Equivalence Point Explained: Master Titration Now!
Titration, a fundamental laboratory technique, finds its most crucial moment at the equivalence point. This pivotal state, often determined using indicators like phenolphthalein or via sophisticated pH meters, signifies the complete neutralization of an analyte. The concept of equivalence point is intimately linked to stoichiometry; accurate calculations, often performed by analytical chemists, ensure that the reaction has proceeded to completion. Understanding the equivalence point is essential for applications ranging from pharmaceutical analysis to environmental monitoring.
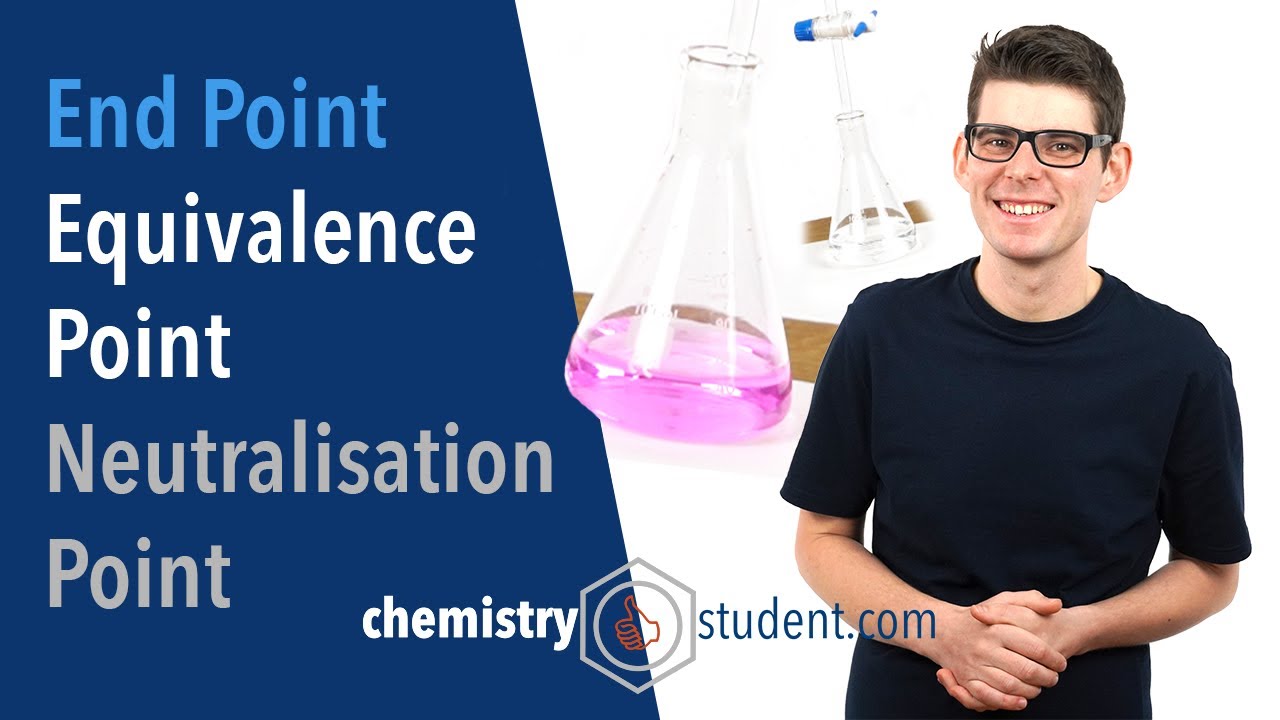
Image taken from the YouTube channel Chemistry Student , from the video titled Titrations - Equivalence Point, End Point and Neutralisation Point (A-level Chemistry) .
Titration stands as a cornerstone technique in quantitative chemical analysis, offering a precise method for determining the concentration of an unknown solution. It's a process where a solution of known concentration reacts with a solution of unknown concentration to determine the unknown's concentration. The accuracy of any titration hinges on a single, critical point: the equivalence point.
What is Titration?
At its core, titration is a laboratory technique used to determine the concentration of an analyte (the substance being analyzed). This is achieved by gradually adding a titrant, a solution of known concentration, to the analyte until the reaction between them is complete.
