Essential Amino Acids: Building Blocks of Proteins
Amino acids, vital organic compounds, serve as the building blocks of proteins, the molecules that perform a wide array of functions within living organisms. The synthesis of these complex molecules, crucial for muscle repair and overall growth, depends heavily on the availability of nine essential amino acids, some of which cannot be produced by the body and must be obtained through diet. Linus Pauling Institute, a world-renowned research organization, emphasizes the role of balanced nutrition in ensuring adequate intake of these building blocks. Mass spectrometry, a powerful analytical technique, plays an indispensable role in accurately identifying and quantifying amino acids, thereby contributing to studies on human nutrition and protein metabolism.
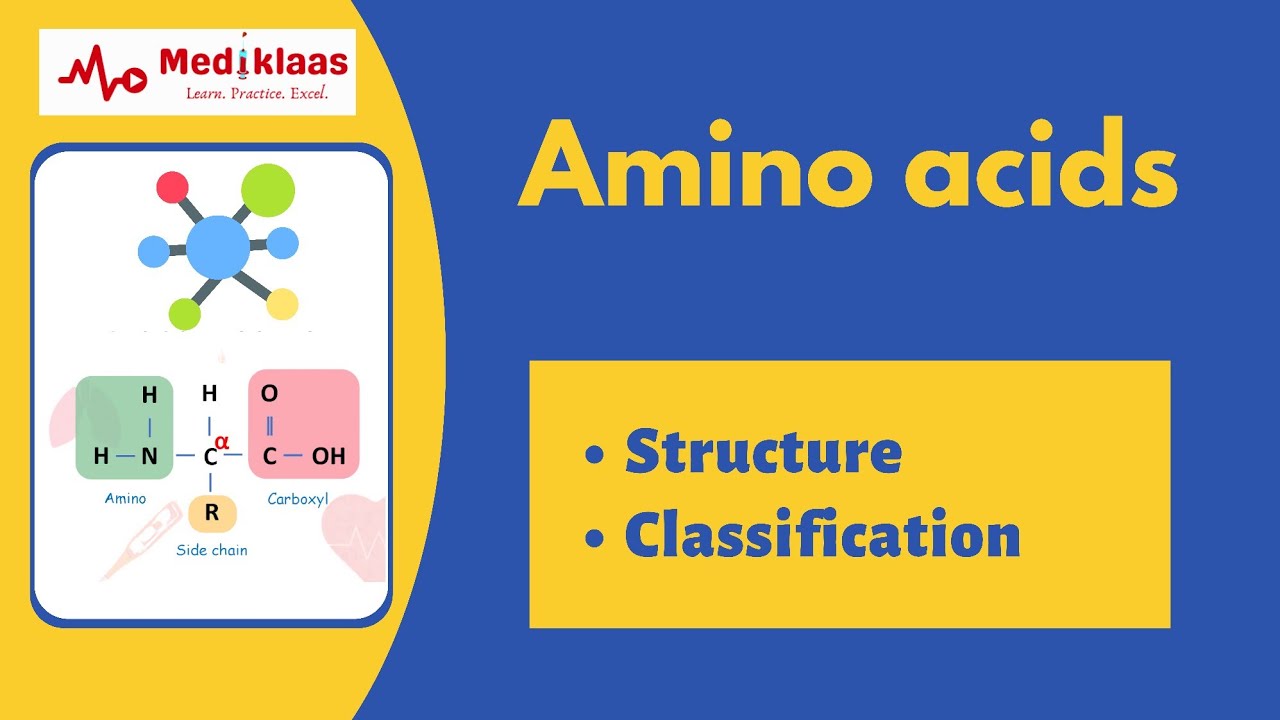
Image taken from the YouTube channel Mediklaas , from the video titled Amino acids - the building blocks of proteins .
Unveiling the Power of Proteins and Amino Acids
Proteins stand as fundamental macromolecules within all living organisms, performing a staggering array of functions essential to life. They are the workhorses of the cell, involved in virtually every biological process imaginable.
From catalyzing biochemical reactions to providing structural support, proteins are indispensable. Understanding their nature and function is paramount to comprehending the intricacies of life itself.
Proteins: The Building Blocks of Life
Proteins are complex polymers constructed from smaller subunits called amino acids. Think of amino acids as individual Lego bricks; when linked together in specific sequences, they form a diverse range of protein structures with unique properties and functions.
The order and type of amino acids determine a protein's shape and, consequently, its specific activity. This precise arrangement is crucial for proteins to execute their designated roles effectively.
Amino Acids: The Monomers of Protein Synthesis
Amino acids, therefore, are the basic building blocks of proteins. Each amino acid contains a central carbon atom bonded to an amino group (-NH2), a carboxyl group (-COOH), a hydrogen atom, and a unique side chain (R-group).
