How Are Fault Block Mountains Formed? Tilted Giants
Enormous landforms, known as fault block mountains, dramatically reshape our planet’s surface through the relentless forces of geology; the Basin and Range Province in the western United States stands as a superb example of this phenomenon. Tectonic plates, driven by the Earth's internal heat, exert tremendous stress on the lithosphere, creating extensive fractures known as faults. The United States Geological Survey (USGS) provides comprehensive data on these geological processes, aiding scientists in understanding the mechanics of mountain building. Understanding how are fault block mountains formed involves examining these fault lines and their subsequent vertical displacement, a process often analyzed with sophisticated modeling software likeMove™ by Petroleum Experts, used to simulate geological deformation.
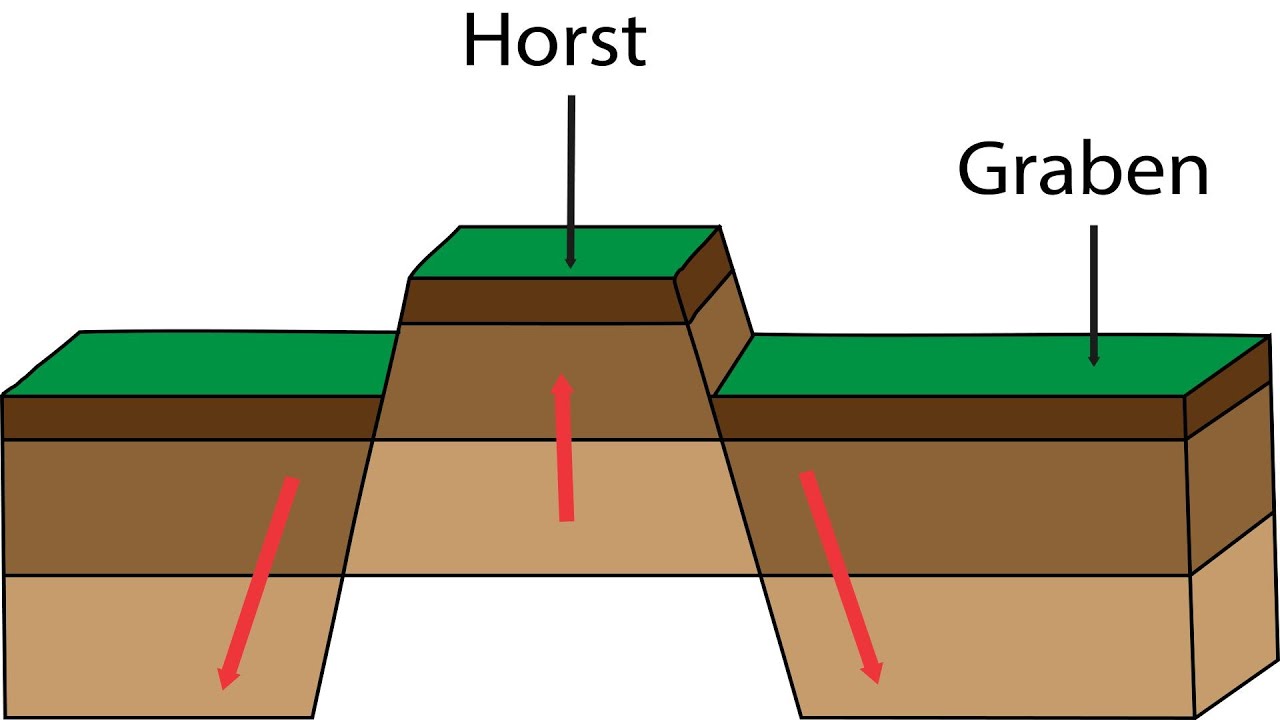
Image taken from the YouTube channel Classroom Nation - Geography , from the video titled How block mountains are formed .
Cracks in the Earth: Exploring Faults and Rifting
The Earth beneath our feet is anything but static. It's a dynamic, ever-shifting puzzle of tectonic plates, driven by forces deep within our planet.
These immense forces shape our world, creating everything from towering mountain ranges to vast, sprawling valleys.
At the heart of many of these dramatic landscapes lies the concept of faults and rifts.
What is a Fault?