Titration plays a crucial role in various fields, including:
- Environmental monitoring: Determining pollutant levels in water or soil.
- Pharmaceutical analysis: Ensuring the correct concentration of active ingredients in medications.
- Food science: Measuring the acidity or alkalinity of food products.
- Industrial chemistry: Monitoring the quality of raw materials and finished products.
The Equivalence Point: The Heart of Titration
The equivalence point represents the theoretical point in a titration where the amount of titrant added is stoichiometrically equivalent to the amount of analyte present. In simpler terms, it's the point where the titrant has completely reacted with the analyte, based on the balanced chemical equation for the reaction.
Achieving and accurately identifying the equivalence point is paramount. It is the key to calculating the unknown concentration. A miscalculated or poorly determined equivalence point directly translates to inaccurate titration results, rendering the analysis unreliable.
Mastering the Equivalence Point: Our Goal
This article aims to provide a comprehensive understanding of the equivalence point, its significance, and how to accurately determine it in various titration scenarios. We will delve into the theoretical underpinnings of the equivalence point. We will explore practical methods for its identification, ensuring that you gain the knowledge and skills necessary to perform accurate and reliable titrations. Ultimately, you'll have mastered the concept.
Titration stands as a cornerstone technique in quantitative chemical analysis, offering a precise method for determining the concentration of an unknown solution. It's a process where a solution of known concentration reacts with a solution of unknown concentration to determine the unknown's concentration. The accuracy of any titration hinges on a single, critical point: the equivalence point.
Mastering the art of titration, however, requires more than just understanding the equivalence point. It demands a solid grasp of the fundamental terminology that underpins the entire process. Before diving deeper into the intricacies of the equivalence point, let's establish a strong foundation by defining the key players and concepts involved in titration.
Titration Terminology: A Foundation for Understanding
To navigate the world of titrations effectively, a firm understanding of its core vocabulary is essential. Let's break down the key terms that form the bedrock of this analytical technique.
The Titrant: The Known Quantity
The titrant, also often referred to as the standard solution, is the reagent of precisely known concentration that is added to the analyte.
Its role is to react with the analyte in a controlled and quantifiable manner.
The titrant is typically added gradually, often using a burette, to ensure accuracy and control over the reaction.
The Analyte: The Unknown Quantity
Conversely, the analyte is the solution being analyzed. Its concentration is what we ultimately seek to determine through the titration process.
The analyte reacts with the titrant, and by carefully monitoring this reaction, we can deduce the original concentration of the analyte.
Standard Solutions: Preparing the Titrant
A standard solution is a crucial component of any accurate titration. It is a solution with a precisely known concentration of a specific substance.
The accuracy of the entire titration hinges on the accuracy of the standard solution.
These solutions are typically prepared in two ways:
- Direct Preparation: Dissolving a precisely weighed amount of a highly pure solid in a known volume of solvent. This method requires a primary standard, a reagent that is exceptionally pure, stable, and has a high molecular weight to minimize weighing errors.
- Standardization: Determining the concentration of a solution by titrating it against a primary standard. This is necessary when the titrant is not available in sufficiently pure form or is prone to changes in concentration over time.
Indicators: Signaling the Endpoint
Indicators are substances that undergo a visible change, usually a color change, near the equivalence point of the titration.
This color change signals the endpoint of the titration, which is an approximation of the equivalence point.
The ideal indicator should change color as close as possible to the true equivalence point to minimize titration error.
Types of Indicators and pH Ranges
Many different indicators exist, each exhibiting a color change within a specific pH range.
The choice of indicator depends on the type of titration being performed and the pH at the equivalence point.
For example:
- Phenolphthalein: A common indicator that is colorless in acidic solutions and pink in basic solutions (pH range 8.3-10.0). It's often used in titrations of strong acids with strong bases.
- Methyl Orange: Red in acidic solutions and yellow in basic solutions (pH range 3.1-4.4). Useful for titrations involving stronger acids.
- Bromothymol Blue: Yellow in acidic solutions and blue in basic solutions (pH range 6.0-7.6). Suitable for titrations where the equivalence point is near neutral pH.
