Fault Block Mountains: Definition, Features, & Formation
Fault block mountains, dramatic expressions of the Earth's tectonic activity, stand as testaments to the powerful forces shaping our planet's surface; geologists classify these formations based on their unique structural characteristics, which arise from the displacement of large crustal blocks along fault lines; the Basin and Range Province in the western United States exemplifies this process on a grand scale, showcasing the quintessential landscape of parallel mountain ranges separated by valleys; understanding the definition of fault block mountains requires appreciating that these elevated landforms are fundamentally a consequence of tensional forces and the subsequent fracturing of the lithosphere.
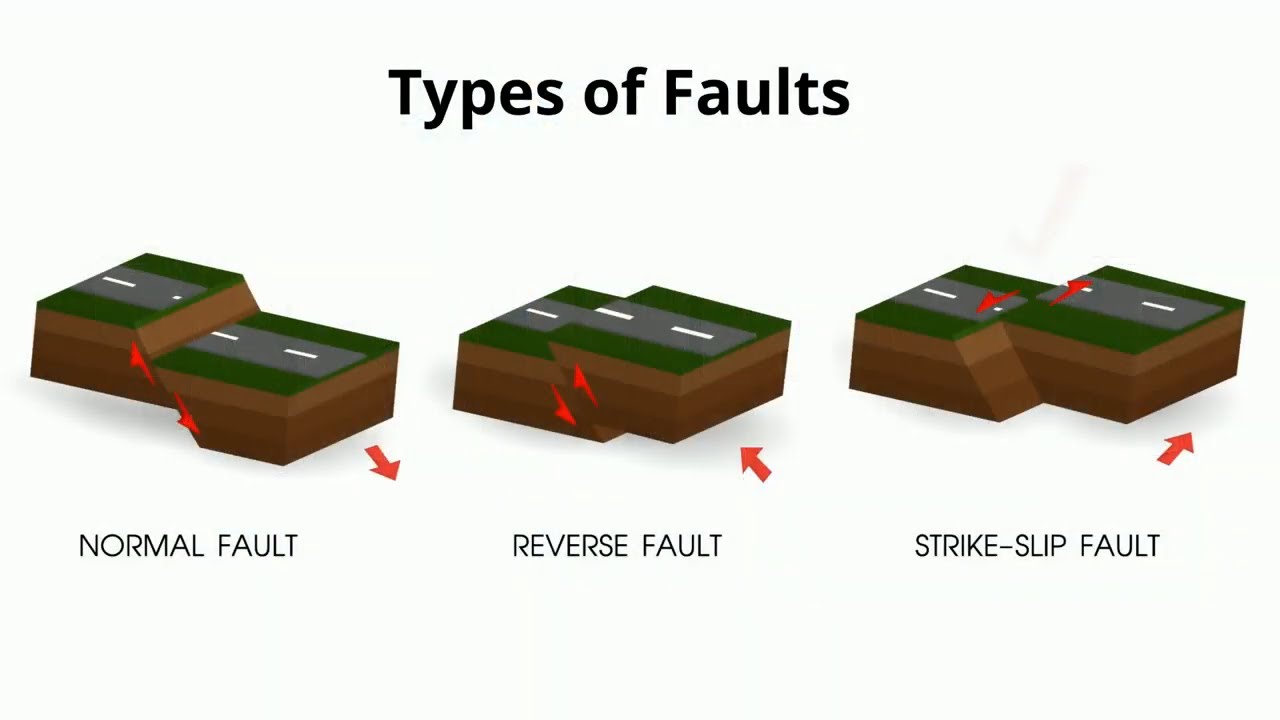
Image taken from the YouTube channel MooMooMath and Science , from the video titled Types of Faults in Geology .
Unveiling the Majesty of Fault Block Mountains
Fault block mountains represent a compelling testament to the Earth's restless nature.
These imposing geological features, unlike their folded counterparts, are not sculpted by compressional forces.
Instead, they are directly born from the fracturing and uplift of the Earth's crust.
Defining these landforms is crucial to understanding their origin.
Defining Fault Block Mountains
Fault block mountains are uplifted crustal blocks meticulously defined and bordered by faults.
These faults act as the geological chisels, carving out distinct mountain ranges and valleys.
The most common type of fault associated with these mountains are normal faults.
The Genesis: Tensional Forces and Faulting
The formation of fault block mountains is an elegant dance between stress and strain.
It begins with tensional forces acting upon the Earth's crust.
Imagine the crust being pulled apart; this is precisely the scenario that leads to their creation.
As the crust stretches, it inevitably fractures, leading to the development of faults.