It is the variability in these R-groups that gives each of the 20 common amino acids its distinct chemical properties. These differences dictate how amino acids interact with each other and with other molecules, influencing the final three-dimensional structure of the protein.
The Interplay of Nutrition and Biochemistry
The study of proteins and amino acids is fundamentally intertwined with both nutrition and biochemistry. Nutrition explores how the body obtains and utilizes these essential components from food, while biochemistry delves into the molecular mechanisms underlying their synthesis, function, and degradation within cells.
The two disciplines complement each other, providing a holistic understanding of the protein lifecycle from dietary intake to its ultimate impact on cellular processes.
The Multifaceted Roles of Proteins
Proteins fulfill an astonishingly broad spectrum of functions within the body. They serve as enzymes, catalyzing biochemical reactions with remarkable specificity and efficiency.
They act as structural components, providing support and shape to cells and tissues. Proteins also function as hormones, transmitting signals between cells and regulating physiological processes.
Antibodies, which are proteins, defend the body against foreign invaders. Transport proteins carry molecules throughout the body, and contractile proteins enable muscle movement.
The diversity in protein function underscores their vital importance in maintaining overall health and well-being. From the smallest cellular process to complex physiological functions, proteins are central players in the drama of life.
Amino Acid Anatomy: Structure, Classification, and Significance
Proteins stand as fundamental macromolecules within all living organisms, performing a staggering array of functions essential to life. They are the workhorses of the cell, involved in virtually every biological process imaginable. Understanding the fundamental building blocks of these proteins, the amino acids, is crucial to grasping their overall function.
The Basic Blueprint: Amino Acid Structure
At the heart of every amino acid lies a conserved structure: a central carbon atom (the α-carbon) bound to four distinct groups.
These include an amino group (-NH₂), a carboxyl group (-COOH), a hydrogen atom (-H), and a unique side chain, also known as the R-group.
It is this R-group that differentiates each of the 20 standard amino acids and imparts their unique chemical properties.
This seemingly simple variation is what gives rise to the incredible diversity of protein structure and function.
Essential vs. Non-Essential: A Nutritional Divide
Amino acids are categorized based on our bodies’ ability to synthesize them.
Those we can produce internally are termed non-essential.
On the other hand, essential amino acids cannot be synthesized de novo or in sufficient quantities by the human body and must be obtained through our diet.
This dietary imperative underscores the critical role of nutrition in maintaining optimal health and supporting essential biological processes.
The Nine Essentials: A Dietary Cornerstone
There are nine essential amino acids that the body cannot produce:
- Histidine
- Isoleucine
- Leucine
- Lysine
- Methionine
- Phenylalanine
- Threonine
- Tryptophan
- Valine
These amino acids are the cornerstone of a healthy diet.
A deficiency in any one of them can significantly impair protein synthesis and lead to various health problems.
Consuming a balanced diet rich in diverse protein sources is key to ensuring an adequate intake of all nine.
The Limiting Factor: Impact on Protein Synthesis
The concept of a limiting amino acid is crucial in understanding protein synthesis.
If one essential amino acid is present in insufficient amounts, it can limit the rate at which the body can build proteins, even if all other amino acids are available in abundance.
Imagine a construction crew building a house. If they run out of bricks (the limiting amino acid), they can't complete the walls, even if they have plenty of wood, nails, and other materials.
This highlights the importance of consuming complete proteins, which contain all nine essential amino acids in adequate amounts.
Alternatively, strategically combining incomplete proteins from various sources can ensure that all essential amino acid needs are met.
Building Blocks to Masterpieces: Protein Synthesis and Structure
[Amino Acid Anatomy: Structure, Classification, and Significance Proteins stand as fundamental macromolecules within all living organisms, performing a staggering array of functions essential to life. They are the workhorses of the cell, involved in virtually every biological process imaginable. Understanding the fundamental building blocks of these...] We now move to appreciate how amino acids, once obtained or synthesized, are meticulously assembled into functional proteins. This section unravels the complex choreography of protein synthesis, the vital peptide bonds that hold it all together, and the hierarchical levels of protein structure, each contributing to its unique functionality.
The Symphony of Protein Synthesis
Protein synthesis, also known as translation, is the elegant process by which cells construct proteins from their constituent amino acids, according to the genetic blueprints encoded in DNA. It's a multi-step, highly regulated process involving numerous cellular components.
This process is not a simple stringing together of amino acids. Instead, it is an intricate and orchestrated molecular dance involving several key players and distinct phases.
From DNA to mRNA: Transcription's Role
The journey from gene to protein begins with transcription, the process where the DNA sequence of a gene is copied to create a complementary RNA molecule, specifically messenger RNA (mRNA).