Understanding the properties and pH ranges of different indicators is critical for selecting the appropriate indicator for a given titration, ensuring accurate results.
The Equivalence Point: Definition and Significance
With the key terminology of titration now defined, the next logical step is to delve into the heart of the process: the equivalence point. It’s the theoretical bullseye we're aiming for in every titration, the point where our reaction reaches completion.
Defining the Equivalence Point
The equivalence point in a titration is the point at which the amount of titrant added is stoichiometrically equivalent to the amount of analyte present in the sample. Put simply, it's where the titrant has completely reacted with the analyte.
At the equivalence point, the moles of titrant added relate to the moles of analyte present according to the balanced chemical equation for the reaction. This is a theoretical concept, representing the ideal completion of the reaction.
Stoichiometry at the Equivalence Point
Understanding the stoichiometry of the reaction is paramount when determining the equivalence point. Stoichiometry refers to the quantitative relationship between reactants and products in a chemical reaction.
For example, in the reaction between hydrochloric acid (HCl) and sodium hydroxide (NaOH), the reaction is 1:1:
HCl(aq) + NaOH(aq) → NaCl(aq) + H2O(l)
This means that one mole of HCl will react completely with one mole of NaOH. At the equivalence point, the number of moles of HCl added as the titrant will equal the number of moles of NaOH originally present as the analyte.
However, not all reactions have a 1:1 stoichiometry. Some reactions may require a different molar ratio.
For instance, the reaction between sulfuric acid (H2SO4) and sodium hydroxide (NaOH) has a 1:2 stoichiometry:
H2SO4(aq) + 2NaOH(aq) → Na2SO4(aq) + 2H2O(l)
In this case, one mole of sulfuric acid reacts with two moles of sodium hydroxide. Therefore, at the equivalence point, the number of moles of NaOH added as the titrant will be twice the number of moles of H2SO4 originally present as the analyte.
Calculating Analyte Concentration Using the Equivalence Point
The equivalence point is crucial because it allows us to calculate the concentration of the unknown analyte. By knowing the volume and concentration of the titrant added to reach the equivalence point, and by understanding the stoichiometry of the reaction, we can determine the moles of analyte present in the original sample.
Here's the general formula:
Moles of Analyte = Moles of Titrant × (Stoichiometric Ratio)
Once the moles of analyte are known, the concentration can be calculated using the following formula:
Concentration of Analyte = Moles of Analyte / Volume of Analyte Solution
For example, consider a titration where 20.0 mL of 0.1 M HCl is required to reach the equivalence point with 25.0 mL of an unknown NaOH solution. The reaction is 1:1.
- Moles of HCl = (0.020 L) * (0.1 mol/L) = 0.002 moles
- Moles of NaOH = 0.002 moles (since the ratio is 1:1)
- Concentration of NaOH = (0.002 moles) / (0.025 L) = 0.08 M
Therefore, the concentration of the unknown NaOH solution is 0.08 M.
Understanding the equivalence point, and how to calculate analyte concentration from it, is essential for performing accurate titrations and obtaining reliable quantitative results.
Endpoint vs. Equivalence Point: Understanding the Nuances of Titration
We've established the equivalence point as the theoretical ideal in a titration, that moment of perfect stoichiometric balance between titrant and analyte. However, in practice, we rely on visual cues, such as indicator color changes, to signal the reaction's completion. This brings us to a critical distinction: the difference between the endpoint and the equivalence point.
Defining the Endpoint
The endpoint of a titration is the point at which a noticeable change occurs that signals the end of the titration.
Most commonly, this involves a color change in a chemical indicator.
The indicator is selected so that this change occurs as close as possible to the equivalence point.
The key difference here is that the equivalence point is a calculated, theoretical value, whereas the endpoint is an observed, experimental value.
Endpoint as an Approximation
The endpoint serves as an approximation of the equivalence point.
Ideally, these two points would coincide perfectly.
However, the indicator changes color over a small range of titrant volumes, not at a single, defined point.
This is largely due to the indicator itself undergoing a chemical change that's dependent on concentration and pH.
Consequently, the endpoint inherently carries a degree of error.
Factors Causing Discrepancies
Several factors contribute to the discrepancy between the endpoint and the equivalence point.
