Earthquake Focus: Everything You Need to Know!
The Earth's crust, a vital entity in understanding seismic activity, influences earthquake focus, the point of rupture underground. The Richter scale, developed by Charles Richter, serves as a fundamental tool for quantifying the magnitude related to earthquake focus. Understanding earthquake focus is crucial for areas within the Pacific Ring of Fire, a region known for its heightened seismic activity. Seismographs are important instruments that allows scientists to measure and to analyze the characteristics of the earthquake focus.
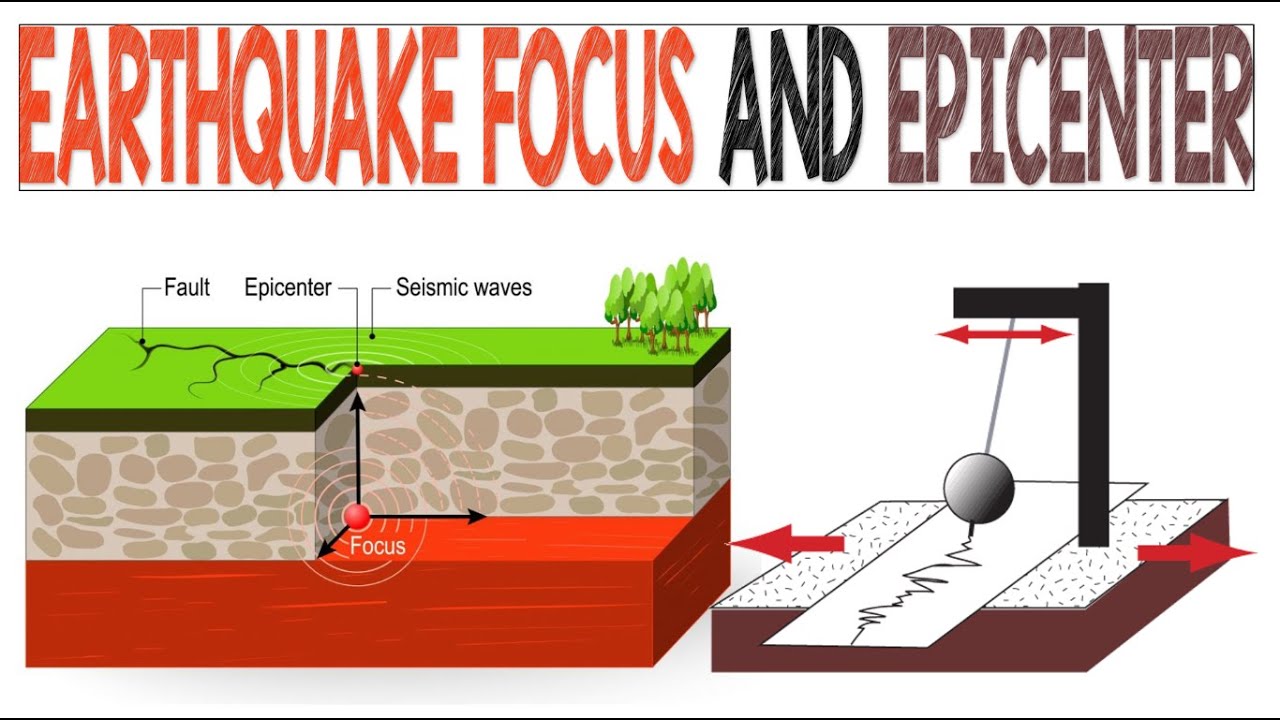
Image taken from the YouTube channel EarthPen , from the video titled Earthquake Focus and Epicenter | Animation .
Earthquakes, those sudden and often devastating tremors that shake our planet, are a stark reminder of the immense power contained within the Earth. Their impact can be catastrophic, leading to widespread destruction of infrastructure, loss of life, and long-term economic disruption. Understanding these phenomena is not merely an academic exercise; it's a matter of survival for millions who live in earthquake-prone regions.
At the heart of understanding earthquakes lies the concept of the earthquake focus, also known as the hypocenter. This single point deep within the Earth is where the rupture initiates, where the accumulated stress finally overcomes the friction and rigidity of the rocks, unleashing a torrent of energy in the form of seismic waves.
What is an Earthquake?