Consider mRNA as a crucial intermediate. It carries the genetic code from the nucleus, where DNA resides, to the ribosomes in the cytoplasm, the protein synthesis machinery.
The Ribosome's Stage: Translation Unveiled
Translation is the process where the mRNA sequence is decoded by ribosomes to assemble a specific amino acid chain, ultimately forming a polypeptide.
Ribosomes, complex molecular machines found in the cytoplasm, serve as the site of protein synthesis. Think of them as the assembly line.
The mRNA molecule threads through the ribosome. Transfer RNA (tRNA) molecules, each carrying a specific amino acid, recognize and bind to corresponding codons (three-nucleotide sequences) on the mRNA.
These codons are the blueprints, ensuring the amino acids are added in the precise order dictated by the gene.
The Peptide Bond: The Architect of Protein Chains
As each tRNA delivers its amino acid, a peptide bond forms between the carboxyl group of one amino acid and the amino group of the next.
This covalent bond, strong and stable, links the amino acids together, creating a growing polypeptide chain.
Imagine it as the mortar holding the bricks (amino acids) together in a wall (polypeptide).
Polypeptides: Chains of Amino Acid Precision
A polypeptide is simply a chain of amino acids linked by peptide bonds. These chains can vary greatly in length and amino acid composition.
The sequence of amino acids within a polypeptide is critical, dictating the protein's ultimate structure and function. It is like the order of letters in a word, determining its meaning.
Levels of Protein Structure: From String to Sculpture
Proteins exhibit a hierarchical organization of structure, progressing from the simple linear sequence of amino acids to complex three-dimensional arrangements.
These levels are critical for the function. They determine the protein's stability, interactions, and overall biological activity.
Primary Structure: The Amino Acid Sequence
The primary structure refers to the linear sequence of amino acids in a polypeptide chain.
This sequence is genetically determined and is fundamental to all higher levels of protein structure.
Any alteration in this sequence, even a single amino acid change, can drastically affect the protein's function.
Secondary Structure: Local Folding Patterns
Secondary structure arises from local folding patterns within the polypeptide chain, stabilized by hydrogen bonds between amino acids.
The two most common secondary structures are alpha helices and beta sheets. Alpha helices are coiled structures. Beta sheets are pleated or folded structures.
These patterns provide the initial architectural framework for protein folding.
Tertiary Structure: The Three-Dimensional Conformation
Tertiary structure describes the overall three-dimensional shape of a single polypeptide molecule.
This structure is determined by various interactions between amino acid side chains, including hydrophobic interactions, hydrogen bonds, ionic bonds, and disulfide bridges.
Tertiary structure is crucial for protein function, defining its active sites and binding pockets.
Quaternary Structure: Assembling the Multimers
Quaternary structure applies only to proteins composed of multiple polypeptide subunits.
It describes the arrangement and interactions of these subunits within the protein complex.
Hemoglobin, for example, consists of four subunits. Their coordinated action is essential for oxygen transport.
The Protein Journey: Digestion, Absorption, and Metabolism
After we consume protein-rich foods, a complex and carefully orchestrated series of events unfolds within the body to break down these large molecules into usable components. This process, involving digestion, absorption, and subsequent metabolic transformations, is critical for providing the amino acids needed for protein synthesis, energy production, and other vital functions. Understanding this "protein journey" is key to appreciating how our bodies utilize dietary protein.
Protein Digestion: Unraveling the Structure
Protein digestion begins in the stomach, where gastric chief cells release pepsinogen, an inactive zymogen of pepsin. Hydrochloric acid (HCl), secreted by parietal cells, denatures proteins, unfolding their complex three-dimensional structures. This denaturation makes the peptide bonds more accessible to enzymatic cleavage. HCl also converts pepsinogen into its active form, pepsin.
Pepsin, an endopeptidase, specifically cleaves peptide bonds adjacent to aromatic amino acids like phenylalanine, tyrosine, and tryptophan. This initial digestion yields smaller polypeptides, which then move into the small intestine for further processing.
In the small intestine, pancreatic enzymes play a crucial role. Trypsin, chymotrypsin, elastase, and carboxypeptidases are secreted as inactive proenzymes and activated in the intestinal lumen.
- Trypsin, activated by enteropeptidase, activates other proenzymes.
- Chymotrypsin cleaves peptide bonds near aromatic amino acids.
- Elastase hydrolyzes peptide bonds adjacent to small, nonpolar amino acids.
- Carboxypeptidases act as exopeptidases, removing amino acids from the carboxyl ends of the polypeptides.
The end result of this orchestrated enzymatic action is a mixture of free amino acids, dipeptides, and tripeptides that are ready for absorption.