The interplay between tensional forces and faulting is the engine driving their uplift.
The Landscape: Horsts and Grabens
Fault block mountain landscapes are not monolithic; they are complex tapestries of elevation.
These landscapes are characterized by a distinctive duo: Horsts and Grabens.
Horsts are the uplifted blocks that form the mountain ranges themselves.
Grabens, conversely, are the down-dropped blocks that create the adjacent valleys.
These features are a direct consequence of faulting, creating a visually striking and geologically significant pattern.
Fault Block Mountains Formation: A Landscape Shaped by Tension
The geological processes behind their formation are intrinsically linked to broader tectonic activity.
This link emphasizes their role as key indicators of regional stress regimes within the Earth’s crust.
Fault block mountains are formed by tensional forces acting on the Earth's crust, specifically through the process of faulting. This process is intrinsically linked to broader extensional tectonics, resulting in characteristic landforms such as Horsts and Grabens.
This statement encapsulates the core mechanism of their origin, underscoring the fundamental role of tension and faulting in shaping these majestic landforms.
The Engine of Creation: Faulting and Normal Faults
The towering presence of fault block mountains hints at the powerful forces at play beneath the Earth's surface. While erosion and weathering subtly reshape these landforms over eons, the primary architect of their existence is a far more dramatic process: faulting. It is this fundamental geological mechanism that fractures and displaces the Earth's crust, setting the stage for the uplift and creation of these imposing mountain ranges.
Understanding Faulting: The Foundation of Fault Block Mountains
At its core, faulting is the process of fracturing and displacement within the Earth's crust. It occurs when rocks are subjected to stresses that exceed their ability to withstand deformation. Unlike folding, which involves bending and warping of rock layers, faulting results in a distinct break or fracture plane along which movement occurs.
This movement can be vertical, horizontal, or a combination of both, depending on the orientation of the fault plane and the nature of the stresses involved.
Faulting is not merely a cosmetic alteration of the Earth's crust; it is the fundamental mechanism responsible for the very existence of fault block mountains. Without faulting, the stresses within the crust would likely be accommodated through other forms of deformation.
The Central Role of Faulting
Faulting plays the primary role in the creation of fault block mountains. The Earth's crust is subjected to tremendous forces, and when these forces become too great, the rock fractures and faults form.
These faults act as pathways for the movement of large blocks of crust. Some blocks are uplifted, forming the mountains themselves, while others subside, creating valleys or basins between them. This differential movement is what defines the characteristic landscape of fault block mountain regions.
Normal Faults: The Key to Uplift
While various types of faults exist, normal faults are most intimately associated with the formation of fault block mountains. Normal faults are characterized by a specific type of movement: the hanging wall (the block of rock above the fault plane) moves downward relative to the footwall (the block of rock below the fault plane).
This movement is typically driven by tensional forces, which stretch and thin the Earth's crust.
The downward movement of the hanging wall along a normal fault effectively creates a void, which is then filled by the upward movement of the footwall.
This process of repeated faulting and uplift results in the gradual elevation of the footwall block, eventually forming a fault block mountain.
Visualizing the Mechanics: The Importance of Diagrams
Understanding the relationship between faulting, normal faults, and the creation of fault block mountains is greatly enhanced through visual aids. Diagrams illustrating the movement of the hanging wall and footwall along a normal fault provide a clear and intuitive understanding of the process.
These diagrams can also depict the formation of Horsts and Grabens, the characteristic uplifted and down-dropped blocks that define the landscape of fault block mountain regions. By visualizing these geological processes, we can better appreciate the dynamic forces that shape our planet.
Tensional Forces and Extensional Tectonics: Stretching the Earth's Crust
The Engine of Creation: Faulting and Normal Faults The towering presence of fault block mountains hints at the powerful forces at play beneath the Earth's surface. While erosion and weathering subtly reshape these landforms over eons, the primary architect of their existence is a far more dramatic process: faulting. It is this fundamental geological process of faulting, in turn, that is driven by yet another underlying force: Tension.
Tension: The Prime Mover of Normal Faults
Tension is the geological force that pulls the Earth's crust apart. It is the antithesis of compression. This force is the primary driver behind the development of normal faults, the very faults that define fault block mountains.
When subjected to tension, rocks within the crust experience stress that can exceed their breaking point. This results in fracturing.
Crustal Thinning: A Consequence of Tension
As tension stretches the crust, it inevitably leads to thinning. Imagine pulling on a piece of dough; it becomes thinner as it is stretched. Similarly, the Earth's crust responds to tension by elongating and decreasing in thickness.