An earthquake is, in essence, the result of a sudden release of energy in the Earth's lithosphere, creating seismic waves. This energy radiates outwards from the focus, causing the ground to shake, sometimes violently. The effects can range from barely perceptible tremors to powerful ground motions that flatten buildings and trigger landslides.
The destructive potential of an earthquake depends on several factors, including the magnitude of the energy released, the depth of the focus, the geological conditions of the affected area, and the quality of construction of buildings and infrastructure. Earthquakes can also trigger secondary hazards, such as tsunamis, which can cause even greater devastation in coastal regions.
The Significance of the Earthquake Focus
The earthquake focus is much more than just a point of origin; it's a key to unlocking deeper insights into earthquake behavior. By accurately locating the focus, seismologists can begin to understand the fault rupture process, the stresses that led to the earthquake, and the potential for future events in the area.
Understanding the depth of the focus, for example, is crucial. Shallow-focus earthquakes, which occur closer to the Earth's surface, tend to be more destructive than deeper earthquakes of the same magnitude because the energy has less distance to dissipate before reaching the surface.
The location of the focus relative to known fault lines also provides valuable information about the tectonic forces at play in the region. It can help scientists understand how stress is accumulating along these faults and where future ruptures are most likely to occur.
The Imperative of Preparedness and Mitigation
Given the destructive potential of earthquakes, it is vital to prioritize earthquake preparedness and risk mitigation. This involves a multi-faceted approach, including:
-
Investing in earthquake-resistant construction: Buildings and infrastructure should be designed and built to withstand the forces of ground shaking.
-
Developing early warning systems: These systems can provide valuable seconds or even minutes of warning before strong shaking arrives, allowing people to take protective actions.
-
Educating the public: People need to know what to do before, during, and after an earthquake.
-
Conducting research: Continuous research is crucial to improve our understanding of earthquakes and develop more effective mitigation strategies.
Ultimately, our ability to minimize the impact of earthquakes depends on our understanding of these complex phenomena. By studying the earthquake focus and the processes that lead to earthquakes, we can better protect ourselves and build more resilient communities.
Earthquakes, as we’ve established, are a manifestation of the Earth's dynamic nature, with the earthquake focus serving as the point of origin. But to truly understand the spatial context of these seismic events, it's essential to move beyond simply identifying the where and begin understanding how we pinpoint it. This leads us to two critical concepts: the hypocenter and the epicenter. These terms, while often used interchangeably, represent distinct locations that are crucial for accurately describing an earthquake and understanding its potential impact.
Locating the Earthquake's Source: Hypocenter and Epicenter
The ability to pinpoint the exact location of an earthquake's origin is fundamental to understanding its behavior and potential impact. This requires differentiating between two key points: the hypocenter (or focus) and the epicenter.
Defining the Hypocenter (Focus)
The Origin Point Within the Earth
The hypocenter, also known as the focus, is the actual location within the Earth where the earthquake rupture begins. It's the three-dimensional point where the accumulated stress finally overcomes the rock's strength, initiating the fault slip and releasing energy in the form of seismic waves.
Think of it as the ignition point of the earthquake, hidden beneath the surface. Understanding its depth is critical, as deeper earthquakes tend to cause less surface shaking than shallower ones of the same magnitude, although they can be felt over a wider area.
The Process of Energy Release
At the hypocenter, the rocks along a fault line have been subjected to immense pressure over long periods. Eventually, this stress exceeds the frictional forces holding the rocks together.
When this threshold is crossed, a sudden rupture occurs, causing the rocks to slip past each other. This rapid movement releases a tremendous amount of energy, radiating outwards as seismic waves.
This process can be visualized as snapping a tightly wound spring. The point where the spring breaks is analogous to the hypocenter, and the energy released is similar to the seismic waves that travel through the Earth.
The Epicenter's Significance
The Relationship Between Hypocenter and Epicenter
The epicenter is the point on the Earth's surface directly above the hypocenter. It is essentially the geographical location that is most directly affected by the earthquake's initial energy release.
While the hypocenter is the source of the earthquake, the epicenter is the point most people will associate with the event, as it's the location usually reported in news and media.
The Surface Location Directly Above the Focus
The epicenter is determined by projecting a vertical line from the hypocenter to the Earth's surface. It is a two-dimensional location, defined by latitude and longitude, making it easier to locate on a map.