Amino Acid Absorption: Entering the Bloodstream
The absorption of amino acids primarily occurs in the small intestine, specifically in the jejunum. Specialized transport systems located on the apical membrane of enterocytes facilitate the uptake of amino acids and small peptides. Several different transport systems exist, each with a specificity for certain groups of amino acids.
Amino acids are transported across the apical membrane via sodium-dependent and sodium-independent transporters. Sodium-dependent transporters utilize the electrochemical gradient of sodium to drive the uptake of amino acids.
Dipeptides and tripeptides are transported into the enterocytes via the PepT1 transporter, which is also proton-dependent. Once inside the enterocyte, dipeptidases and tripeptidases hydrolyze these small peptides into free amino acids.
From within the enterocytes, amino acids are then transported across the basolateral membrane into the bloodstream, again using specific transport systems. This allows them to be distributed throughout the body.
Protein Turnover: A Dynamic Process
Protein turnover is the continuous process of protein synthesis and degradation that occurs within the body. This dynamic process allows cells to adapt to changing conditions, repair damaged proteins, and regulate enzyme activity. The rate of protein turnover varies depending on the tissue and the physiological state.
Highly active tissues, such as the liver and intestines, have high rates of protein turnover, while more stable tissues, such as muscle, have slower rates. Protein degradation primarily occurs via the ubiquitin-proteasome pathway and lysosomal autophagy.
The ubiquitin-proteasome pathway involves tagging proteins with ubiquitin, which marks them for degradation by the proteasome. Lysosomal autophagy involves the engulfment of cellular components, including proteins, by lysosomes, which then degrade them.
Deamination and Transamination: Key Metabolic Steps
Deamination is the removal of an amino group (-NH2) from an amino acid. This process is crucial because the amino group can be toxic if it accumulates in the body. The amino group is converted into ammonia, which is then converted to urea in the liver via the urea cycle and excreted in the urine.
Transamination involves the transfer of an amino group from one amino acid to a keto acid. This process is catalyzed by aminotransferases (also called transaminases), which require pyridoxal phosphate (vitamin B6) as a cofactor. Transamination allows the body to synthesize non-essential amino acids from essential amino acids, as well as interconvert amino acids.
Nitrogen Balance: Intake vs. Excretion
Nitrogen balance is a measure of the balance between nitrogen intake and nitrogen excretion. Nitrogen is a key component of amino acids and proteins, so nitrogen balance reflects protein metabolism. Positive nitrogen balance occurs when nitrogen intake exceeds nitrogen excretion, indicating net protein synthesis. This is typical during growth, pregnancy, and recovery from illness.
Negative nitrogen balance occurs when nitrogen excretion exceeds nitrogen intake, indicating net protein breakdown. This can occur during starvation, severe illness, or trauma. Nitrogen balance is a crucial indicator of nutritional status and can be used to assess the adequacy of protein intake.
In summary, the journey of dietary protein through digestion, absorption, and metabolism is a tightly regulated process. It provides the building blocks and energy needed for countless biological functions. Understanding these processes is crucial for nutrition and overall health.
Fueling the Body: Dietary Sources and Protein Requirements
After we consume protein-rich foods, a complex and carefully orchestrated series of events unfolds within the body to break down these large molecules into usable components. This process, involving digestion, absorption, and subsequent metabolic transformations, is critical for providing the body with the essential amino acids it needs for a myriad of functions. Understanding dietary sources of protein, therefore, becomes paramount.
Complete vs. Incomplete Proteins: The Amino Acid Profile
Proteins are not created equal. They differ significantly in their amino acid composition. This difference is what classifies them as either complete or incomplete proteins.
Complete proteins contain adequate amounts of all nine essential amino acids. These are histidine, isoleucine, leucine, lysine, methionine, phenylalanine, threonine, tryptophan, and valine. Animal products like meat, poultry, fish, eggs, and dairy are excellent sources of complete proteins.
Incomplete proteins, on the other hand, are deficient in one or more essential amino acids. Most plant-based protein sources fall into this category. Examples include legumes, grains, nuts, seeds, and vegetables. While individually these sources may lack certain amino acids, a well-planned diet can easily address this.
Protein Combining: The Art of Complementary Proteins
The concept of protein combining, also known as complementary proteins, is vital, especially for individuals following vegetarian or vegan diets. It involves consuming different incomplete protein sources throughout the day to ensure that all essential amino acids are adequately supplied.
For example, grains are often low in lysine but contain adequate amounts of methionine. Legumes, conversely, are typically rich in lysine but deficient in methionine. Combining grains and legumes, such as rice and beans, provides a complete amino acid profile.
This approach ensures the body receives all the building blocks it needs for protein synthesis, even without consuming animal products. Strategic planning is key to achieving this balance.