Indicator selection is paramount. The pH range in which the indicator changes color must overlap with the pH at the equivalence point.
If the indicator changes color too early or too late, a significant error will be introduced.
The concentration of the indicator can also play a role. Too much indicator can skew the results by contributing to the solution's color even before the equivalence point is reached.
Additionally, subjective interpretation of the color change can vary between individuals, leading to inconsistencies.
Finally, in certain titrations, the reaction may proceed slowly near the equivalence point. This can lead to over-titration, pushing the endpoint beyond the true equivalence point.
Strategies for Minimization
While it's impossible to eliminate the difference between the endpoint and equivalence point entirely, several strategies can minimize the error:
Careful Indicator Selection
Choose an indicator whose transition range closely matches the pH at the equivalence point. This requires some knowledge of the reaction being studied. Reference materials and titration curves can assist in indicator selection.
Minimizing Indicator Concentration
Use the smallest amount of indicator necessary to observe a clear color change. This reduces the indicator's influence on the solution's pH and color.
Precise Titration Technique
Add the titrant slowly, especially near the expected endpoint. Dropwise addition allows for more precise control and prevents over-titration.
Employing a Titration Blank
Perform a titration blank to account for any interference from the indicator itself or other components in the solution.
This involves performing the titration without the analyte present. The volume of titrant required to reach the endpoint in the blank titration can then be subtracted from the volume required in the actual titration.
Instrumental Methods
Consider using instrumental methods such as potentiometry or spectrophotometry to determine the equivalence point.
These methods rely on direct measurement of pH or absorbance, rather than subjective visual assessment of a color change.
They can provide more accurate and precise results, particularly for complex titrations or when dealing with colored solutions.
By understanding the nuances between the endpoint and equivalence point, and by implementing appropriate strategies, we can significantly improve the accuracy and reliability of titration results.
Tools and Equipment: Essential for Accurate Titration
Having explored the subtle yet critical differences between the endpoint and the equivalence point, it becomes clear that achieving accurate titration results hinges on more than just theoretical understanding. The quality of the tools we use and the precision with which we employ them are equally paramount. Let's delve into the essential equipment for titration, emphasizing the role of each component in securing reliable and reproducible data.
The Burette: A Controlled Release System
The burette stands as the cornerstone of any titration setup. It is a graduated glass tube with a stopcock at its lower end, designed for the precise dispensing of liquid titrant.
Its narrow bore and fine graduations allow for accurate volume measurements, often to the nearest 0.01 mL.
Proper technique is critical when using a burette. It involves careful reading of the meniscus at eye level to avoid parallax errors.
Equally important is the slow, controlled addition of titrant, especially as you approach the endpoint, to ensure you don't overshoot the mark.
The Pipette: Precision Volume Delivery
While the burette delivers the titrant, the pipette is primarily responsible for accurately measuring the analyte volume.
Pipettes come in various forms, including volumetric pipettes, which deliver a single, precise volume, and graduated pipettes, which allow for variable volume delivery.
For titrations, volumetric pipettes are generally preferred when dispensing a known volume of the analyte, as they offer the highest level of accuracy.
Like the burette, proper usage is key. This involves drawing the liquid up to the calibration mark, releasing it while ensuring the tip remains in contact with the receiving vessel, and allowing the liquid to drain under gravity.
The Erlenmeyer Flask: A Reaction Vessel
The Erlenmeyer flask serves as the reaction vessel where the titrant and analyte meet and react. Its conical shape and wide base make it ideal for swirling the reaction mixture to ensure thorough mixing.
Unlike beakers, the conical shape of Erlenmeyer flasks minimizes the risk of spillage during titration, especially when swirling.
While not typically used for precise volume measurements, the Erlenmeyer flask provides a stable and convenient environment for the titration reaction to occur.
The Importance of Calibrated Glassware
All glassware used in titration, including burettes, pipettes, and volumetric flasks, must be properly calibrated to ensure the accuracy of the results.
Calibration involves determining the exact volume of liquid delivered or contained by the glassware at a specific temperature.
Calibration is crucial because glassware manufacturing tolerances can introduce slight variations in volume, which can significantly impact the accuracy of the titration.