The epicenter is not necessarily the point of greatest damage. The distribution of damage depends on factors such as the direction of rupture, the geological composition of the ground, and the quality of construction in the area.
However, it serves as a crucial reference point for emergency responders and researchers alike, providing a starting point for assessing the scope and impact of the earthquake. Without the knowledge of both, it would be difficult to paint a complete picture of the event and how best to respond.
Earthquakes, as we’ve established, are a manifestation of the Earth's dynamic nature, with the earthquake focus serving as the point of origin. But to truly understand the spatial context of these seismic events, it's essential to move beyond simply identifying the where and begin understanding how we pinpoint it. This leads us to two critical concepts: the hypocenter and the epicenter. These terms, while often used interchangeably, represent distinct locations that are crucial for accurately describing an earthquake and understanding its potential impact.
Seismic Waves: The Messengers of Earthquakes
The energy released at the earthquake's focus doesn't simply vanish; it radiates outwards in the form of seismic waves. These waves act as crucial messengers, carrying information about the earthquake's location, magnitude, and even the Earth's internal structure.
Understanding the generation, types, and behavior of these waves is paramount to earthquake seismology. Without them, we would be blind to the Earth's inner workings and unable to effectively locate and characterize seismic events.
The Birth of Seismic Waves
At the hypocenter, the sudden rupture of rock generates intense vibrations that propagate outwards in all directions. This is the birthplace of seismic waves. The energy released transforms into a complex interplay of different wave types, each with unique characteristics.
The initial fracturing and subsequent fault slip send a cascade of energy through the surrounding rock, initiating the various seismic wave patterns.
Types of Seismic Waves: A Primer
Seismic waves are broadly categorized into two main types: body waves and surface waves. Body waves travel through the Earth's interior, while surface waves propagate along the Earth's surface.
Each type provides distinct insights into both the earthquake event and the properties of the materials they traverse.
Body Waves: Probing the Earth's Depths
Body waves consist of two subtypes: primary waves (P-waves) and secondary waves (S-waves).
P-waves: The Speed Demons
P-waves, or primary waves, are compressional waves. This means that the particle motion is parallel to the direction of wave propagation, similar to sound waves. They are the fastest seismic waves and can travel through solid, liquid, and gaseous materials. This allows them to traverse the Earth's core.
S-waves: Shear Waves with Limitations
S-waves, or secondary waves, are shear waves. Here, the particle motion is perpendicular to the direction of wave propagation. S-waves can only travel through solids. Their inability to pass through liquids provides critical evidence for the Earth's liquid outer core.
Surface Waves: The Ground Rollers
Surface waves, as the name suggests, travel along the Earth's surface and are responsible for much of the ground shaking experienced during an earthquake. They are generally slower than body waves and have larger amplitudes.
Love Waves: Horizontal Shakers
Love waves are surface waves with a horizontal shearing motion, perpendicular to the direction of propagation. They are typically faster than Rayleigh waves.
Rayleigh Waves: Rolling Motions
Rayleigh waves produce a rolling, elliptical motion of the ground, similar to waves on water. They are often the most destructive type of seismic wave due to their amplitude and the ground motion they generate.
Locating the Earthquake: Triangulation with Seismic Waves
The different speeds of P- and S-waves are crucial for determining the location of an earthquake. Seismographs, sensitive instruments that detect and record ground motion, are strategically placed around the world.
By analyzing the arrival times of P- and S-waves at multiple seismograph stations, scientists can use a process called triangulation to pinpoint the earthquake's hypocenter.
The time difference between the arrival of the P-wave and the S-wave (the "S-P interval") increases with distance from the earthquake. Using this information from at least three seismograph stations, a location can be determined. Where the distance circles intersect marks the epicenter.
Tectonic Plates and Fault Lines: Where Earthquakes are Born
Seismic waves have revealed much about earthquakes, but what geological processes give rise to them in the first place? The answer lies deep within the Earth, in the dynamic interplay of tectonic plates and the fractures that crisscross our planet's crust: fault lines.
These fundamental elements of Earth's geology are the primary drivers behind the vast majority of seismic events, transforming imperceptible movement into cataclysmic shaking.
Tectonic Plates: The Earth's Ever-Shifting Puzzle Pieces
The Earth's lithosphere, the rigid outer layer, is not a single, unbroken shell. It's fragmented into approximately fifteen major and numerous minor tectonic plates.
