Diatomic Elements: Properties, Uses & Safety
Diatomic elements, a foundational concept in chemistry, exhibit unique molecular properties due to their structure, which is comprised of two atoms bonded together. Linus Pauling, a prominent figure in the field of chemical bonding, significantly contributed to our understanding of these properties, particularly concerning electronegativity and bond strength within diatomic molecules. Industries utilizing gas chromatography, a powerful analytical technique, depend on the purity and stability of diatomic gases like nitrogen and hydrogen. Safety regulations established by organizations such as the Occupational Safety and Health Administration (OSHA) are vital when handling diatomic elements like chlorine, due to their potential hazards.
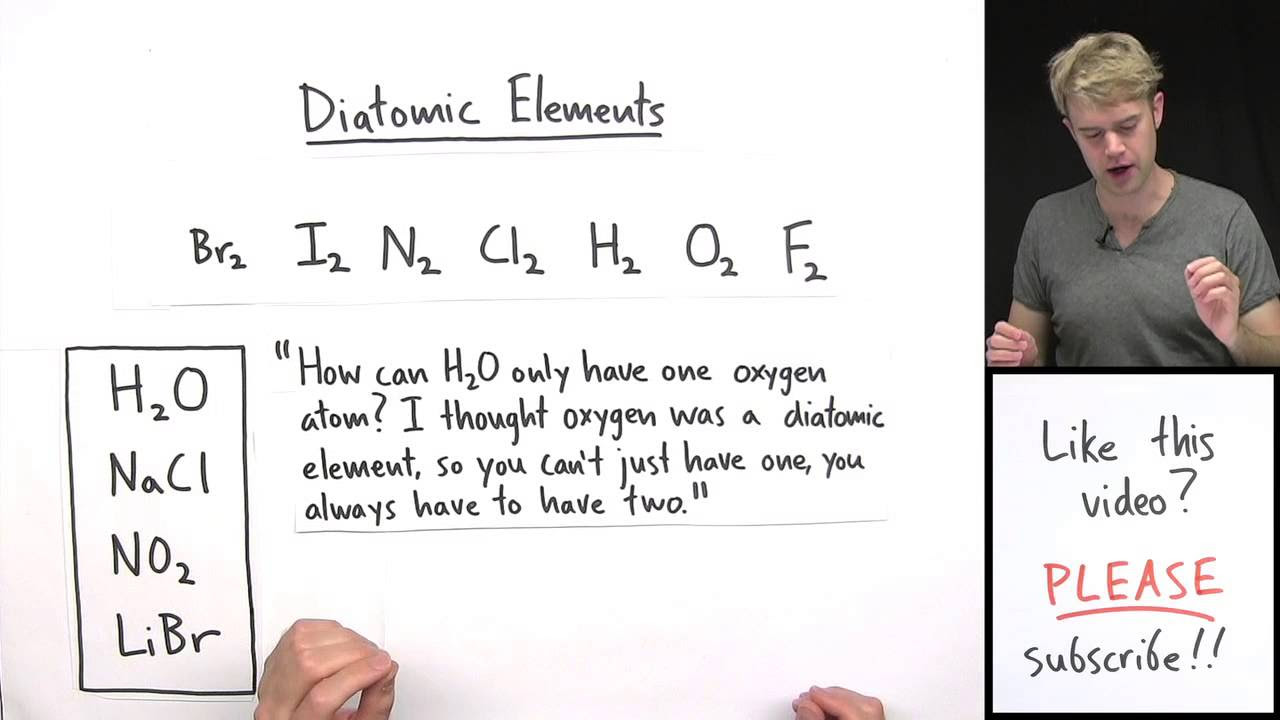
Image taken from the YouTube channel Tyler DeWitt , from the video titled Super Common Mistake: Diatomic Elements .
The Ubiquitous Diatomic Elements: Foundations of Life and Industry
Diatomic molecules, composed of two atoms of the same element bonded together, represent a fundamental class of chemical species. These molecules, far from being mere chemical curiosities, are integral to the very fabric of our existence and the engines of modern industry. Familiar examples include atmospheric mainstays such as oxygen (O₂) and nitrogen (N₂), as well as reactive elements like hydrogen (H₂) and chlorine (Cl₂).