Calibration should be performed regularly, especially for glassware that is frequently used or exposed to harsh chemicals.
By meticulously employing calibrated equipment and mastering proper techniques, the accuracy of titration experiments can be significantly improved.
Having established the crucial role of equipment in achieving titration accuracy, let's now shift our focus from the how to the what of titrations.
While the principles of endpoint determination and meticulous technique remain constant, the chemical reactions that drive a titration can vary significantly. This variation gives rise to different types of titrations, each tailored to analyze specific types of substances.
Types of Titrations: Acid-Base and Beyond
Titration, at its core, is a method of quantitative chemical analysis that relies on the complete reaction between the analyte (the substance being analyzed) and the titrant (a solution of known concentration).
However, the nature of this reaction dictates the type of titration performed. While acid-base titrations are perhaps the most commonly encountered, the world of titrimetry extends far beyond, encompassing redox, complexometric, and precipitation titrations, each with its unique applications.
Acid-Base Titrations: The Foundation
Acid-base titrations are predicated on the neutralization reaction between an acid and a base. This type of titration is used extensively to determine the concentration of acidic or basic solutions. The key principle is the reaction between hydrogen ions (H+) from the acid and hydroxide ions (OH-) from the base to form water (H2O).
The progress of the reaction is typically monitored using an indicator, a substance that changes color depending on the pH of the solution, or a pH meter, which provides a direct reading of the pH.
Strong Acid-Strong Base Titrations
The titration of a strong acid with a strong base serves as an excellent illustration of acid-base titration principles.
In this scenario, the reaction proceeds essentially to completion, meaning the acid and base react quantitatively until one or the other is entirely consumed. The pH curve for this type of titration exhibits a sharp change in pH near the equivalence point, making endpoint detection relatively straightforward using a suitable indicator.
Weak Acid/Base Titrations and Buffer Regions
Titrating a weak acid with a strong base (or vice versa) introduces additional complexity. Unlike strong acids and bases, weak acids and bases do not fully dissociate in solution.
This incomplete dissociation results in a more gradual change in pH during the titration, and the pH at the equivalence point will not be exactly 7. Furthermore, a buffer region emerges during the titration.
The Role of Buffer Solutions
A buffer solution resists changes in pH upon the addition of small amounts of acid or base. In the context of weak acid/base titrations, the buffer region occurs because the weak acid (or base) and its conjugate base (or acid) coexist in solution.
This buffering action moderates the pH change, creating a flatter region on the titration curve before the sharp rise or fall near the equivalence point. Understanding the buffer region is crucial for selecting an appropriate indicator that changes color within the steepest part of the curve, ensuring accurate endpoint determination.
Beyond Acid-Base: A Glimpse at Other Titration Types
While acid-base titrations hold a prominent place, the versatility of titration extends to other reaction types:
-
Redox Titrations: These titrations involve oxidation-reduction reactions, where electrons are transferred between the analyte and titrant. They are used to determine the concentration of oxidizing or reducing agents.
-
Complexometric Titrations: These titrations rely on the formation of a complex between the analyte and the titrant. EDTA (ethylenediaminetetraacetic acid) is a common titrant in complexometric titrations, used to determine the concentration of metal ions.
Each type of titration requires a specific set of reagents, indicators, and techniques, tailored to the particular chemistry involved. However, the fundamental principle remains the same: the precise determination of the equivalence point to quantify the analyte of interest.
However, understanding the theoretical underpinnings is only half the battle. Successfully performing titrations hinges on your ability to translate these concepts into practice. Let's delve into practical examples that illustrate how to calculate the equivalence point, solidifying your grasp of these vital calculations.
Calculating the Equivalence Point: Worked Examples
The equivalence point, as we've established, is the cornerstone of accurate titrations. Calculating it precisely allows us to determine the unknown concentration of our analyte. Let's explore several worked examples, covering both strong acid-strong base and weak acid-strong base scenarios, while also incorporating varying stoichiometric ratios to enhance your problem-solving skills.
Strong Acid-Strong Base Titration: A 1:1 Stoichiometry Example
Consider the titration of 25.00 mL of 0.100 M hydrochloric acid (HCl) with 0.100 M sodium hydroxide (NaOH). HCl is a strong acid, and NaOH is a strong base, and they react in a 1:1 molar ratio.