This thinning is not uniform. It tends to be concentrated along zones of weakness, often pre-existing faults or areas of higher heat flow.
Extensional Tectonics: A Regional Perspective
Tension and the resulting faulting are rarely localized phenomena. They are typically part of a much larger regional geological process known as extensional tectonics.
Extensional tectonics involves the widespread stretching and thinning of large portions of the Earth's lithosphere. It is a force that reshapes entire continents and ocean basins.
This regional extension can be caused by various factors, including mantle upwelling, gravitational collapse of mountain ranges, and changes in plate boundary forces.
Rifting: The Birth of New Boundaries
One of the most dramatic manifestations of extensional tectonics is rifting. Rifting is the process by which the Earth's crust and lithosphere are pulled apart, leading to the formation of new plate boundaries.
As the crust stretches and thins during rifting, it fractures along numerous normal faults. The central block between these faults often subsides, forming a rift valley.
Fault Block Mountains and Rift Valleys: A Symbiotic Relationship
Fault block mountains are intrinsically linked to rifting processes. As the crust is pulled apart, blocks of crust are uplifted along faults to form mountains, while the down-dropped blocks form rift valleys.
Often, fault block mountains are found flanking rift valleys, representing the elevated shoulders of the actively extending region. The East African Rift System, for example, is bordered by impressive fault block mountains that owe their existence to the ongoing rifting process.
Horsts and Grabens: The Building Blocks of the Landscape
Tensional forces wrenching at the Earth's crust do not simply create isolated mountains; they orchestrate a dynamic interplay of uplift and subsidence, crafting the very fabric of fault block landscapes. The resultant pattern of elevated and depressed blocks, known as Horsts and Grabens, represent the fundamental architectural elements that define these regions. These are not independent entities but rather complementary features born from the same tectonic processes.
The Interconnected Genesis of Horsts and Grabens
Horsts and Grabens are inextricably linked, their formation a direct consequence of normal faulting within an extensional regime. As the crust stretches, it fractures along multiple parallel faults. The central block that subsides between two such faults forms a Graben, a down-dropped valley or basin. Conversely, the adjacent blocks that remain elevated relative to the Graben become Horsts, forming ridges or mountains.
Imagine a series of dominoes, some falling (Grabens) while others remain standing (Horsts) – this analogy, though simplified, captures the essence of their concurrent development. It's crucial to understand that neither can exist without the other in this context. The creation of a Graben inevitably leads to the formation of bordering Horsts, and vice versa.
Defining the Landscape: More Than Just Mountains and Valleys
The significance of Horsts and Grabens extends beyond their mere presence as elevated and depressed landforms. They dictate the overall topography, drainage patterns, and even the ecological distribution within fault block mountain regions.
Topographical Dominance
The alternating pattern of Horsts and Grabens creates a distinctive “ribbed” landscape, characterized by parallel ridges and valleys. This structural grain influences everything from wind patterns to sunlight exposure, shaping microclimates and affecting vegetation zones.
Drainage Dynamics
Grabens often serve as natural drainage basins, collecting water and sediment from the surrounding Horsts. This leads to the formation of lakes, rivers, and alluvial plains within the Grabens, creating fertile areas amidst the otherwise rugged terrain. The deposition of sediments within Grabens further accentuates the relief, gradually burying the original fault scarps and creating broad, flat-bottomed valleys.
Ecological Impact
The contrasting environments of Horsts and Grabens support diverse ecosystems. Horsts, with their steeper slopes and thinner soils, tend to harbor drought-resistant vegetation and specialized animal life. Grabens, with their abundant water and nutrient-rich sediments, often support lush vegetation and a greater variety of species.
Visualizing the Structure: Diagrams and Real-World Examples
Understanding the spatial relationship between Horsts and Grabens is greatly aided by visual representation. Cross-sectional diagrams clearly illustrate the faulting geometry and the relative movement of the crustal blocks. These diagrams highlight the essential features: the steeply dipping normal faults, the uplifted Horsts, and the down-dropped Grabens.
Real-world examples provide tangible evidence of these features. The Basin and Range Province in the western United States offers a stunning showcase of numerous parallel Horsts and Grabens. Similarly, the Rhine Graben in Europe exemplifies a large-scale Graben structure bordered by the uplifted Vosges and Black Forest mountains, both acting as Horsts. These landscapes serve as natural laboratories, where the interplay of tectonics, erosion, and sedimentation has sculpted dramatic and informative geological features.
By recognizing the interconnected formation and defining influence of Horsts and Grabens, we gain a deeper appreciation for the complexity and beauty inherent in fault block mountain landscapes.
