can be physical, ecological, or even behavioral.
While some barriers may not completely prevent gene flow, they can reduce it sufficiently to allow for genetic divergence and eventual speciation.
Defining Barriers to Gene Flow
Barriers to gene flow are diverse, ranging from obvious physical obstacles like mountains and rivers to more subtle ecological differences that limit interbreeding.
The effectiveness of a barrier depends on the species in question; what is a significant barrier for one organism may be easily traversable for another.
Mountains: Impeding Terrestrial Movement
Mountain ranges represent significant barriers for many terrestrial species. They create physical obstacles that limit movement and gene flow between populations on either side.
The high altitude, rugged terrain, and distinct climate zones can create strong selective pressures, further driving divergence.
Rivers: Barriers for Non-Aquatic Organisms
Rivers can act as effective barriers for non-aquatic organisms. While birds and strong-flying insects may easily cross rivers, many small mammals, reptiles, and amphibians are restricted by them.
The width, depth, and current of a river all influence its effectiveness as a barrier to gene flow.
Oceans: Major Barriers to Terrestrial Species
Oceans represent major barriers for terrestrial species. The vast expanse of saltwater is impassable for most land-dwelling organisms, leading to significant isolation of island populations.
This isolation is a primary driver of endemism, with many island species found nowhere else on Earth. The unique flora and fauna of islands like Madagascar and the Galapagos are testaments to the power of oceanic barriers.
Deserts: Isolating Moist-Environment Species
Deserts, with their arid conditions and extreme temperatures, can create effective barriers for species that require moist environments. Patches of suitable habitat within a desert become isolated "islands" for these species.
The resulting fragmentation of populations leads to localized adaptation and potential speciation.
In conclusion, the mechanisms that lead to geographic isolation are multifaceted and diverse. Vicariance, dispersal, and barriers to gene flow each contribute to the fragmentation of populations, setting the stage for independent evolution and the generation of biodiversity. Understanding these mechanisms is critical for comprehending the processes that shape the natural world.
Evolutionary Consequences: From Isolation to Divergence
Geographic isolation initiates a cascade of evolutionary events that ultimately lead to divergence and, potentially, the formation of new species. By severing the connections between populations, isolation allows other evolutionary forces to act independently, driving each population down its own unique evolutionary path.
These forces—gene flow, genetic drift, and natural selection—interact in complex ways to reshape the genetic makeup of isolated groups, resulting in distinct morphological, behavioral, and physiological differences.
The Attenuation of Gene Flow
Gene flow, defined as the movement of genes between populations, is a potent homogenizing force. It counteracts divergence by introducing new alleles and spreading existing ones, thereby maintaining genetic similarity across populations.
Geographic isolation directly undermines gene flow. When populations are physically separated, the exchange of genetic material is significantly reduced or entirely eliminated.
This cessation of gene flow is a critical first step in the divergence process, as it allows each isolated population to accumulate genetic differences independently.
Genetic Drift: The Random Walk of Alleles
Genetic drift refers to the random fluctuations in allele frequencies within a population. These fluctuations are particularly pronounced in small populations, where chance events can have a disproportionately large impact on the genetic makeup of the group.
Geographic isolation often leads to smaller population sizes, either through the founder effect during dispersal or simply as a consequence of habitat fragmentation during vicariance.
In these isolated, smaller populations, genetic drift can be accelerated, leading to rapid and unpredictable changes in allele frequencies.
Some alleles may become fixed (reaching a frequency of 100%), while others may be lost entirely, irrespective of their adaptive value.
This random process contributes significantly to the divergence between isolated populations.
Natural Selection: Sculpting Adaptation
Natural selection, the differential survival and reproduction of individuals based on their traits, is a primary driver of adaptation. In geographically isolated populations, natural selection can lead to divergence as each population adapts to its unique local environment.
Different environments impose different selective pressures. For instance, one population might face intense predation pressure, while another might experience a scarcity of resources.
These differing selective pressures favor different traits in each population, leading to the evolution of distinct adaptations.
Over time, these adaptations can accumulate, resulting in substantial morphological, physiological, and behavioral differences between the isolated groups.
Divergence: The Path to Distinctness
Divergence is the process by which populations evolve to become increasingly different from one another. It is the cumulative result of reduced gene flow, genetic drift, and natural selection acting independently on isolated populations.
The manifestations of divergence can be observed at multiple levels, including morphological differences (e.g., body size, shape, coloration), behavioral differences (e.g., mating rituals, foraging strategies), and genetic differences (e.g., changes in DNA sequence, chromosome structure).
As divergence progresses, the isolated populations become increasingly distinct, both genetically and phenotypically.