These plates, composed of both continental and oceanic crust, float atop the semi-molten asthenosphere.
Driven by convection currents in the mantle below, these plates are in constant, albeit slow, motion. This movement, though often measured in just centimeters per year, has profound consequences.
The grinding, colliding, and sliding of these plates against each other are the root cause of many earthquakes.
Plate Boundaries: Earthquake Epicenters
The boundaries between tectonic plates are, unsurprisingly, the most seismically active regions on Earth. These boundaries are zones of intense geological activity, where plates interact in three primary ways:
-
Convergent Boundaries: Where plates collide. One plate often subducts (slides) beneath the other, creating immense pressure and friction. This process leads to powerful earthquakes, such as those experienced in subduction zones like Japan or Chile.
-
Divergent Boundaries: Where plates move apart. As plates separate, magma rises from the mantle to fill the gap, creating new crust. This process is usually associated with weaker, shallower earthquakes, such as those along the Mid-Atlantic Ridge.
-
Transform Boundaries: Where plates slide horizontally past each other. The friction between the plates as they grind past each other generates significant stress, which can be released in the form of large earthquakes. The San Andreas Fault in California is a prime example of a transform boundary.
Fault Lines: Fractures Under Pressure
While plate boundaries represent large-scale interactions, fault lines are fractures within the Earth's crust where localized movement occurs.
These fractures can exist within a single plate or along a plate boundary. Fault lines are inherent weaknesses in the Earth's crust, zones where the accumulated stress is most likely to be released.
Stress Buildup and Release: The Earthquake Cycle
Over time, the movement of tectonic plates causes stress to accumulate along fault lines. The rocks on either side of the fault become locked together by friction, resisting movement.
This stress can build for decades, centuries, or even millennia.
Eventually, the stress exceeds the strength of the rocks, and the fault ruptures. This sudden release of energy is what we experience as an earthquake.
The energy radiates outwards in the form of seismic waves, shaking the ground and causing damage. The cycle then begins anew, as stress gradually builds up along the fault line once more, setting the stage for the next earthquake.
Understanding the relationship between tectonic plates, fault lines, and stress buildup is crucial for assessing earthquake hazards and developing strategies for risk mitigation.
Tectonic plates may set the stage, but understanding the real-world impact of earthquakes requires a way to quantify their strength. Scientists have developed sophisticated tools and scales to measure the energy released, providing crucial data for understanding earthquake behavior and its consequences.
Measuring Earthquake Strength: Richter and Moment Magnitude Scales
Quantifying an earthquake's size is crucial for understanding its potential impact and for informing effective responses. Two primary scales are used for this purpose: the Richter Scale and the Moment Magnitude Scale. Each scale offers a different approach to measuring earthquake intensity and magnitude, with its own strengths and limitations.
The Richter Scale: A Logarithmic Measurement
Developed by Charles F. Richter in 1935, the Richter Scale was one of the first widely used methods for measuring earthquake magnitude. It measures the amplitude of the largest seismic wave recorded on a seismograph.
The scale is logarithmic, meaning that each whole number increase on the Richter Scale represents a tenfold increase in the amplitude of the seismic waves. For example, an earthquake with a magnitude of 6.0 on the Richter Scale has seismic waves ten times larger than an earthquake with a magnitude of 5.0.
This logarithmic progression also translates to energy released: each whole number increase represents roughly a 31.6 times increase in energy. So, a magnitude 7.0 earthquake releases about 31.6 times more energy than a magnitude 6.0 earthquake.
The Richter Scale is particularly useful for measuring small to moderate earthquakes that occur locally. However, it tends to underestimate the magnitude of very large earthquakes. This limitation led to the development and widespread adoption of the Moment Magnitude Scale.
The Moment Magnitude Scale: A More Comprehensive Measure
The Moment Magnitude Scale (Mw) is now the standard scale used by seismologists for measuring the magnitude of earthquakes, especially larger ones. It provides a more accurate estimate of earthquake size because it considers several factors beyond just the amplitude of seismic waves.
Unlike the Richter Scale, which relies on a single measurement from a seismograph, the Moment Magnitude Scale calculates the seismic moment, which is related to the physical size of the fault rupture, the amount of slip on the fault, and the rigidity of the rocks.
This calculation provides a more comprehensive measure of the total energy released by the earthquake.