Crustal Extension, Rifting, and the Lithosphere: The Bigger Picture
Fault block mountains do not arise in isolation. To understand their formation, one must step back and observe the broader geological context – the grand tapestry of crustal extension, rifting, and the role of the lithosphere. These processes, intertwined and interdependent, provide the framework within which fault block mountains are sculpted.
Crustal Extension: The Initiating Force
At the heart of fault block mountain formation lies crustal extension. This is the stretching and thinning of the Earth’s crust, driven by tensional forces deep within the Earth. These forces are not applied uniformly.
Instead, they concentrate along zones of weakness, setting the stage for dramatic geological events. It's important to remember that this extension is not simply a surface phenomenon. It is a deep-seated process affecting the entire thickness of the Earth's crust.
Rifting: A Consequence of Extension
As crustal extension intensifies, it can lead to rifting. Rifting is the process where the Earth's lithosphere stretches and thins, often eventually splitting apart. This process results in the formation of rift valleys – elongated depressions bounded by normal faults.
These valleys are geological cradles where new crust can eventually be formed, given enough time and extension. Think of the East African Rift Valley, a prime example of an active rift zone where the African continent is slowly being pulled apart.
Rift Valleys and Fault Block Mountains: A Close Relationship
Fault block mountains frequently flank rift valleys. The same tensional forces that create the rift also generate the normal faults responsible for uplifting mountain blocks. This juxtaposition is not coincidental.
The presence of one often implies the existence of the other. The Rhine Graben in Europe, for instance, illustrates this relationship perfectly, with fault block mountains rising along its edges.
The Lithosphere: A Stage for Deformation
The lithosphere is the Earth's rigid outer layer, encompassing the crust and the uppermost part of the mantle. It is this layer that responds to tensional forces by fracturing and faulting.
The lithosphere, unlike the more ductile layers beneath it, cannot simply flow or deform plastically under stress. It breaks, creating the faults that define fault block mountains. The behavior of the lithosphere is critical to understanding the scale and style of faulting in a region.
In essence, fault block mountains are not isolated features but tangible expressions of deep-seated geological processes. Crustal extension, leading to rifting and deformation of the lithosphere, creates the conditions for their formation. Understanding these broader processes is key to deciphering the origin and evolution of these dramatic landscapes.
Escarpments and the Shaping Power of Erosion and Sedimentation
Fault block mountains do not emerge as static monuments. To understand the complete narrative of their existence, we must consider the dynamic interplay of erosion and sedimentation, forces that relentlessly sculpt these geological forms over vast stretches of time. The initial, stark architecture created by faulting is continuously refined, softened, and sometimes even obscured by these processes, resulting in landscapes that are as much a testament to weathering as they are to tectonic upheaval.
The Birth of an Escarpment: Instantaneous Creation
The immediate consequence of faulting is the creation of a fault scarp, or escarpment. This is the freshly exposed cliff face that marks the line of rupture where the Earth's crust has been vertically displaced.
The formation of an escarpment is an almost instantaneous event on geological timescales, a dramatic expression of the energy released during an earthquake. The sheer height and angle of the scarp reflect the magnitude of the faulting event, and its initial form provides a clear record of the tectonic forces at play.
Erosion: The Sculptor of Mountains
However, the sharp, angular appearance of a newly formed escarpment is ephemeral. Erosion, driven by wind, water, and ice, immediately begins to dismantle the scarp, gradually rounding its edges and reducing its overall height.
Weathering Processes
Weathering processes, both physical and chemical, weaken the rock, preparing it for removal. Freeze-thaw cycles crack the rock face, while chemical reactions dissolve susceptible minerals, undermining the structure.
Transportation of Material
The loosened material is then transported away by gravity, water, and wind, contributing to the gradual retreat of the escarpment and the widening of the adjacent valley. This erosion process not only softens the landscape, but also generates vast quantities of sediment.
Sedimentation: Filling the Grabens
The sediments produced by erosion do not simply vanish. Instead, they accumulate in the down-dropped grabens that lie adjacent to the uplifted horsts, creating valleys and basins. This process of sedimentation has a profound impact on the landscape, modifying its topography and influencing its ecological characteristics.
Valley Formation
Over time, the infilling of grabens with sediment can create broad, flat-bottomed valleys that contrast sharply with the steep, rugged slopes of the adjacent fault block mountains. These valleys often become focal points for human activity, as they provide fertile land for agriculture and offer relatively easy routes for transportation.