Reproductive Isolation: The Point of No Return
Reproductive isolation is the ultimate consequence of geographic isolation and divergence. It refers to the inability of different populations to interbreed and produce viable, fertile offspring.
Reproductive isolation is essential for the completion of speciation, as it prevents the merging of diverging gene pools.
Reproductive isolation can arise through various mechanisms, categorized as prezygotic and postzygotic barriers.
Prezygotic Barriers
Prezygotic barriers prevent the formation of a zygote (a fertilized egg). These barriers include:
- Habitat isolation: Populations live in different habitats and do not interact.
- Temporal isolation: Populations breed at different times of day or year.
- Behavioral isolation: Populations have different courtship rituals or mating preferences.
- Mechanical isolation: Populations have incompatible reproductive structures.
- Gametic isolation: Populations have incompatible eggs and sperm.
Postzygotic Barriers
Postzygotic barriers occur after the formation of a hybrid zygote. These barriers include:
- Reduced hybrid viability: Hybrid offspring are unable to survive.
- Reduced hybrid fertility: Hybrid offspring are infertile.
- Hybrid breakdown: First-generation hybrids are fertile, but subsequent generations are infertile.
Speciation or Hybridization?
The development of reproductive isolation determines the ultimate fate of geographically isolated populations. If reproductive isolation is complete, the populations will continue to diverge until they become distinct species, incapable of interbreeding.
However, if reproductive isolation is incomplete, the populations may still be able to interbreed, resulting in the formation of hybrid zones.
Hybrid zones are regions where two previously isolated populations come into contact and interbreed, producing hybrid offspring. The stability and long-term outcome of hybrid zones depend on the fitness of the hybrids and the extent of gene flow between the parental populations.
In conclusion, geographic isolation sets in motion a series of evolutionary processes that reshape the genetic makeup and phenotypic characteristics of isolated populations. These processes, including reduced gene flow, genetic drift, and natural selection, lead to divergence and, ultimately, reproductive isolation, paving the way for the formation of new species and the diversification of life on Earth.
Island Biogeography: Natural Laboratories of Evolution
Islands, by their very nature, represent compelling instances of geographic isolation, rendering them invaluable as natural laboratories for the study of evolutionary processes. Separated from mainland ecosystems by expanses of water, island populations are subject to unique selective pressures and evolutionary dynamics. This combination of isolation and novel environments has made islands key sites for understanding the mechanisms driving speciation and adaptation.
Islands as Evolutionary Arenas
Islands are not merely isolated landmasses; they are dynamic arenas where the forces of evolution play out in accelerated and often dramatic ways.
The reduced gene flow inherent in island populations, coupled with the effects of genetic drift and natural selection, can lead to rapid divergence from mainland relatives.
This makes islands ideal locations for observing the early stages of speciation and for testing evolutionary hypotheses.
The Significance of Isolation
The geographic isolation of islands is paramount to their evolutionary significance. This isolation limits gene flow, which is a crucial factor.
Without the constant influx of new genes from mainland populations, island populations are free to evolve along their own trajectory.
The limited gene flow, in conjunction with unique environmental conditions, often results in the emergence of endemic species.
Island Biogeography: Unveiling the Interplay of Richness and Isolation
The field of island biogeography, pioneered by Robert MacArthur and E.O. Wilson, provides a theoretical framework for understanding the factors that determine species richness on islands.
Their equilibrium theory of island biogeography posits that the number of species on an island is determined by a dynamic equilibrium between immigration and extinction rates.
The rate of immigration is influenced by the distance of the island from the mainland, while the rate of extinction is influenced by the size of the island.
The Size-Distance Relationship
The equilibrium theory highlights two key factors affecting species richness: island size and distance from the mainland.
Larger islands tend to support more species due to greater habitat diversity and larger population sizes, which reduce the risk of extinction.
Islands closer to the mainland experience higher rates of immigration, leading to greater species richness.
This size-distance relationship is a fundamental principle of island biogeography and has been supported by numerous empirical studies.
Endemic Species: A Testament to Isolation
One of the most striking features of island ecosystems is the high proportion of endemic species – species that are found nowhere else on Earth.
Endemism is a direct consequence of geographic isolation and the unique evolutionary pressures experienced by island populations.
Over time, isolated populations accumulate genetic differences that lead to the evolution of distinct species that are uniquely adapted to their island home.
Examples of Island Endemism
Classic examples of island endemism include the Galapagos finches, which played a pivotal role in Darwin's development of the theory of evolution by natural selection.
Each finch species has evolved a unique beak morphology that is adapted to a specific food source on the islands.
Other notable examples include the lemurs of Madagascar, the Hawaiian honeycreepers, and the flightless birds of New Zealand.