Defining Diatomic Molecules
A diatomic molecule is formed when two atoms of the same element share electrons to achieve a more stable electronic configuration. These elements, under standard conditions, do not exist as single, independent atoms.
Instead, they pair up to form a molecule. This pairing is energetically favorable.
Classic instances of diatomic molecules include hydrogen (H₂), nitrogen (N₂), oxygen (O₂), fluorine (F₂), chlorine (Cl₂), bromine (Br₂), and iodine (I₂). These seven elements, collectively, account for a significant portion of the Earth's atmosphere and are critical building blocks in numerous chemical compounds.
The Drive for Stability: Why Diatomic Molecules Form
The existence of diatomic elements stems from the inherent drive of atoms to achieve electronic stability. Individual atoms of these elements possess incomplete valence shells, rendering them highly reactive.
By forming covalent bonds with another atom of the same element, they can share electrons. This sharing effectively completes their valence shells and achieves a more stable, lower-energy state.
For instance, oxygen (O) has six valence electrons and requires two more to complete its octet. By bonding with another oxygen atom to form O₂, each atom effectively achieves a full valence shell, resulting in a stable diatomic molecule.
Cornerstones of Life and Technological Progress
The significance of diatomic elements cannot be overstated. They are not simply abundant in the environment but are also deeply implicated in both sustaining life and driving industrial advancements.
Oxygen (O₂), for example, is essential for respiration, providing the energy that fuels nearly all living organisms. Nitrogen (N₂), the most abundant gas in the atmosphere, is a key component of proteins and nucleic acids, the building blocks of life.
Furthermore, diatomic elements play pivotal roles in numerous industrial processes. Hydrogen (H₂) is used in ammonia production and as a clean energy source in fuel cells. Chlorine (Cl₂) is indispensable in water purification and the production of various plastics and pharmaceuticals. These elements are essential in modern economies.
Key Players: Exploring Essential Diatomic Elements
Diatomic elements are not a monolithic group; rather, they exhibit a diverse array of properties and play distinct roles in the chemical landscape. Let us delve into the individual characteristics and applications of some of the most important diatomic elements.
Hydrogen (H₂)
Hydrogen, the most abundant element in the universe, exists as a diatomic molecule (H₂) under standard conditions. Its simplicity belies its significance.
Its unique properties, such as its small size and high reactivity, make it a cornerstone of many chemical processes.
Role in Chemical Reactions
Hydrogen plays a pivotal role in a vast number of chemical reactions. Combustion, for instance, is a prime example, where hydrogen reacts vigorously with oxygen to produce water and release substantial energy.
Applications
Hydrogen's versatility extends to a wide range of applications.
Fuel cells harness the chemical energy of hydrogen to generate electricity with water as the only byproduct.
The Haber-Bosch process, a groundbreaking innovation, utilizes hydrogen and nitrogen to synthesize ammonia, a critical component of fertilizers.
Other industrial applications include hydrogenation reactions in food processing and the production of various chemicals.
Nitrogen (N₂)
Nitrogen constitutes approximately 78% of the Earth's atmosphere. As a diatomic molecule (N₂), it is relatively inert due to the strong triple bond between the nitrogen atoms.
Biological Importance
Despite its inertness, nitrogen is indispensable for life. It is a crucial component of amino acids, the building blocks of proteins, and nucleic acids (DNA and RNA), the carriers of genetic information.
Industrial Uses
The Haber-Bosch process, mentioned earlier, is also the primary method for industrial nitrogen fixation, converting atmospheric nitrogen into ammonia-based fertilizers.
Nitrogen is also used to create inert atmospheres in various industrial processes, preventing unwanted reactions with oxygen.
Liquid nitrogen's extremely low temperature is exploited for cryopreservation and other cooling applications.