Altering Drainage Patterns
Sedimentation also alters drainage patterns, as streams and rivers are forced to adjust their courses to navigate the accumulating deposits. This can lead to the formation of alluvial fans, braided river systems, and other fluvial features that further contribute to the complexity of the landscape.
The Enduring Legacy: A Balanced Landscape
The interplay of erosion and sedimentation is an ongoing process, continuously reshaping fault block mountains over millions of years. While faulting creates the initial relief, erosion and sedimentation modify and redistribute the material, resulting in a dynamic equilibrium.
The final landscape is a complex mosaic of uplifted mountains, down-dropped valleys, and sediment-filled basins, a testament to the enduring power of both tectonic forces and surface processes. Understanding this interplay is crucial for interpreting the geological history of a region and for managing its natural resources in a sustainable manner.
Earthquakes: The Seismic Signature of Faulting
Fault block mountains are not silent witnesses to geological time; they are often seismically active regions. To fully grasp the dynamics of these landscapes, one must understand the intimate and inevitable relationship between faulting and earthquakes. Earthquakes are not merely coincidental occurrences in these areas; they are, in essence, the acoustic signatures of faulting itself.
The Inevitable Consequence: Fault Rupture and Seismic Waves
The connection is simple, yet profound: earthquakes are a direct result of faulting. The Earth's crust, under immense stress from tensional forces, eventually reaches a breaking point. When this threshold is crossed, the rock fractures along a fault line, and the two sides of the fault slip past each other.
This sudden release of stored energy propagates outwards in the form of seismic waves. These waves ripple through the Earth, causing the ground to shake – the phenomenon we experience as an earthquake. The severity of the earthquake is directly related to the amount of energy released during the fault rupture and the size of the fault area that slips.
Quantifying the Connection: Magnitude and Fault Displacement
The magnitude of an earthquake provides a quantitative measure of the energy released. A larger magnitude typically corresponds to a greater amount of fault displacement – the distance the two sides of the fault move relative to each other.
In fault block mountain regions, the dominant type of faulting is normal faulting. Normal faults are characterized by the hanging wall moving downwards relative to the footwall. During an earthquake along a normal fault, this downward movement can be quite dramatic, leading to significant ground deformation and potentially causing landslides and other geological hazards.
Seismic Monitoring: Unveiling Subsurface Dynamics
Seismic monitoring plays a vital role in understanding the dynamics of fault block mountain regions. By deploying networks of seismometers, scientists can detect and locate earthquakes, even those too small to be felt by humans.
The data gathered from these networks provides valuable insights into the geometry and behavior of faults beneath the surface. This can help to assess the potential for future earthquakes and to develop strategies for mitigating seismic risk.
The Broader Context: Earthquakes as a Window into Earth's Interior
Earthquakes are more than just destructive events. They are also powerful tools for studying the Earth's interior. Seismic waves generated by earthquakes travel through the Earth's layers, and their speed and direction are affected by the properties of the rocks they encounter.
By analyzing the patterns of seismic wave propagation, seismologists can infer the composition, density, and temperature of the Earth's mantle and core. In this way, earthquakes provide a unique window into the hidden depths of our planet. Understanding the seismic signature of fault block mountains not only helps us to assess local hazards but also contributes to a broader understanding of Earth's dynamic processes.
[Earthquakes: The Seismic Signature of Faulting Fault block mountains are not silent witnesses to geological time; they are often seismically active regions. To fully grasp the dynamics of these landscapes, one must understand the intimate and inevitable relationship between faulting and earthquakes. Earthquakes are not merely coincidental occurrences; they are direct consequences of the very forces that sculpt these dramatic terrains. Let us now pivot from the theoretical to the tangible, examining specific locales where the processes of fault block mountain formation are vividly displayed.]
Regional Examples: Case Studies in Fault Block Mountain Formation
The principles of fault block mountain formation, while elegantly explained in theory, find their most compelling validation in real-world examples. Across the globe, there are regions where the telltale signs of tensional forces, normal faulting, and crustal uplift are etched into the landscape with remarkable clarity. These areas serve as living laboratories, allowing geologists to refine their understanding of the Earth's dynamic processes.
The Basin and Range Province: A Testament to Extension
Perhaps the most iconic example of fault block mountain formation is the Basin and Range Province of western North America. Spanning across several states, this vast region is characterized by a distinctive topography of roughly parallel mountain ranges (the "Basins") separated by broad, sediment-filled valleys (the "Ranges").
This landscape is the direct result of prolonged crustal extension, where the Earth's lithosphere has been stretched and thinned over millions of years.
The tensional forces have caused extensive normal faulting, resulting in the uplift of mountain blocks (Horsts) and the subsidence of valley floors (Grabens).