Imagine a giant slab of rock, fractured and broken along a jagged line. Now, picture those broken pieces sliding against each other.
That, in essence, is a fault.
A fault is a fracture in the Earth’s crust where there has been measurable movement.
This movement can be slow and gradual, or sudden and catastrophic, leading to earthquakes.
Faults are fundamental to understanding how the Earth's crust deforms and responds to stress.
The Power of Rifting
Rifting is another crucial concept.
Rifting is the geological process where the Earth’s lithosphere stretches and pulls apart.
This extensional force creates a zone of weakness, a place where the crust begins to thin and eventually break.
Think of it like slowly pulling on a piece of taffy – it stretches, becomes thinner, and eventually snaps.
Unveiling the Landscape
This blog post is your gateway to understanding the fascinating world of faults and rifting.
We'll explore the spectacular landforms that arise from these processes. We'll delve into the mechanisms that sculpt our planet.
Prepare to discover the tilted giants and sunken valleys born from the Earth's powerful internal forces!
Understanding Faults: Normal Faults and Extensional Forces
Now that we've laid the groundwork with faults and rifting, let's zoom in and understand these incredible geological features.
Specifically, we will be looking at normal faults, the workhorses of extensional terrains. And the extensional forces that drive them.
Prepare to have the Earth's stretching and straining revealed!
Defining the Normal Fault: A Matter of Relative Motion
Imagine a climbing wall.
On one side of a crack (the fault!) is a climber standing there. He stays perfectly still.
The other side of the crack slides down relative to the first guy.
This sliding down is the key to understanding a normal fault.
In geological terms, we define a normal fault by the movement of its two sides, or blocks.
The Hanging Wall
The hanging wall is the block of rock that lies above the fault plane.
Think of it as the wall you could hang a lantern on if the fault were a tunnel.
In a normal fault, the hanging wall moves downward relative to the footwall.
The Footwall
The footwall is the block of rock that lies below the fault plane.
It's the wall you could walk on – the floor of the tunnel.
In a normal fault, the footwall moves upward relative to the hanging wall, or stays put as the hanging wall block slides down.
So, normal faults are called normal because the hanging wall moves down.
Extensional Forces: Pulling the Earth Apart
What causes this downward slide?
The answer is extensional forces, also known as tension.
These forces act to stretch and thin the Earth's crust.
Think of pulling on a rubber band. As you pull, the rubber band becomes thinner.
Eventually, if you pull hard enough, it will snap.
In the Earth's crust, extensional forces similarly stretch the rock, creating zones of weakness.
When the stress exceeds the strength of the rock, a fault forms, allowing the crust to relieve that tension through movement.
These forces are not random; they are intrinsically linked to the grand dance of plate tectonics.
The Magnificent Rift Valley: Where the Earth Sinks
Having explored the forces shaping the Earth's crust, we now venture into the realm of rift valleys – dramatic landscapes born from the very act of the Earth tearing itself apart. These valleys aren't just scenic wonders; they're living laboratories where geological processes are on full display.
Imagine a slice of Earth's crust caught in a cosmic tug-of-war, pulled in opposite directions.
The result? A spectacular sinking, a grand subsidence that sculpts vast depressions across continents.
These are rift valleys, and their story is one of geological drama played out on a colossal scale.
The Mechanics of Subsidence: A Sinking Act
The key to understanding rift valleys lies in the behavior of parallel normal faults.
Picture two (or more!) cracks forming in the ground, running roughly alongside each other.
Now, imagine the land between those cracks giving way, dropping downwards.
This is the essence of rift valley formation: the ground subsides between parallel normal faults.
It's like the floor dropping out from beneath you, creating a valley where there once was solid ground.
Another good visual is the trapdoor effect; think of two hinges on either side of a door.
The "door" is the rift valley block.
As the hinges give way (the parallel faults), the door swings downward, creating the sunken valley.
Examples
Rift valleys aren't just theoretical constructs; they're real-world features that dominate landscapes across the globe.
Here are some amazing examples.
The East African Rift System: A Continent in the Making
Perhaps the most iconic example is the East African Rift System, a colossal geological scar stretching thousands of kilometers across eastern Africa.
This isn't a single valley, but a complex network of rift valleys, volcanic mountains, and shimmering lakes.
It's an active zone of ongoing rifting, where the African continent is slowly being pulled apart.