These endemic species serve as powerful reminders of the evolutionary potential of isolated environments and the importance of conserving island ecosystems.
The Vulnerability of Endemic Species
While endemic species are a source of great biodiversity, they are also particularly vulnerable to extinction.
Their restricted geographic range makes them susceptible to habitat loss, invasive species, and climate change.
The introduction of non-native predators, competitors, or diseases can have devastating consequences for endemic populations.
The conservation of island ecosystems is therefore crucial for preserving the unique evolutionary heritage they harbor.
Tools of the Trade: Studying Isolation and Speciation
Understanding the intricate processes of geographic isolation and speciation requires a robust toolkit of scientific methods. From unraveling the genetic code to reconstructing evolutionary trees, researchers employ sophisticated techniques to explore the mechanisms driving the formation of new species.
Among these methods, DNA sequencing and phylogenetic analysis stand out as indispensable tools for assessing genetic differences and charting evolutionary trajectories. These approaches provide critical insights into the historical and contemporary dynamics of species divergence.
DNA Sequencing: Unveiling Genetic Divergence
DNA sequencing has revolutionized the study of evolution, providing a detailed view of the genetic makeup of organisms. By determining the precise order of nucleotides in an organism's DNA, scientists can quantify the genetic differences between populations.
This information is invaluable for assessing the extent of genetic divergence that has occurred following geographic isolation. Regions of the genome that exhibit significant variation between isolated populations can pinpoint areas under selection or those affected by genetic drift.
Furthermore, DNA sequencing allows for the identification of unique mutations that have arisen in isolated populations. The presence and frequency of these mutations serve as markers of their evolutionary history, thus illustrating the divergence process.
Applications of DNA Sequencing
DNA sequencing plays a pivotal role in various aspects of speciation research. Comparative genomics, enabled by sequencing technology, reveals the specific genes and regulatory elements that contribute to adaptive differences between newly formed species.
Metagenomic studies further expand the scope of analysis by examining the genetic diversity within entire communities. This allows for a comprehensive understanding of how geographic isolation impacts the interplay of species and their environment.
Phylogenetic Analysis: Reconstructing Evolutionary History
Phylogenetic analysis uses genetic data to reconstruct the evolutionary relationships among species or populations. By creating phylogenetic trees, scientists can visualize the branching patterns of lineage divergence and estimate the timing of speciation events.
These trees are constructed based on the principle that species with more similar DNA sequences are more closely related. Sophisticated algorithms analyze DNA sequence data to create a visual representation of these relationships.
Phylogenetic analysis provides a framework for understanding how geographic isolation has shaped the diversity of life on Earth. It can reveal instances of convergent evolution, where geographically isolated populations evolve similar traits independently due to similar environmental pressures.
Application in Speciation Studies
Phylogenetic trees are indispensable for determining if speciation has occurred following a period of geographic isolation. If populations that were once connected now form distinct and well-supported branches on a phylogenetic tree, this provides strong evidence of speciation.
Moreover, phylogenetic analysis can reveal the geographic origins and dispersal routes of species. By mapping the distribution of genetic diversity onto a phylogenetic tree, scientists can trace the historical movements of populations and understand how geographic barriers have influenced their evolutionary trajectories.
Combining phylogenetic analysis with biogeographic data allows for a comprehensive understanding of the interplay between geographic isolation and evolutionary divergence. This integration is crucial for unraveling the complex history of life and informing conservation strategies.
Video: Geographic Isolation Definition Biology: Guide
Frequently Asked Questions
How does geographic isolation actually lead to new species?
Geographic isolation definition biology: It prevents gene flow between populations. Over time, the isolated groups accumulate different genetic mutations. If these genetic differences become significant enough to prevent successful interbreeding, new species are formed.
What are some examples of geographic barriers?
Examples of geographic barriers include mountain ranges, large bodies of water (oceans, lakes), deserts, and glaciers. These barriers physically separate populations, contributing to geographic isolation definition biology.
Is geographic isolation the only way new species can evolve?
No. While geographic isolation definition biology is a common mechanism, new species can also arise through reproductive isolation without geographic separation. This is known as sympatric speciation and can occur through mechanisms like polyploidy or disruptive selection.
Can a barrier be considered "geographic" if it's man-made?
Yes, man-made structures like roads, dams, or large-scale deforestation can act as geographic barriers. Geographic isolation definition biology simply requires physical separation that limits or prevents gene flow, regardless of the barrier's origin.
So, that's geographic isolation definition biology in a nutshell! Hopefully, this guide has given you a clearer understanding of how mountains, rivers, and even deserts can play a huge role in the evolution of life. Now you can impress your friends with your newfound knowledge!