The sheer scale of the Basin and Range, coupled with the ongoing seismic activity, makes it a prime location for studying the intricacies of fault block mountain formation.
Sierra Nevada: A Tilted Giant
The Sierra Nevada mountain range in California presents a slightly different, yet equally compelling, case study. Unlike the more symmetrical Basin and Range, the Sierra Nevada is a massive, tilted fault block. Its eastern face rises abruptly along a major fault system, creating a dramatic escarpment.
The western slope, in contrast, descends gradually towards the Central Valley. This asymmetry is a key indicator of its fault-block origin. The entire range has been uplifted along the eastern fault zone, while the western side has gently subsided.
The sheer scale and pronounced tilt of the Sierra Nevada underscore the immense power of tectonic forces in shaping mountain ranges.
The Harz Mountains: A European Example
While North America boasts some of the most prominent examples, fault block mountains are by no means limited to that continent. The Harz Mountains in Germany provide a fascinating European analogue.
This range, located in central Germany, exhibits the classic Horst and Graben structure, although on a smaller scale than the Basin and Range.
The Harz Mountains were formed during the late Paleozoic Era, and have been repeatedly reactivated by tectonic forces throughout their history. Their location within a complex geological setting highlights the widespread nature of fault block mountain formation.
Wasatch Range: Urban Tectonics
The Wasatch Range, towering over the Salt Lake Valley in Utah, presents a compelling example of a still-active fault block mountain range impacting a major urban area. The range is defined by the Wasatch Fault, one of the longest and most active normal faults in the world.
The fault's continued movement poses a significant seismic risk to the densely populated Wasatch Front.
The juxtaposition of a major city with an active fault block mountain range makes the Wasatch Range a critical area for geological research and hazard mitigation.
Rift Valley Edges: A Global Phenomenon
Fault block mountains frequently flank the edges of rift valleys, those dramatic linear depressions formed by the splitting apart of the Earth's crust. The East African Rift System, a prime example, is bordered by numerous fault block mountain ranges. As the rift valley widens due to extensional forces, the adjacent crust is uplifted along normal faults, creating towering escarpments and rugged mountain ranges.
The Rhine Graben in Europe also exhibits similar features, albeit on a smaller scale. The Black Forest and the Vosges Mountains, flanking the Rhine Valley, are classic examples of fault block mountains formed in association with rifting.
These examples demonstrate that the formation of fault block mountains is intimately linked to the process of continental rifting, a fundamental aspect of plate tectonics.
Secondary Features: Alluvial Fans and Death Valley
Fault block mountains, sculpted by the raw power of tectonic forces, do not exist in isolation. Their presence invariably shapes the surrounding landscape, giving rise to a suite of secondary features that offer further insights into the geological processes at play. Among the most prominent of these are alluvial fans, sprawling deposits of sediment that fan out at the base of the mountains, and the stark, dramatic expanse of Death Valley, a quintessential example of a Graben intricately linked to the broader Basin and Range Province.
Alluvial Fan Formation: A Story of Erosion and Deposition
The formation of alluvial fans is inextricably linked to the processes of erosion that relentlessly attack the uplifted fault blocks. As mountains rise, they are immediately subjected to weathering, the breakdown of rocks through physical and chemical processes. This fragmented material, ranging from massive boulders to fine silts, is then transported downslope by the relentless force of water.
During periods of intense rainfall or snowmelt, torrential flows carve channels through the steep mountain slopes, carrying vast quantities of sediment. As these streams emerge from the confined canyons onto the relatively flat plains at the mountain's base, their velocity abruptly decreases.
This sudden loss of energy causes the water to lose its capacity to carry its heavy load.
Sediment is subsequently deposited, with the coarsest materials dropping out first, followed by progressively finer particles.
Over countless cycles of erosion and deposition, this process builds up a characteristic fan-shaped accumulation of sediment – the alluvial fan. The fan's shape is a direct consequence of the stream's tendency to shift its course over time, creating a braided network of channels that spread out across the plain.
Death Valley: A Graben's Extreme Expression
Death Valley, located in the Basin and Range Province, exemplifies a graben taken to its extreme. It's a geological masterpiece carved by extensional tectonics, resulting in a deep, narrow depression flanked by towering fault block mountain ranges.
Tectonic Origins of Death Valley
The Basin and Range Province is characterized by widespread crustal extension, where the Earth's crust is being pulled apart. This extension results in the formation of numerous normal faults, causing some blocks of the crust to subside (Grabens) while others are uplifted (Horsts).