The Moment Magnitude Scale is better suited for measuring larger earthquakes, as it doesn't suffer from the saturation effect that limits the Richter Scale. This "saturation" refers to the tendency of the Richter Scale to level off and underestimate the magnitude of very large events.
The Moment Magnitude Scale is considered a more reliable indicator of an earthquake’s true size, particularly for events exceeding magnitude 7.0.
The Role of Seismographs in Earthquake Measurement
Both the Richter Scale and the Moment Magnitude Scale rely on data collected by seismographs. A seismograph is an instrument that detects and records ground motion caused by seismic waves.
Modern seismographs are highly sensitive and can detect even the smallest vibrations in the Earth's surface. The data recorded by seismographs, called seismograms, provides a detailed record of the arrival times, amplitudes, and frequencies of seismic waves.
Seismograph data is crucial for determining not only the magnitude of an earthquake, but also its location (hypocenter and epicenter) and depth. By analyzing the arrival times of P-waves and S-waves at multiple seismograph stations, seismologists can pinpoint the origin of the earthquake.
The amplitude and characteristics of the recorded seismic waves are then used to calculate the earthquake's magnitude using either the Richter Scale (for smaller, local events) or the Moment Magnitude Scale (for larger, more distant events). Seismograph networks around the world continuously monitor seismic activity, providing vital data for earthquake research, hazard assessment, and early warning systems.
Global Earthquake Hotspots: The Pacific Ring of Fire and Beyond
Having established the means by which earthquake strength is measured, it's time to turn our attention to where these seismic events are most likely to occur. Earthquakes aren't randomly distributed across the globe; rather, they cluster in specific zones dictated by the dynamics of our planet's tectonic plates.
The Pacific Ring of Fire: A Zone of Intense Seismic Activity
The Pacific Ring of Fire is perhaps the most well-known and geographically significant earthquake zone. This horseshoe-shaped region encircles the Pacific Ocean, tracing the boundaries of several tectonic plates.
It’s characterized by a high concentration of volcanoes and earthquake epicenters, making it a global hotspot for seismic activity.
Tectonic Drivers Behind the Ring of Fire
The intense seismic activity within the Ring of Fire is primarily driven by the subduction of oceanic plates beneath continental plates or other oceanic plates.
Along these subduction zones, one plate is forced beneath another, generating immense friction and stress. This stress accumulates over time until it exceeds the strength of the rocks, resulting in sudden slippage and the release of energy in the form of earthquakes.
The depth of these earthquakes can vary significantly, ranging from shallow events near the surface to deep-focus earthquakes hundreds of kilometers below.
The Ring of Fire accounts for approximately 90% of the world's earthquakes and is home to many of the planet's largest and most devastating seismic events.
Beyond the Ring: Other Significant Earthquake Zones
While the Pacific Ring of Fire dominates global earthquake statistics, other regions also experience significant seismic activity. These zones, though perhaps less widely known, pose considerable risk to local populations and infrastructure.
The Alpide Belt
Extending from Southern Europe, through Turkey, Iran, and into Northern India, the Alpide belt is another major earthquake zone.
This belt is the result of the collision between the Eurasian and African, as well as the Indian tectonic plates. The ongoing collision creates compressional forces. This leads to frequent earthquakes across the region.
Countries like Italy, Greece, Turkey, Iran, and Pakistan are all highly vulnerable to earthquakes due to their location within this seismic belt.
Mid-Atlantic Ridge
While primarily known for its volcanic activity, the Mid-Atlantic Ridge, a divergent plate boundary running down the center of the Atlantic Ocean, also experiences earthquakes.
These earthquakes are generally smaller in magnitude compared to those in subduction zones. However, they are a persistent feature of this geologically active region.
Intraplate Earthquakes
It's important to note that earthquakes can also occur within the interior of tectonic plates, far from plate boundaries. These so-called intraplate earthquakes are less frequent than those at plate boundaries, but they can still be powerful and destructive.
The mechanisms driving intraplate earthquakes are not always well understood. They are often attributed to ancient faults or zones of weakness within the plate.
The New Madrid Seismic Zone in the central United States is a prime example of an intraplate region capable of generating significant earthquakes.