The balanced chemical equation is:
HCl(aq) + NaOH(aq) → NaCl(aq) + H2O(l)
At the equivalence point, the moles of acid will equal the moles of base.
Moles of HCl = Molarity × Volume = 0.100 mol/L × 0.025 L = 0.0025 moles.
Since the reaction is 1:1, we need 0.0025 moles of NaOH to reach the equivalence point. Now, we calculate the volume of NaOH required:
Volume of NaOH = Moles / Molarity = 0.0025 moles / 0.100 mol/L = 0.025 L = 25.00 mL.
Therefore, the equivalence point is reached when 25.00 mL of 0.100 M NaOH has been added.
Weak Acid-Strong Base Titration: Acetic Acid and NaOH
Let's analyze the titration of 25.00 mL of 0.100 M acetic acid (CH3COOH) with 0.100 M NaOH. Acetic acid is a weak acid, requiring a slightly different approach due to its incomplete dissociation.
The balanced chemical equation is:
CH3COOH(aq) + NaOH(aq) → CH3COONa(aq) + H2O(l)
Similar to the previous example, the reaction follows a 1:1 stoichiometry. The moles of acetic acid initially present are:
Moles of CH3COOH = Molarity × Volume = 0.100 mol/L × 0.025 L = 0.0025 moles.
To reach the equivalence point, we need an equal number of moles of NaOH: 0.0025 moles. The volume of NaOH required can be calculated as follows:
Volume of NaOH = Moles / Molarity = 0.0025 moles / 0.100 mol/L = 0.025 L = 25.00 mL.
In this case, even though we are titrating a weak acid, the volume of NaOH required to reach the equivalence point is the same as in the strong acid case because the stoichiometry is 1:1. However, the pH at the equivalence point will be different due to the formation of the acetate ion (CH3COO-), which is a weak base.
Accounting for Different Stoichiometric Ratios
Titrations don't always occur with 1:1 stoichiometry. Let's consider an example with a different ratio.
Imagine titrating 20.00 mL of 0.050 M sulfuric acid (H2SO4) with 0.100 M KOH (potassium hydroxide). Sulfuric acid is a diprotic acid, meaning it has two acidic protons, and it reacts with KOH in a 1:2 ratio.
The balanced chemical equation is:
H2SO4(aq) + 2KOH(aq) → K2SO4(aq) + 2H2O(l)
First, calculate the moles of sulfuric acid:
Moles of H2SO4 = Molarity × Volume = 0.050 mol/L × 0.020 L = 0.001 moles.
Because of the 1:2 stoichiometry, we need twice as many moles of KOH to react completely with the sulfuric acid:
Moles of KOH = 2 × Moles of H2SO4 = 2 × 0.001 moles = 0.002 moles.
Now, calculate the volume of KOH required:
Volume of KOH = Moles / Molarity = 0.002 moles / 0.100 mol/L = 0.020 L = 20.00 mL.
Therefore, the equivalence point is reached when 20.00 mL of 0.100 M KOH has been added.
These examples showcase the fundamental principles behind calculating the equivalence point in different titration scenarios. By carefully considering the stoichiometry of the reaction and applying the molarity and volume relationships, you can accurately determine the equivalence point and, consequently, the concentration of your analyte. Remember to always double-check your calculations and units to ensure accuracy.
Of course, here's the standalone section of the article you requested, expanded from the original outline provided:
pH Curves and Equivalence Point Determination
Having explored the calculations that pinpoint the equivalence point, it's time to visualize this critical concept. pH curves, generated during titrations, offer a powerful graphical method for identifying the equivalence point. These curves plot pH values against the volume of titrant added, providing a visual representation of the titration process.
Understanding pH Curves
A pH curve is essentially a graph that tracks the change in pH of a solution as a titrant is added.
The shape of the curve provides valuable information about the strength of the acid and base involved, and most importantly, pinpoints the equivalence point.
The equivalence point on the pH curve is the point where the pH changes most rapidly with the addition of titrant.