Death Valley represents one of the deepest and most dramatic Grabens within this province. The valley floor has subsided along major fault lines over millions of years, creating a profound depression that reaches the lowest elevation in North America.
Unique Characteristics
Beyond its tectonic origins, Death Valley is renowned for its extreme environment. The combination of its low elevation, narrow confines, and surrounding mountains creates a hyper-arid climate with scorching summer temperatures.
Rainfall is scarce, and evaporation rates are exceptionally high, contributing to the valley's desolate and otherworldly landscape. The valley floor is covered in vast salt flats, remnants of ancient lakes that once filled the depression.
These salt flats, combined with the rugged mountains and stark desert scenery, create a truly unique and unforgettable landscape.
A Window into Earth’s Processes
Alluvial fans and Death Valley, though distinct in their appearance and formation, represent tangible evidence of the powerful forces that shape our planet. Studying these secondary features enhances our comprehension of fault block mountain formation and the broader geological context in which they exist. They serve as a reminder that landscapes are not static entities but rather dynamic expressions of Earth's ongoing evolution.
Research and Analysis Techniques: Decoding the Landscape
Fault block mountains, sculpted by the raw power of tectonic forces, do not exist in isolation. Their presence invariably shapes the surrounding landscape, giving rise to a suite of secondary features that offer further insights into the geological processes at play. Among the most prominent of these is a suite of sophisticated investigative methods that allow geoscientists to reconstruct the intricate history and ongoing dynamics of these regions.
These techniques, ranging from traditional field mapping to cutting-edge remote sensing and geochronology, collectively provide a multifaceted perspective on the formation and evolution of fault block mountains.
The Foundation: Geological Mapping
Geological mapping remains the cornerstone of understanding fault block terrains. By meticulously documenting the distribution, orientation, and relationships of rock units, faults, and other structural features, geologists construct detailed maps that serve as the foundation for further analysis.
These maps reveal the structural architecture of the region, delineating the horsts and grabens that define the fault block landscape. The stratigraphy, or layering, of the rocks provides a temporal framework, allowing geologists to reconstruct the sequence of events that led to the mountain's formation.
Eyes in the Sky: Remote Sensing
Remote sensing techniques, including satellite imagery and LiDAR (Light Detection and Ranging), offer a bird's-eye view of fault block regions. Satellite imagery captures broad-scale patterns of landforms and vegetation, revealing the regional extent of fault systems and the overall geomorphology.
LiDAR, on the other hand, provides high-resolution topographic data, allowing for the precise measurement of fault scarps, alluvial fans, and other subtle features that may be difficult to discern from the ground. This data facilitates the creation of digital elevation models (DEMs), which are invaluable for analyzing drainage patterns and landform evolution.
Probing the Depths: Seismic Surveys
While surface mapping and remote sensing provide valuable insights, seismic surveys allow geologists to peer beneath the surface and image the subsurface structure of fault block regions. These surveys involve generating seismic waves that travel through the Earth and are reflected or refracted by subsurface layers.
By analyzing the arrival times and amplitudes of these waves, geophysicists can create detailed images of the subsurface, revealing the geometry of faults, the thickness of sedimentary layers, and the presence of hidden structures. Seismic data is particularly useful for understanding the deep roots of fault systems and the relationship between surface and subsurface deformation.
Tracking Movement: GPS and Geodetic Measurements
Fault block mountains are not static features; they are constantly evolving under the influence of tectonic forces. GPS (Global Positioning System) and other geodetic techniques provide a means of monitoring ongoing crustal deformation and fault movement.
By establishing a network of GPS stations across a fault block region, scientists can measure the precise positions of these stations over time. Any changes in position indicate movement along faults or deformation of the surrounding crust. This data is crucial for understanding the rate and style of deformation, as well as for assessing the potential for future earthquakes.
Dating the Past: Geochronology
Geochronology, the science of dating rocks and minerals, provides a temporal framework for understanding the evolution of fault block mountains. Radiometric dating techniques, such as uranium-lead dating and argon-argon dating, allow geologists to determine the age of faulting and uplift.
By dating different rock units and fault surfaces, geochronologists can reconstruct the history of fault activity and determine the rates of mountain building over millions of years. This information is essential for understanding the long-term evolution of fault block regions and their relationship to broader tectonic processes.
A Holistic Approach
In conclusion, decoding the landscape of fault block mountains requires a holistic approach that integrates data from a variety of sources. Geological mapping provides the foundation, remote sensing offers a broad perspective, seismic surveys probe the depths, GPS monitors ongoing movement, and geochronology provides a temporal framework. By combining these techniques, geoscientists can unravel the complex history and ongoing dynamics of these fascinating geological features.