Navigating Vegetarian and Vegan Protein Intake
Vegetarian and vegan diets require careful planning to meet protein needs. Paying close attention to food combinations and diversifying protein sources is essential.
Beyond combining complementary proteins, vegetarians can also rely on eggs and dairy to fulfill their amino acid requirements. Vegans, however, must exclusively focus on plant-based sources and combinations.
Fortified foods, such as plant-based milks and cereals, can also contribute to meeting protein goals. Awareness and mindful meal planning are crucial for success.
Protein Supplements: A Double-Edged Sword
Protein supplements, including powders, bars, and drinks, are widely available and marketed for various purposes, from muscle building to weight management.
While supplements can be convenient for increasing protein intake, they are not always necessary or superior to whole food sources. Whole foods provide a broader range of nutrients, including vitamins, minerals, and fiber, which are absent in many supplements.
Furthermore, excessive protein intake from supplements can place unnecessary strain on the kidneys. It is crucial to consult with a healthcare professional or registered dietitian before incorporating protein supplements into your diet.
Decoding Food Labels: Understanding Protein Content
Food labels provide valuable information about the protein content of packaged foods. The Nutrition Facts panel lists the amount of protein in grams per serving.
Pay attention to the serving size and adjust your calculations accordingly. The Percent Daily Value (%DV) for protein is based on a 50-gram reference intake. Use this as a guide to understand how much a particular food contributes to your daily protein needs. Remember that individual protein requirements may vary.
Dietary Reference Intakes (DRIs): Establishing Protein Requirements
Dietary Reference Intakes (DRIs) are nutrient reference values developed by the Food and Nutrition Board of the Institute of Medicine. The Recommended Dietary Allowance (RDA) for protein for adults is 0.8 grams per kilogram of body weight per day.
This recommendation is intended to meet the needs of most healthy individuals. However, protein requirements can vary depending on factors such as age, activity level, and health status. Athletes, pregnant women, and individuals recovering from illness may require higher protein intakes.
Sports Nutrition: Optimizing Protein for Athletic Performance
Protein plays a crucial role in sports nutrition, supporting muscle repair, growth, and recovery. Athletes often require higher protein intakes than the general population to meet the demands of intense training.
The optimal protein intake for athletes varies depending on the type and intensity of exercise. Endurance athletes may require 1.2 to 1.4 grams of protein per kilogram of body weight per day, while strength athletes may benefit from 1.6 to 1.7 grams per kilogram of body weight per day.
Distributing protein intake evenly throughout the day, particularly after workouts, can optimize muscle protein synthesis and promote recovery. Careful attention to protein timing and source is essential for maximizing athletic performance.
The Science of Proteins: Techniques in Protein Analysis
Fueling the Body: Dietary Sources and Protein Requirements After we consume protein-rich foods, a complex and carefully orchestrated series of events unfolds within the body to break down these large molecules into usable components. This process, involving digestion, absorption, and subsequent metabolic transformations, is critical for providing the building blocks necessary for growth, repair, and myriad other physiological functions. But how do scientists delve into the intricate world of proteins to understand their structure, function, and behavior? The answer lies in a diverse array of sophisticated analytical techniques.
Unraveling Protein Mysteries: A Toolkit for Discovery
Scientists employ a range of tools to dissect the complex world of proteins, each providing unique insights into their structure and function. Mass spectrometry, chromatography, and gene sequencing are essential for identifying, quantifying, and characterizing these essential biomolecules. These techniques, refined over decades, empower researchers to explore the intricacies of protein biology and unlock new avenues for understanding health and disease.
Mass Spectrometry: Identifying and Quantifying Proteins
Mass spectrometry (MS) has revolutionized protein analysis, allowing scientists to identify and quantify proteins with remarkable precision and sensitivity. The basic principle involves ionizing molecules and then separating the ions based on their mass-to-charge ratio.
This creates a unique "fingerprint" for each protein, enabling its identification. Modern MS techniques, like tandem mass spectrometry (MS/MS), can even determine the amino acid sequence of a protein by fragmenting it into smaller pieces and analyzing the masses of the fragments.
Quantitative proteomics, a powerful application of MS, allows researchers to measure changes in protein expression levels in response to various stimuli or disease states. This information is invaluable for identifying potential drug targets and biomarkers.
Chromatography: Separating and Analyzing Amino Acids
Chromatography encompasses a range of techniques used to separate mixtures of molecules, including amino acids and proteins, based on their physical and chemical properties. Different chromatographic methods exist, each tailored for specific applications.
Gas chromatography (GC) is commonly used to separate volatile compounds, while high-performance liquid chromatography (HPLC) is ideal for separating a broader range of molecules, including proteins and peptides.