This is actively widening, as the Somalian plate and African plate slowly separate.
The geological processes that are occurring here are very exciting.
The Basin and Range Province: A Fault-Carved Landscape
Across the Atlantic, the Basin and Range Province in the western United States offers another stunning example of rift valley formation.
Here, a series of roughly parallel normal faults has created a landscape of alternating mountain ranges (the "ranges") and valleys (the "basins").
The basins are grabens, the down-dropped blocks between the faults, while the ranges are horsts, the uplifted blocks.
It’s a testament to the power of extensional forces to sculpt vast landscapes over millions of years.
The Rhine Graben: A European Landmark
Europe also boasts its share of rift valleys, with the Rhine Graben being a prominent example.
This valley stretches through Germany, France, and Switzerland, marking a zone of geological weakness in the heart of Europe.
The Rhine River itself flows through the Graben, utilizing the sunken topography.
It's a reminder that even in seemingly stable continental interiors, the Earth's crust is still subject to the forces of rifting and faulting.
Horst and Graben: The Building Blocks of Rift Valleys
Having journeyed through the mechanics of rift valley formation, we now turn our attention to the distinctive landforms that define these dramatic landscapes. Think of rift valleys as sprawling stages, and horst and graben as the key players, each acting out its role in the grand geological drama.
Understanding these terms is crucial to deciphering the story etched into the Earth's surface. These features aren't merely incidental; they are fundamental components, the very building blocks that give rift valleys their characteristic appearance.
Defining the Players: Horst and Graben
Let's start with horst. Imagine a section of land stubbornly resisting the downward pull, standing tall between two sinking blocks. This uplifted block, bounded by normal faults on either side, is a horst. It represents the ground that didn't give way, a testament to the uneven distribution of stress within the Earth's crust.
Now, picture the opposite: a block of land that did succumb to the extensional forces, dropping down between two parallel faults. This down-dropped block is a graben. The Rhine Graben is a good example. The term comes from German, meaning ditch or grave.
Graben are the valleys in the mountain-valley landscape.
These are often the sites of lakes, rivers, and human settlement, drawn to the lower elevation and often fertile soils.
The Topography of Tension: A Landscape of Alternation
The interplay between horsts and grabens is what creates the distinctive topography of rift valleys. It's a landscape of striking contrasts, an alternation of elevated ridges and sunken valleys that stretches across vast distances.
This "horst and graben topography" is like a geological fingerprint, a telltale sign of the extensional forces that have shaped the land.
Think of the Basin and Range Province in the western United States. Here, the repeated pattern of north-south trending mountain ranges (horsts) and valleys (grabens) extends for hundreds of kilometers, a monumental testament to the power of faulting.
In essence, the horsts form the "ranges" and the grabens form the "basins," creating a visual rhythm across the landscape.
More Than Just Geology: A Home for Life
It's easy to get caught up in the geological details, but it's important to remember that these landscapes are more than just rocks and faults. They are dynamic environments that support a diverse array of life.
The valleys, or grabens, often become natural conduits for water, forming rivers and lakes. These water sources, in turn, attract wildlife and provide essential resources for human communities.
Horsts can create unique microclimates, providing refuge for specialized plant and animal species.
So, the next time you encounter a rift valley, take a moment to appreciate the complex interplay of geological forces and ecological processes that have shaped this remarkable landscape. Look for the horsts standing tall and the grabens cradling life, and recognize them as the essential building blocks of a truly magnificent natural phenomenon.
Anatomy of a Fault: Decoding Earth's Fractures
Having explored the grand scale of rift valleys and their characteristic horst and graben topography, it's time to zoom in and examine the intricate anatomy of a fault.
Understanding the individual components of a fault – the footwall, hanging wall, and the relentless forces of uplift and erosion – is essential for truly grasping how these geological structures shape our world.
It's like understanding the individual brushstrokes that compose a masterpiece: only then can we appreciate the full complexity and artistry of the work.
The Foundation: Footwall and Hanging Wall Defined
At the heart of every fault lies the fault plane, the surface along which movement occurs.
Imagine slicing through a cake at an angle. The two resulting pieces represent the two sides of a fault.
The footwall is the block of rock below the fault plane. It's the sturdy foundation, the ground beneath our feet (literally!).