Oxygen (O₂)
Oxygen, vital for respiration and combustion, exists as a diatomic molecule (O₂) under normal conditions.
Discovery
The discovery of oxygen is often credited to Joseph Priestley, Carl Wilhelm Scheele, and Antoine Lavoisier, who independently investigated its properties.
Role in Respiration and Combustion
Oxygen is essential for aerobic respiration, the process by which organisms convert food into energy. It is also a key component of combustion, a rapid oxidation process that releases heat and light.
Medical and Industrial Applications
Oxygen finds widespread use in medical settings for oxygen therapy, assisting patients with respiratory problems.
Industrially, oxygen is used in steel production, welding, and various chemical manufacturing processes.
Fluorine (F₂)
Fluorine is the most electronegative element and exists as a highly reactive diatomic molecule (F₂). Its extreme reactivity necessitates careful handling.
Isolation
Humphry Davy is credited with isolating fluorine in 1807, although it took many years to fully characterize its properties due to its hazardous nature.
Applications
Fluorine's reactivity is leveraged in the production of various compounds. Fluoride compounds are added to toothpaste to prevent tooth decay.
Fluoropolymers, such as Teflon (PTFE), exhibit exceptional chemical resistance and are used in non-stick cookware and other applications.
Fluorine-containing pharmaceuticals are also prevalent, playing a role in various therapeutic treatments.
Chlorine (Cl₂)
Chlorine is a greenish-yellow gas at room temperature and exists as a diatomic molecule (Cl₂). While beneficial in controlled applications, chlorine can be dangerous in high concentrations.
Water Purification and Bleaching
Chlorine is a widely used disinfectant for water purification, effectively killing harmful bacteria and viruses. It is also a common bleaching agent for textiles and paper.
Chemical Synthesis and Other Applications
Chlorine is a versatile building block in chemical synthesis, used to produce a wide range of compounds. It's a key component in the production of PVC (polyvinyl chloride), a widely used plastic. Chlorine is also used in various disinfectant and cleaning products.
Bromine (Br₂)
Bromine is a reddish-brown liquid at room temperature that exists as a diatomic molecule (Br₂).
Applications
Bromine compounds find applications as flame retardants in plastics and textiles, inhibiting the spread of fire.
Bromine is also used in the production of certain dyes and pharmaceuticals.
Iodine (I₂)
Iodine is a dark-purple solid that sublimes to a violet gas at room temperature, existing as a diatomic molecule (I₂).
Antiseptics and Thyroid Function
Iodine is used as an antiseptic to prevent infection. It is also essential for the production of thyroid hormones, which regulate metabolism. Iodized salt is a common source of dietary iodine, preventing iodine deficiency.
Theoretical Underpinnings: Understanding Diatomic Bonding
Diatomic elements, seemingly simple in their composition, present a fascinating study in chemical bonding. Understanding why these elements exist as stable diatomic molecules requires a deep dive into the fundamental principles that govern their interactions. We will explore Molecular Orbital Theory, the nature of covalent bonding, electronegativity, and the crucial concepts of bond length and bond energy.
Molecular Orbital Theory and Diatomic Molecules
Molecular Orbital (MO) Theory provides a powerful framework for understanding bonding in diatomic molecules. Unlike simpler models that treat electrons as localized between two atoms, MO theory considers electrons delocalized across the entire molecule.
Atomic orbitals combine to form molecular orbitals, which can be either bonding or antibonding.
Bonding orbitals are lower in energy than the original atomic orbitals and promote stability when occupied. Antibonding orbitals are higher in energy and detract from stability.
The filling of these molecular orbitals with electrons dictates the overall stability and bond order of the diatomic molecule. For example, in the case of diatomic oxygen (O₂), the MO diagram shows a net positive bond order, confirming its stability as a diatomic molecule.
Covalent Bonding in Diatomic Elements
Covalent bonding is the primary type of chemical bond that holds diatomic molecules together. It involves the sharing of electrons between two atoms to achieve a stable electron configuration, typically resembling that of a noble gas.