Let's delve into the characteristic shapes of pH curves for different types of titrations.
pH Curve for Strong Acid-Strong Base Titration
The pH curve for a strong acid-strong base titration exhibits a distinctive S-shape. Initially, the pH changes gradually as the strong acid is neutralized.
As the equivalence point is approached, there is a rapid and dramatic change in pH.
This sharp vertical region makes it relatively easy to identify the equivalence point.
The equivalence point for a strong acid-strong base titration will always be at pH 7, because neither the conjugate base of the strong acid nor the conjugate acid of the strong base will undergo hydrolysis.
pH Curve for Weak Acid-Strong Base Titration
The pH curve for a weak acid-strong base titration differs significantly from that of a strong acid-strong base titration.
The initial pH is higher than that of a strong acid, and the pH change near the equivalence point is less abrupt.
A key feature of this curve is the presence of a buffer region before the equivalence point.
This region is where the weak acid and its conjugate base coexist in significant concentrations, resisting drastic pH changes upon the addition of small amounts of base.
The equivalence point for a weak acid-strong base titration will be at a pH greater than 7, due to the hydrolysis of the conjugate base of the weak acid.
Identifying the Equivalence Point from pH Curves
The equivalence point can be visually determined from the pH curve by identifying the inflection point of the steep vertical region.
This can be done manually by estimating the midpoint of the vertical section or by using software to calculate the first or second derivative of the curve.
Visual Determination
Locate the steepest part of the curve; the midpoint of this section represents the equivalence point.
Draw a vertical line from this midpoint to the x-axis (volume of titrant added).
The corresponding volume represents the volume of titrant needed to reach the equivalence point.
Using Example Graphs
Imagine a graph depicting the titration of acetic acid (a weak acid) with sodium hydroxide (a strong base).
The curve starts at a relatively higher pH, gradually increases, and then shows a less pronounced vertical jump near the equivalence point.
The equivalence point will be located slightly above pH 7.
Derivative Plots: A More Precise Method
Derivative plots provide an alternative and often more precise method for detecting the equivalence point.
By plotting the derivative of the pH curve (the rate of change of pH with respect to volume) against the volume of titrant, the equivalence point is identified as the peak of the derivative curve.
This method is particularly useful for titrations where the pH change near the equivalence point is less sharp, such as in weak acid-strong base titrations, making equivalence point detection much easier and more reliable.
Derivative plots provide a clearer, mathematical approach to pinpointing this critical point, and offer a valuable tool for accurate titration analysis.
Having examined pH curves and their use in pinpointing the equivalence point, it is crucial to acknowledge that achieving accurate titration results depends heavily on minimizing experimental errors. Let's delve into common error sources in titrations and how to address them.
Minimizing Errors: Achieving Accurate Titration Results
Titration, while a powerful analytical technique, is susceptible to various errors that can compromise the accuracy of the results. Diligence and attention to detail are paramount. Recognizing and addressing these potential pitfalls is essential for obtaining reliable and meaningful data.
Common Sources of Error in Titrations
Several factors can introduce errors into titration experiments. Addressing these factors directly leads to more precise outcomes.
-
Inaccurate Volume Measurements: One of the most significant sources of error stems from inaccurate volume measurements. This includes errors in measuring both the titrant and the analyte.
- Burette Reading Errors: Misreading the burette scale, parallax errors, and improper meniscus alignment can all contribute to volume inaccuracies.
- Pipette Errors: Using improperly calibrated pipettes or failing to properly control the drainage rate can lead to errors in the amount of analyte delivered.
- Volumetric Flask Errors: Erroneous use of the volumetric flask, like failing to ensure the solution is mixed well or reading the meniscus incorrectly, can cause inconsistencies.
-
Incorrect Indicator Choice: The choice of indicator is critical. The indicator's color change must occur as close as possible to the equivalence point to minimize the difference between the endpoint and the equivalence point.
- If the indicator changes color too early or too late, it leads to a significant error in determining the actual equivalence point.
- Understanding the pH range of the titration at the equivalence point is necessary for selecting an appropriate indicator.
-
Improper Technique: Errors in technique can significantly impact accuracy. This includes issues with mixing, dropwise addition, and handling the equipment.