The hanging wall, conversely, is the block above the fault plane.
Think of it as the section of rock that seems to "hang" over the fault.
In a normal fault, which we've been discussing in the context of rift valleys and fault-block mountains, the hanging wall moves down relative to the footwall.
This movement is driven by extensional forces, as we've already established.
A simple diagram illustrating these relationships is invaluable for understanding the fundamental geometry of a fault.
Uplift: The Earth Reaching for the Sky
Uplift is the vertical rise of land relative to a base level (often sea level). It's a fundamental process in mountain building and landscape evolution.
Uplift can occur due to a variety of forces, including tectonic activity (like faulting and folding), magmatic activity (volcanoes), and even the removal of overlying material (erosion).
In the context of fault-block mountains, uplift is often associated with the tilting of fault blocks. As one side of a block rises along a fault, the entire block is uplifted, creating a mountain range.
It's important to note that uplift and erosion often work in tandem.
As land is uplifted, it becomes more susceptible to erosion, which can then shape the landscape into its final form.
Erosion: Sculpting the Land, Grain by Grain
Erosion is the gradual wearing away of rock and soil by natural agents such as wind, water, and ice.
It's a powerful force that can transform mountains into rolling hills and carve deep canyons into the Earth's surface.
Erosion plays a crucial role in shaping fault-block mountains.
As uplift occurs, the exposed rock is subjected to weathering and erosion, which gradually break down the rock and transport the resulting sediment.
This process can create dramatic features such as steep cliffs, V-shaped valleys, and alluvial fans.
The rate of erosion is influenced by a variety of factors, including climate, rock type, and slope angle.
In arid regions, for example, wind erosion can be particularly effective, while in humid regions, water erosion dominates.
Glacial erosion, with its grinding power, can dramatically sculpt high-altitude landscapes.
A Dynamic Duo: Uplift and Erosion in Constant Competition
Uplift and erosion are often described as being in a constant tug-of-war.
Uplift builds up the land, while erosion tears it down.
The resulting landscape is a reflection of the balance between these two opposing forces.
In areas where uplift is dominant, mountains tend to be high and rugged.
In areas where erosion is dominant, the landscape tends to be low and subdued.
The interplay between uplift and erosion is what gives rise to the dynamic and ever-changing landscapes that we see around us. Understanding these processes is essential for deciphering the geological history of a region and predicting how it will evolve in the future.
Plate Tectonics and Continental Breakup: The Big Picture
Having delved into the mechanics of faulting and the formation of rift valleys, it’s time to zoom out and consider the grand orchestrator behind these dramatic geological events: plate tectonics.
Plate tectonics isn't just a theory; it's the fundamental framework for understanding the Earth's dynamic surface, the driving force behind mountain building, volcanic eruptions, and, crucially, the faulting that creates the landscapes we've been exploring.
Plate Tectonics: The Engine of Change
The Earth's lithosphere, its rigid outer shell, isn't a single, unbroken piece.
Instead, it's fractured into a series of tectonic plates that are constantly in motion, albeit at a glacial pace.
These plates interact with each other at their boundaries, giving rise to a variety of geological phenomena.
It's at these boundaries that the story of fault-block mountains truly begins to unfold.
Divergent Plate Boundaries: Where Continents Tear Apart
One of the most important types of plate boundary for our discussion is the divergent plate boundary.
This is where two plates are moving away from each other, driven by forces deep within the Earth's mantle.
As plates separate, the crust is stretched and thinned, creating a zone of extensional stress.
This extensional stress is precisely the force that leads to the formation of normal faults, the very building blocks of rift valleys and fault-block mountains.
These divergent boundaries are essentially zones of creation, giving birth to new crustal material as magma rises from the mantle to fill the void left by the separating plates.
Continental Rifting: A Continent's Identity Crisis
When divergent plate boundaries occur within a continent, the process is known as continental rifting.
Think of it as a continent undergoing a slow, agonizing split.
The classic example of this is the East African Rift System, a vast network of valleys, volcanoes, and faults stretching for thousands of kilometers across eastern Africa.
This is a living laboratory, a place where we can witness firsthand the processes that eventually lead to the formation of new oceans.
As the continent is pulled apart, a series of parallel normal faults develop, creating the characteristic horst and graben topography that we discussed earlier.