Having explored the global distribution of earthquakes, it's clear that understanding these phenomena is not merely an academic exercise. It's a critical imperative for safeguarding lives and property. This necessitates robust monitoring systems, in-depth research, and proactive hazard assessment, spearheaded by dedicated organizations around the world.
Monitoring and Research: Understanding Earthquake Hazards
The complexity of earthquake science demands constant vigilance and a commitment to unraveling the underlying mechanisms that trigger these events. Institutions like the United States Geological Survey (USGS) play a pivotal role in this endeavor, acting as sentinels, researchers, and knowledge disseminators. Their work encompasses a broad spectrum of activities, all aimed at mitigating the risks posed by earthquakes.
The Central Role of the USGS
The USGS stands as a cornerstone of earthquake monitoring and research in the United States and globally. Their multifaceted approach integrates real-time data collection, advanced scientific investigation, and public education, making them an indispensable resource for understanding and responding to seismic threats.
Real-time Monitoring and Earthquake Detection
At the heart of the USGS's mission is the operation of extensive seismic networks. These networks consist of seismometers strategically placed across the country and around the world.
These sophisticated instruments continuously record ground motion, detecting even the faintest tremors that might presage larger earthquakes.
The data collected is then transmitted to centralized processing centers, where algorithms and expert analysts work in tandem to identify, locate, and characterize seismic events in near real-time.
This rapid detection capability is crucial for issuing timely warnings and initiating emergency response protocols.
In-Depth Research and Seismic Studies
Beyond monitoring, the USGS is actively engaged in cutting-edge research to improve our understanding of earthquake physics. This includes studying fault behavior, analyzing historical earthquake data, and developing sophisticated computer models to simulate seismic activity.
These models help scientists to forecast potential earthquake scenarios and to assess the vulnerability of different regions.
Understanding the complexities of plate tectonics, fault mechanics, and wave propagation is crucial for refining our ability to predict and prepare for future earthquakes.
Assessing Earthquake Hazards and Risks
One of the USGS's most vital functions is assessing earthquake hazards and risks. This involves creating detailed maps that delineate areas prone to ground shaking, landslides, and other earthquake-related hazards.
These maps are essential tools for urban planners, engineers, and policymakers. They inform building codes, land-use regulations, and infrastructure development decisions, helping to minimize the potential impact of future earthquakes.
The USGS also conducts probabilistic seismic hazard assessments, which estimate the likelihood of earthquakes of different magnitudes occurring in specific areas over a given period.
This information is invaluable for insurance companies, emergency management agencies, and individuals making decisions about earthquake preparedness.
Public Education and Outreach Programs
Recognizing that knowledge is power, the USGS invests significantly in public education and outreach programs.
They provide a wealth of information about earthquakes, including how to prepare for them, what to do during an earthquake, and how to recover afterward.
This information is disseminated through websites, publications, workshops, and community events, reaching a diverse audience from schoolchildren to senior citizens.
By empowering the public with knowledge, the USGS helps to create more resilient communities that are better equipped to withstand the inevitable challenges posed by earthquakes.
Video: Earthquake Focus: Everything You Need to Know!
Frequently Asked Questions About Earthquake Focus
Here are some common questions about the focus of an earthquake and what it means.
What exactly is the earthquake focus?
The earthquake focus, also known as the hypocenter, is the point within the Earth where the earthquake rupture begins. Think of it as the precise spot where the energy is first released, setting off the seismic waves. Understanding the earthquake focus helps determine its depth and location.
How is the earthquake focus different from the epicenter?
The epicenter is the point on the Earth's surface directly above the earthquake focus. It's the location most often reported in news and seismic updates. While both are important, the focus describes the quake's origin within the Earth, while the epicenter is a surface point.
Why is knowing the depth of the earthquake focus important?
The depth of the earthquake focus significantly impacts the damage. Shallower earthquakes tend to cause more damage at the surface than deeper ones of similar magnitude. Studying earthquake focus depths helps scientists understand the tectonic processes in that region.
How do scientists determine the location of the earthquake focus?
Seismologists use data from seismographs located around the world. By analyzing the arrival times of seismic waves at different stations, they can triangulate the location of the earthquake focus. Analyzing the speed and type of waves reveals further details on earthquake focus location and depth.
So, now you've got a handle on earthquake focus! Hopefully, this has been a helpful deep dive. Go forth and tell your friends about what you've learned about the amazing things happening underground. Until next time!