-
Standard Solution Instability: The concentration of the standard solution, or titrant, is assumed to be known precisely. If the standard solution degrades or reacts with the atmosphere, its concentration changes, leading to systematic errors.
- Some standard solutions, like sodium hydroxide, readily absorb carbon dioxide from the air, altering their concentration.
- Regular standardization of the titrant against a primary standard is necessary to ensure accuracy.
-
Reaction Rate and Equilibrium: In some titrations, the chemical reaction between the titrant and analyte might not proceed rapidly or completely to equilibrium.
- Slow reactions or incomplete reactions can make it difficult to accurately determine the endpoint.
- Heating the solution or using a catalyst may be necessary in some cases to accelerate the reaction.
Tips for Improving Titration Technique
Adopting careful and refined techniques can significantly reduce errors in titration experiments. Let's examine some strategies for improving procedural accuracy.
-
Careful Dropwise Addition Near the Endpoint: As the titration approaches the endpoint, it is crucial to add the titrant dropwise. This ensures that the endpoint is not overshot, reducing the error between the endpoint and the equivalence point.
- Using a wash bottle to rinse the sides of the flask helps to ensure all titrant reaches the reaction mixture.
-
Proper Mixing: Continuous and thorough mixing of the solution during the titration is essential. This ensures that the titrant reacts evenly with the analyte, preventing localized over-titration.
- Magnetic stirrers are particularly useful for maintaining consistent mixing.
-
Accurate Burette Readings: Take burette readings at eye level to avoid parallax errors. Ensure the meniscus is properly aligned with the graduation mark.
- Use a burette with fine graduations for greater precision.
-
Appropriate Titration Speed: Maintain a consistent titration speed while ensuring thorough mixing and awareness of subtle color changes that signal the approach of the endpoint.
-
Replicate Titrations: Performing multiple titrations and averaging the results can help to identify and minimize random errors. Replicate trials also provide a measure of the precision of the analysis.
Equipment Calibration and Controls
Proper equipment calibration and the use of appropriate controls are vital for ensuring the accuracy and reliability of titration results.
-
Calibration of Volumetric Glassware: Burettes, pipettes, and volumetric flasks should be calibrated regularly to ensure accurate volume measurements.
- Calibration involves determining the actual volume delivered or contained by the glassware and correcting for any discrepancies.
-
Standardization of Titrants: The concentration of the titrant must be accurately known. Standardizing the titrant against a primary standard ensures the titrant's concentration is precisely determined.
- A primary standard is a highly pure, stable compound that can be accurately weighed and used to determine the concentration of the titrant.
-
Use of Controls and Blanks: Running control experiments (without the analyte) can help identify any systematic errors or contamination. Blank titrations can account for any background interference.
- These controls provide a baseline against which to compare the results of the actual titrations.
By meticulously addressing these potential sources of error and implementing careful techniques, it is possible to achieve highly accurate and reliable titration results. Mastering these practices is essential for any chemist seeking to perform quantitative analysis with confidence.
Video: Equivalence Point Explained: Master Titration Now!
FAQs About Equivalence Point in Titration
Hopefully, this FAQ section addresses some common questions about equivalence points and titration.
What exactly is the equivalence point in a titration?
The equivalence point is the point in a titration where the moles of titrant added are stoichiometrically equivalent to the moles of the analyte in the sample. Essentially, the acid and base have completely neutralized each other according to the balanced chemical equation.
How do you find the equivalence point during a titration?
Experimentally, you find the equivalence point by monitoring a physical property that changes drastically at or near the equivalence point. This can be done using an indicator (like phenolphthalein), a pH meter, or by observing a precipitate forming.
Is the equivalence point always at pH 7?
No, the equivalence point is not always at pH 7. This is only true when titrating a strong acid with a strong base. When a weak acid or weak base is involved, the resulting salt will hydrolyze, leading to an equivalence point that is not pH neutral.
Why is understanding the equivalence point so important in titrations?
Knowing the equivalence point allows you to accurately determine the concentration of the unknown analyte. By knowing the volume of titrant required to reach the equivalence point and the titrant's concentration, you can calculate the moles of analyte and, therefore, its concentration. This is the fundamental goal of most titrations.