Over millions of years, the rift valley may widen and deepen, eventually filling with water to form a new ocean basin.
The Red Sea is a prime example of a rift valley that has progressed to this stage, representing an embryonic ocean basin steadily widening over geological timescales.
Crustal Thinning and Uplift: A Balancing Act
A crucial aspect of continental rifting is crustal thinning.
As the continent is stretched, the crust becomes thinner and less dense.
This thinning has a profound effect on the underlying mantle, allowing it to rise closer to the surface.
The rising mantle is hotter and less dense than the surrounding material, which in turn leads to uplift of the rift valley margins.
This uplift can contribute to the formation of fault-block mountains along the edges of the rift, adding another layer of complexity to the landscape.
This intricate interplay between crustal thinning and uplift is essential to fully understanding the formation of these tectonic wonders.
Fault-Block Mountains: Majestic Examples of Extensional Tectonics
Having explored the mechanics of faulting and the grand scale of plate tectonics, let's now journey to some real-world examples that showcase the sheer power and beauty of extensional forces: fault-block mountains.
These tilted giants stand as testaments to the Earth's dynamic processes, landscapes sculpted by millions of years of tectonic activity. Each mountain range tells a unique story of rifting, faulting, and the relentless shaping power of nature.
Sierra Nevada: A Classic Example of a Fault-Block Mountain Range
The Sierra Nevada in California is arguably one of the most iconic fault-block mountain ranges on Earth.
Its dramatic eastern escarpment, rising abruptly from the Owens Valley, is a textbook example of a fault scarp, a direct expression of the normal faulting that uplifted the range.
The range's formation began millions of years ago, as extensional forces stretched and thinned the Earth's crust in the Basin and Range Province.
As the land was pulled apart, a major fault system developed along the eastern edge of what is now the Sierra Nevada.
The western slope of the Sierra Nevada, in contrast to its steep eastern face, gradually slopes towards California's Central Valley.
This asymmetry is a hallmark of fault-block mountains, reflecting the tilted nature of the crustal block.
Erosion has further sculpted the landscape, carving deep canyons, polishing granite domes, and creating the breathtaking scenery of Yosemite National Park.
The Sierra Nevada's unique geology not only shapes its dramatic landscape but also influences its ecology, climate, and water resources, making it a vital part of California's identity.
The Wasatch Range: Tectonic Activity in the Heart of the American West
Stretching for hundreds of miles across Utah, the Wasatch Range is another superb example of a fault-block mountain range, formed by the same extensional forces that shaped the Basin and Range Province.
The range's dramatic western front, rising sharply above the Salt Lake Valley, is a prominent fault scarp, a testament to the ongoing tectonic activity in the region.
The Wasatch Fault, which runs along the base of the range, is one of the longest and most active normal faults in North America.
This fault is responsible for the uplift of the Wasatch Mountains and the subsidence of the Salt Lake Valley, creating the dramatic topography we see today.
The Wasatch Range is not just a geological wonder; it's also a vital source of water for the arid American West.
Snowmelt from the mountains feeds numerous rivers and streams, providing essential water for agriculture, industry, and urban populations.
The interplay between tectonic activity, erosion, and climate has created a diverse and dynamic landscape, making the Wasatch Range a fascinating place to study the Earth's processes.
The Harz Mountains: A German Fault-Block Landscape
Venturing across the Atlantic to Europe, the Harz Mountains in Germany offer a glimpse of fault-block tectonics in a different geological context.
Unlike the Sierra Nevada and Wasatch Range, which are relatively young mountain ranges, the Harz Mountains have a more complex and ancient history.
They are the northernmost horst block in Germany, elevated during the Cenozoic Era.
The Harz Mountains experienced significant uplift and faulting during the breakup of Pangaea, the supercontinent that existed millions of years ago.
These geological events created a distinct fault-block structure, with steep escarpments and valleys carved by erosion.
The Harz Mountains are not as towering as the Sierra Nevada or Wasatch Range, but their geological history and unique landscape make them a valuable example of fault-block mountain formation.
Furthermore, they have also been a source of heavy metal deposits. This created a need for water to be channeled for use in ore processing, which consequently, resulted in the creation of various artificial ponds, channels and ditches.