In diatomic elements, each atom contributes electrons to the shared pair, forming a strong bond that holds the atoms together.
The strength of this covalent bond is directly related to the number of shared electron pairs; single, double, and triple bonds are all possible depending on the element involved. For instance, diatomic nitrogen (N₂) exhibits a very strong triple bond, contributing to its relative inertness.
Electronegativity and Bond Polarity
Electronegativity, a concept pioneered by Linus Pauling, is the measure of an atom's ability to attract electrons in a chemical bond. While diatomic elements consist of two identical atoms, understanding electronegativity helps to contextualize the nature of electron sharing in these molecules.
In a homonuclear diatomic molecule (e.g., H₂ or Cl₂), the electronegativity difference between the two atoms is zero. This results in a nonpolar covalent bond, where electrons are shared equally.
However, the concept of electronegativity becomes crucial when considering the potential for unequal sharing in more complex molecules. Pauling's scale provides a valuable tool for predicting the polarity of bonds based on electronegativity differences.
Bond Length and Bond Energy: Defining Molecular Stability
Bond length is the distance between the nuclei of two bonded atoms, while bond energy is the energy required to break that bond. These two parameters are intrinsically linked and play critical roles in determining the stability and reactivity of diatomic molecules.
Shorter bond lengths generally correspond to stronger bonds and higher bond energies. A high bond energy indicates a very stable diatomic molecule that requires significant energy input to dissociate.
For instance, the strong triple bond in N₂ results in a short bond length and a high bond energy, explaining its stability. Understanding these concepts is essential for predicting the behavior of diatomic elements in chemical reactions and various applications.
Diatomic Traits: Physical and Chemical Characteristics
Having understood the theoretical underpinnings of diatomic bonding, it is now crucial to examine how these bonding characteristics manifest in the observable properties of these elements. The physical and chemical behaviors of diatomic molecules are intrinsically linked to their molecular structure and intermolecular forces.
This section explores the distinct traits that define these essential elements, from their melting points and states of matter to their reactivity and involvement in chemical reactions.
Physical Properties: A Comparative Overview
The physical properties of diatomic elements, such as boiling points and states of matter, are markedly influenced by the strength of intermolecular forces. Generally, stronger intermolecular forces lead to higher boiling and melting points.
Boiling and Melting Points
Comparing the boiling and melting points across the diatomic series reveals insightful trends. Elements like nitrogen and oxygen, which exist as gases at room temperature, have relatively low boiling points due to weaker intermolecular forces.
In contrast, iodine, which is a solid at room temperature, exhibits a significantly higher melting and boiling point, indicative of stronger intermolecular interactions, specifically London dispersion forces that increase with molecular size.
States of Matter at Room Temperature
At room temperature, the diatomic elements span all three common states of matter. Hydrogen, nitrogen, oxygen, fluorine, and chlorine are gases, bromine is a liquid, and iodine is a solid.
This variation reflects the increasing strength of London dispersion forces as the number of electrons in the molecule increases. The stronger these forces, the more energy is required to overcome them, resulting in higher melting and boiling points.
Chemical Properties: Reactivity and Redox
The chemical properties of diatomic elements are governed by their electronic configurations and their ability to form chemical bonds. Their reactivity varies widely, influenced by factors such as electronegativity and bond strength.
Reactivity of Diatomic Elements
The reactivity of diatomic elements is intrinsically linked to their electronegativity and bond dissociation energy. For instance, fluorine is the most reactive diatomic element due to its high electronegativity and relatively weak F-F bond. This makes it a potent oxidizing agent, readily forming compounds with most other elements.
Nitrogen, on the other hand, is relatively inert due to the strong triple bond in N₂. This high bond energy makes it difficult to break the molecule apart and initiate reactions.
Diatomic Elements in Redox Reactions
Diatomic elements play crucial roles in oxidation-reduction (redox) reactions. Oxygen, as a strong oxidizing agent, is essential for combustion and respiration processes. It accepts electrons from other substances, leading to their oxidation.