The Influence of Isostasy: Gravitational Equilibrium and Uplift
Fault block mountains, sculpted by the raw power of tectonic forces, do not exist in isolation. Their presence invariably shapes the surrounding landscape, giving rise to a suite of secondary features that offer further insights into the geological processes at play. Among the most significant is the principle of isostasy, a fundamental concept in understanding the vertical movement and long-term stability of these imposing geological structures.
Isostasy Defined: A Balancing Act
Isostasy describes the state of gravitational equilibrium between Earth's lithosphere (crust and upper mantle) and the asthenosphere (the viscous, deformable upper mantle). It’s a concept analogous to buoyancy: less dense objects "float" higher on a denser medium.
In geological terms, thicker or less dense crust "floats" higher on the denser mantle below, while thinner or denser crust sinks deeper. This balance is not static but rather a dynamic process of adjustment.
Isostatic Rebound and Mountain Uplift
The formation of fault block mountains inherently disrupts isostatic equilibrium. The uplift of a mountain range represents a localized thickening of the crust. This thickening creates an imbalance, as the increased mass exerts greater pressure on the underlying mantle.
To restore equilibrium, the lithosphere responds through isostatic rebound, a slow, upward adjustment of the crust. This rebound effectively amplifies the initial tectonic uplift, contributing significantly to the overall height and prominence of the mountain range. The process continues until a new state of gravitational balance is achieved.
Erosion's Role: Unloading and Further Uplift
Erosion plays a crucial, albeit indirect, role in isostatic uplift. As mountains are weathered and eroded, vast quantities of material are removed from their peaks and slopes. This removal of mass "unloads" the crust, reducing the pressure on the underlying mantle.
In response, the crust rebounds upwards, further elevating the mountain range. This process creates a fascinating feedback loop: tectonic uplift creates mountains, erosion wears them down, and isostatic rebound counteracts the erosion by lifting them back up.
Isostatic Compensation: Rooted in the Mantle
The concept of isostatic compensation is intrinsically linked to the idea of a 'crustal root'. Think of an iceberg: a significant portion of its mass lies submerged beneath the water's surface. Similarly, mountain ranges possess a thickened crustal root that extends deep into the mantle.
This root provides the necessary buoyancy to support the elevated topography above. As erosion removes material from the mountain's surface, the root becomes relatively larger, causing the crust to rise in compensation, maintaining isostatic balance.
Regional Variations and Tectonic Setting
The extent to which isostasy influences the uplift of fault block mountains can vary significantly depending on the regional tectonic setting and the properties of the lithosphere. In regions with a strong, rigid lithosphere, isostatic adjustments may be slower and less pronounced.
Conversely, in areas with a weaker, more ductile lithosphere, isostatic rebound can be a more significant factor in mountain building. The interplay between tectonic forces and isostatic compensation ultimately determines the final form and elevation of these majestic landforms. Understanding isostatic principles provides critical insights into the dynamic processes shaping our planet's landscapes.
Video: Fault Block Mountains: Definition, Features, & Formation
FAQs: Fault Block Mountains
How are fault block mountains different from other mountain types?
Fault block mountains are formed by the movement of large crustal blocks along faults. Unlike folded mountains that result from compression, or volcanic mountains built by eruptions, fault block mountains arise from tensional forces that cause blocks of the Earth's crust to drop down relative to other blocks. This differing force contributes to the unique definition of fault block mountains.
What is the "fault" in fault block mountains?
The "fault" refers to a fracture or zone of fractures in the Earth's crust where rock masses have moved relative to one another. In the context of fault block mountains, these faults are typically normal faults, where one side (the hanging wall) has moved down relative to the other side (the footwall).
What are some common features of fault block mountains?
Fault block mountains often display a steep, linear slope on one side (the fault scarp) and a gentler slope on the other. They can also exhibit tilted blocks, grabens (down-dropped valleys between parallel faults), and horsts (uplifted blocks bounded by parallel faults). These features clearly define fault block mountains.
What tectonic forces are responsible for the formation of fault block mountains?
Tensional forces within the Earth's crust are primarily responsible. These forces stretch and thin the crust, leading to fracturing and faulting. This extension causes certain blocks of land to subside, forming valleys, while adjacent blocks are uplifted to create the definition of fault block mountains.
So, next time you're driving through a landscape with those sharply angled peaks and valleys, remember you're likely looking at the impressive handiwork of fault block mountains. These dramatic formations, born from the Earth's crust being stretched and broken into tilted blocks, are a powerful reminder of the dynamic forces shaping our planet.