Ion-exchange chromatography separates molecules based on their charge, while size-exclusion chromatography separates them based on their size. Affinity chromatography uses specific interactions between a protein and a ligand to isolate the protein from a complex mixture.
These separation techniques are crucial for preparing protein samples for further analysis by mass spectrometry or other methods.
Gene Sequencing: Deciphering the Blueprint for Protein Synthesis
While not directly analyzing the protein itself, gene sequencing provides the blueprint for protein synthesis. By determining the nucleotide sequence of a gene, scientists can predict the corresponding amino acid sequence of the protein it encodes.
This information is essential for understanding protein function, as the amino acid sequence dictates the protein's three-dimensional structure and its interactions with other molecules.
Next-generation sequencing (NGS) technologies have dramatically increased the speed and throughput of gene sequencing, enabling researchers to analyze entire genomes and transcriptomes rapidly and cost-effectively. This has led to a surge in our understanding of the genetic basis of protein function and its role in disease.
The Synergy of Techniques: A Holistic Approach
It’s crucial to understand that these techniques are often used in combination to provide a complete picture of protein structure, function, and dynamics. For instance, a researcher might use chromatography to purify a protein sample, followed by mass spectrometry to identify and quantify the proteins present, and finally, use gene sequencing to understand the genetic basis of any observed differences.
By integrating these diverse techniques, scientists are continually pushing the boundaries of protein research and gaining deeper insights into the molecular mechanisms underlying life. This holistic approach is key to unlocking new diagnostics, therapies, and interventions for a wide range of diseases.
When Proteins Go Wrong: Deficiency Diseases and Metabolic Disorders
Fueling the Body: Dietary Sources and Protein Requirements The Science of Proteins: Techniques in Protein Analysis After we consume protein-rich foods, a complex and carefully orchestrated series of events unfolds within the body to break down these large molecules into usable components. This process, involving digestion, absorption, and subsequent metabolism, is normally seamless. However, when these processes are disrupted due to deficiency or genetic abnormalities, significant health consequences can arise. These disruptions manifest as deficiency diseases and metabolic disorders, underscoring the crucial importance of balanced protein intake and functional metabolic pathways.
Kwashiorkor: The Devastating Effects of Protein Deficiency
Kwashiorkor is a severe form of malnutrition most often seen in regions experiencing famine or limited food availability. It primarily affects children, typically after weaning, when their diets shift from protein-rich breast milk to carbohydrate-heavy, protein-poor foods.
The hallmark of Kwashiorkor is edema, particularly in the abdomen, ankles, and feet, caused by a lack of adequate protein for maintaining fluid balance. This fluid imbalance is linked to low levels of albumin, a protein synthesized in the liver and critical for maintaining osmotic pressure in the blood.
Other key features include:
- Growth retardation: Children with Kwashiorkor often fail to grow or develop at the expected rate.
- Muscle wasting: While edema may mask the loss of muscle mass, it is a significant feature of the disease.
- Skin lesions: Dermatitis, characterized by flaky skin and areas of discoloration, is common.
- Hair changes: Hair may become thin, brittle, and lose its natural color, often exhibiting a characteristic "flag sign" with alternating bands of light and dark.
- Irritability: Affected children are often irritable, apathetic, and prone to infections due to weakened immune systems.
Phenylketonuria (PKU): A Genetic Challenge in Phenylalanine Metabolism
Phenylketonuria (PKU) is an inherited metabolic disorder resulting from a deficiency in the enzyme phenylalanine hydroxylase (PAH). This enzyme is crucial for converting phenylalanine, an essential amino acid, into tyrosine.
Without functional PAH, phenylalanine accumulates in the blood and tissues, leading to toxic effects, particularly on the developing brain. If left untreated, PKU can cause intellectual disability, seizures, behavioral problems, and other neurological complications.
Newborn screening programs are essential for early detection of PKU, allowing for timely intervention through dietary management. The cornerstone of PKU treatment is a lifelong phenylalanine-restricted diet. This typically involves specialized formulas and careful monitoring of phenylalanine intake from food sources.
Management of PKU
Managing PKU requires a collaborative approach involving physicians, dietitians, and the patient (or their caregivers). The diet should provide sufficient protein for growth and development while limiting phenylalanine intake.
Foods high in protein, such as meat, fish, eggs, and dairy products, must be carefully restricted. Modified low-protein foods and phenylalanine-free amino acid mixtures are used to meet protein requirements.
Maple Syrup Urine Disease (MSUD): Disruptions in Branched-Chain Amino Acid Metabolism
Maple Syrup Urine Disease (MSUD) is another inherited metabolic disorder characterized by a deficiency in the branched-chain alpha-keto acid dehydrogenase (BCKAD) complex. This complex is responsible for the breakdown of branched-chain amino acids (BCAAs): leucine, isoleucine, and valine.