These examples, spanning continents and geological timescales, underscore the universal nature of fault-block mountain formation. Extensional tectonics, the driving force behind these landscapes, is a fundamental process shaping our planet. These mountains, standing tall against the sky, invite us to witness and appreciate the awesome power of geological forces.
The Role of Isostasy: Finding Balance in the Earth's Crust
Having seen how faults shape landscapes and how plate tectonics sets the stage, let's delve into a more subtle but equally powerful force: isostasy.
This principle acts as a kind of geological balancing act, governing the vertical movements of the Earth's crust.
Isostasy, often described as gravitational equilibrium, plays a vital role in understanding uplift and subsidence related to faulting and rifting.
It's the unseen hand that fine-tunes the landscape, responding to changes in weight and density.
The Principle of Buoyancy: A Floating Crust
Imagine icebergs floating in the ocean. The larger the iceberg, the deeper it sinks, yet all remain afloat.
This analogy captures the essence of isostasy.
The Earth's crust, like an iceberg, "floats" on the denser mantle below.
The principle dictates that areas of thicker or less dense crust will rise higher than areas of thinner or denser crust.
This phenomenon allows the Earth’s lithosphere to achieve gravitational balance with the asthenosphere.
Isostatic Rebound: Rising from the Depths
One of the most dramatic demonstrations of isostasy is isostatic rebound.
Think of a large glacier sitting on the land for thousands of years.
Its immense weight presses down the crust.
When the glacier melts, the land, now relieved of this burden, slowly rises back up.
This isostatic rebound is still occurring in regions that were once covered by ice sheets, such as Scandinavia and Canada.
The crust is essentially "bouncing back" after being compressed for millennia.
Erosion, Sedimentation, and Isostatic Adjustment
Isostasy isn't just about ice.
Erosion and sedimentation also play a crucial role.
When mountains erode, the reduced weight causes the crust to uplift, bringing deeply buried rocks to the surface.
Conversely, when sediments accumulate in a basin, the added weight causes the crust to subside.
These processes create a constant interplay between erosion, sedimentation, and isostatic adjustment, constantly reshaping the Earth's surface.
Isostasy and Fault-Block Mountains
So, how does isostasy relate to fault-block mountains?
As faulting creates mountains and valleys, the weight distribution changes.
Uplifted mountain blocks, becoming lighter through erosion, experience further uplift due to isostatic rebound.
Simultaneously, down-dropped valley blocks, accumulating sediments, subside further under the added weight.
This interplay between faulting and isostasy amplifies the topographic relief, creating the dramatic landscapes we associate with fault-block mountains.
The result is an ongoing cycle of uplift, erosion, and isostatic adjustment.
Isostasy, though a subtle force, is a critical component of the Earth's dynamic processes.
It's the unseen hand that balances the crust, responding to changes in weight distribution caused by faulting, erosion, sedimentation, and even the melting of glaciers.
Understanding isostasy is essential for comprehending the long-term evolution of landscapes and the majestic presence of geological formations like fault-block mountains.
Video: How Are Fault Block Mountains Formed? Tilted Giants
FAQs: Fault Block Mountains
What specific type of fault creates fault block mountains?
Normal faults are responsible. These faults occur when tensional forces in the Earth's crust cause one block of rock to slide downwards relative to another. This movement is crucial for how are fault block mountains formed.
How are fault block mountains formed in a series?
Often, several parallel normal faults develop. As the land between these faults drops, the adjacent blocks are uplifted to create mountain ranges. Erosion then shapes these uplifted blocks.
What's the 'tilted' aspect of these mountains?
Fault block mountains typically have one steep, faulted side and a more gently sloping opposite side. This tilted appearance results from the downward sliding along the normal fault, tilting the entire block. This is a key feature of how are fault block mountains formed.
What geological forces usually cause this type of faulting?
Tensional forces, or pulling forces, are the primary cause. These forces can be associated with plate tectonics, such as rifting or extension of the Earth's crust. These extensional forces are what ultimately leads to how are fault block mountains formed.
So, next time you're admiring those majestic, tilted giants, remember the incredible forces at play. It's all about the Earth's crust stretching and cracking, with some blocks dropping down while others rise up along those faults – that's how are fault block mountains formed! Pretty cool, right?