The buildup of BCAAs and their byproducts in the blood and urine leads to a characteristic sweet, maple syrup-like odor in the affected individual's urine, hence the name of the disease.
MSUD can manifest in various forms, ranging from classic (severe) to intermediate and intermittent. The classic form of MSUD, if untreated, can lead to severe neurological damage, including seizures, coma, and death.
Treatment Strategies for MSUD
As with PKU, early diagnosis and dietary management are critical for MSUD. Treatment focuses on:
- Limiting BCAA intake: A special diet restricted in leucine, isoleucine, and valine is necessary.
- Specialized formulas: BCAA-free amino acid mixtures are used to ensure adequate protein intake without causing BCAA accumulation.
- Monitoring: Regular monitoring of BCAA levels is essential to adjust dietary interventions and prevent metabolic crises.
Protein-Energy Malnutrition (PEM): A Broader Perspective
Protein-Energy Malnutrition (PEM) is a broad term encompassing a range of conditions resulting from deficiencies in both protein and energy. It represents a spectrum of malnutrition and is a significant public health problem worldwide, particularly in developing countries.
PEM can manifest in various forms, including Kwashiorkor (primarily protein deficiency) and Marasmus (severe energy and protein deficiency, leading to extreme wasting).
PEM can have devastating consequences, including:
- Impaired growth and development
- Weakened immune function, increasing susceptibility to infections
- Increased morbidity and mortality
Addressing PEM requires a multifaceted approach, including improving access to nutritious foods, promoting breastfeeding, and providing nutritional support and education.
The interplay between genetics, environment, and nutrition underscores the complexity of these conditions. Understanding the biochemical pathways and the impact of deficiencies and genetic abnormalities is critical for developing effective strategies for prevention, diagnosis, and treatment. Ongoing research continues to shed light on these complex disorders, paving the way for improved outcomes and quality of life for affected individuals.
[When Proteins Go Wrong: Deficiency Diseases and Metabolic Disorders Fueling the Body: Dietary Sources and Protein Requirements The Science of Proteins: Techniques in Protein Analysis After we consume protein-rich foods, a complex and carefully orchestrated series of events unfolds within the body to break down these large molecules into usable components. However, the scientific understanding that underpins this knowledge spans across numerous disciplines, each offering a unique perspective.
Behind the Scenes: The Interdisciplinary World of Protein Science
The study of proteins and amino acids is far from a solitary endeavor. It requires the combined expertise of various scientific disciplines, each contributing a critical piece to the overall understanding. Biochemistry, nutrition, molecular biology, and medicine stand out as particularly relevant, providing complementary insights into the intricate world of these essential biomolecules.
Biochemistry: Unraveling the Molecular Mechanisms
Biochemistry lies at the heart of protein science, providing the fundamental understanding of the chemical processes that govern protein structure, function, and metabolism.
It explores how proteins are synthesized, folded, modified, and degraded within living organisms.
Enzymes, which are predominantly proteins, catalyze virtually every biochemical reaction.
Thus, biochemistry elucidates the mechanisms of enzyme action and regulation. This includes detailed analyses of metabolic pathways and how they are affected by various factors.
Nutrition: The Dietary Perspective on Protein
Nutrition focuses on the impact of food on the body. It places specific emphasis on the dietary requirements for proteins and amino acids to maintain optimal health.
Nutritional science investigates the sources of dietary protein, assessing the quality and bioavailability of amino acids from different food sources.
This field also examines the consequences of protein deficiencies or excesses. These consequences range from impaired growth and development to increased risk of chronic diseases.
Moreover, nutrition explores how dietary protein interacts with other nutrients to support overall metabolic function.
Molecular Biology: Decoding the Genetic Blueprint
Molecular biology provides the framework for understanding protein synthesis and function at the molecular level.
This discipline explores the central dogma of molecular biology: DNA → RNA → Protein.
This dogma details how genetic information is transcribed into RNA and subsequently translated into protein sequences.
Molecular biology techniques are used to study gene expression, protein structure, and protein-protein interactions. They offer insights into the cellular mechanisms that regulate protein production and activity.
Medicine: Translating Science into Clinical Practice
Medicine plays a crucial role in translating the knowledge gained from biochemistry, nutrition, and molecular biology into clinical practice.
This involves understanding and treating deficiency diseases and metabolic disorders that are related to proteins and amino acids.
Medical professionals diagnose and manage conditions such as Phenylketonuria (PKU) and Maple Syrup Urine Disease (MSUD). Both of these are inborn errors of metabolism.
They also address acquired conditions like Kwashiorkor, a severe form of protein-energy malnutrition.
Furthermore, medicine utilizes protein-based therapies, such as enzyme replacement therapy and therapeutic antibodies, to treat a wide range of diseases.
By integrating insights from these diverse disciplines, we gain a comprehensive understanding of the critical roles that proteins and amino acids play in maintaining health and combating disease. The synergy between these fields continues to drive advancements in both basic and applied protein science.
Pioneers of Protein Science: Unveiling the Building Blocks of Life
Following an examination of what happens when protein and amino acid metabolism falters, it’s crucial to recognize the towering figures who laid the groundwork for our current understanding. Their dedication and groundbreaking discoveries have transformed the fields of biochemistry, nutrition, and medicine.
The Architects of Our Knowledge
These pioneers, through rigorous experimentation and insightful analysis, deciphered the intricate roles of proteins and amino acids in biological systems. Their work revealed not only the identity of these essential molecules but also their profound impact on human health and disease.
William Cumming Rose: Charting the Essential Amino Acids
William Cumming Rose stands as a monumental figure in the history of nutritional science. His meticulous research during the mid-20th century revolutionized our understanding of dietary protein requirements.
Rose dedicated years to identifying the essential amino acids—those that the human body cannot synthesize and must obtain from the diet. Through painstaking experiments, he systematically eliminated individual amino acids from the diets of human subjects.
He then observed the resulting health consequences.
The Discovery of Threonine
Perhaps Rose's most celebrated achievement was the identification of threonine in 1935 as an essential amino acid. This discovery, along with his identification of other essential amino acids, fundamentally changed the way we think about protein nutrition.
It provided a scientific basis for understanding the importance of a balanced diet.
Rose's work had immediate and far-reaching implications. It informed dietary guidelines, improved the treatment of malnutrition, and laid the foundation for the development of specialized medical foods. His legacy continues to shape nutritional recommendations worldwide.
Frederick Sanger: Sequencing the Secrets of Insulin
Frederick Sanger, a British biochemist, revolutionized the field of protein chemistry with his groundbreaking work on protein sequencing. Sanger's development of the first method for determining the amino acid sequence of a protein earned him the Nobel Prize in Chemistry in 1958.
Deciphering Insulin's Structure
Sanger chose insulin, a hormone crucial for regulating blood sugar, as his target molecule. Insulin was complex enough to present a significant challenge, yet small enough to be manageable with the available technology.
Over a decade, Sanger and his team meticulously broke down insulin into smaller fragments. They then identified and ordered the amino acids within each fragment. Through careful reconstruction, they pieced together the complete amino acid sequence of insulin.
A Paradigm Shift in Biochemistry
Sanger's achievement was more than just the sequencing of a single protein. It provided a proof of concept that proteins had a defined and ordered structure. This was a paradigm shift in biochemistry. It opened up new avenues of research into protein function and the relationship between amino acid sequence and biological activity.
His techniques paved the way for the development of automated sequencing technologies. This has fueled the explosion of knowledge in genomics and proteomics. Sanger's contributions continue to resonate across the life sciences.
Legacies of Innovation
Rose and Sanger, though working in different areas of protein science, shared a common thread: a relentless pursuit of knowledge and a commitment to rigorous experimentation. Their discoveries have had a lasting impact on our understanding of the molecular basis of life. Their legacies continue to inspire scientists to push the boundaries of knowledge and to develop new tools and techniques for exploring the complexities of the biological world.
Video: Essential Amino Acids: Building Blocks of Proteins
Essential Amino Acids: Building Blocks of Proteins FAQs
What makes an amino acid "essential"?
Essential amino acids are those your body can't produce on its own, or can't produce enough of. You must obtain them through your diet to ensure your body has all the necessary building blocks of proteins.
Why are essential amino acids important?
They are vital for many bodily functions, including muscle building, hormone production, and enzyme creation. They’re the fundamental building blocks of proteins, which are essential for growth, repair, and overall health.
What happens if I don't get enough essential amino acids?
Deficiency can lead to various health problems, including muscle loss, weakened immunity, digestive issues, and impaired growth, especially in children. It hinders your body's ability to create the proteins it needs to function properly. These proteins are the building blocks of proteins used for everything from tissue repair to enzyme function.
Where can I find essential amino acids in my diet?
Good sources include meat, poultry, fish, eggs, dairy products, and certain plant-based foods like quinoa and soy. A varied diet generally provides all the necessary building blocks of proteins your body needs.
So, there you have it! Essential amino acids really are the building blocks of proteins, and understanding them is key to fueling your body right. Whether you're a seasoned athlete or just trying to live a healthier life, making sure you're getting enough of these little guys is a great step in the right direction. Now go forth and nourish!