Halogens, such as chlorine and fluorine, are also potent oxidizing agents, frequently used in disinfection and chemical synthesis. The ability of these elements to gain electrons and facilitate redox reactions is a cornerstone of many chemical and biological processes.
London Dispersion Forces in Diatomic Elements
London dispersion forces (LDFs) are the primary intermolecular forces present in nonpolar diatomic elements. These forces arise from temporary fluctuations in electron distribution, creating instantaneous dipoles.
The strength of LDFs increases with the size and number of electrons in the molecule. This is why heavier diatomic elements like iodine have higher melting and boiling points than lighter ones like hydrogen.
Allotropes: The Case of Ozone
Allotropes are different structural forms of the same element, exhibiting distinct physical and chemical properties. Oxygen provides a notable example with its two allotropes: diatomic oxygen (O₂) and ozone (O₃).
Ozone, a triatomic form of oxygen, is a powerful oxidizing agent and plays a critical role in absorbing harmful UV radiation in the stratosphere. Its chemical and physical properties differ significantly from diatomic oxygen due to its bent molecular structure and the presence of resonance structures, underscoring the profound impact of molecular arrangement on elemental behavior.
Safety First: Handling Diatomic Elements Responsibly
Having understood the theoretical underpinnings of diatomic bonding, it is now crucial to examine how these bonding characteristics manifest in the observable properties of these elements. The physical and chemical behaviors of diatomic molecules are intrinsically linked to their molecular structure, dictating their reactivity and potential hazards. Thus, the responsible handling of these fundamental substances necessitates a thorough understanding of their associated risks and the implementation of rigorous safety protocols.
Understanding Chemical Hazards: The Role of Safety Data Sheets
The cornerstone of any chemical safety program is the Material Safety Data Sheet (MSDS), now commonly referred to as the Safety Data Sheet (SDS). These documents are essential resources, providing comprehensive information about the properties of a substance, its potential hazards, and recommended safety measures.
The SDS includes crucial details like:
- Chemical identity and composition.
- Hazard identification (acute and chronic health effects, flammability, reactivity).
- First-aid measures.
- Fire-fighting measures.
- Accidental release measures.
- Handling and storage precautions.
- Exposure controls and personal protection.
- Physical and chemical properties.
- Stability and reactivity.
- Toxicological information.
- Ecological information.
- Disposal considerations.
- Transport information.
- Regulatory information.
A thorough review of the SDS is mandatory before working with any diatomic element. It is the professional obligation of every chemist and technician to remain well informed and act in accordance to all precautions mentioned in the SDS.
Emergency Response Guidance: The ERG
In the event of an accidental release or other hazardous material incident involving diatomic elements, the Emergency Response Guidebook (ERG) provides critical initial guidance for first responders. The ERG is designed for use by firefighters, police, and emergency medical services personnel who may be the first to arrive at the scene of a transportation incident involving hazardous materials.
The ERG offers information on:
- Identifying hazardous materials.
- Potential hazards associated with specific materials.
- Recommended evacuation distances.
- Appropriate personal protective equipment.
- Emergency response procedures.
The ERG is not a substitute for comprehensive training in hazardous materials handling, but it serves as a valuable quick reference in emergency situations.
NIOSH Pocket Guide: A Concise Chemical Safety Resource
The National Institute for Occupational Safety and Health (NIOSH) offers the NIOSH Pocket Guide to Chemical Hazards, a condensed source of essential information on chemical safety. This guide provides key data on hundreds of hazardous substances, including many diatomic elements.
Information included is:
- Chemical name and synonyms.
- CAS number.
- RTECS number.
- Exposure limits (PEL, TLV, IDLH).
- Physical description.
- Chemical and physical properties.
- Incompatibilities and reactivities.
- Measurement methods.
- Personal protective equipment recommendations.
- Respirator recommendations.
- First aid procedures.
The NIOSH Pocket Guide serves as a readily accessible reference for quick verification of vital safety data, supplementing the more comprehensive SDS.
Personal Protective Equipment (PPE): Minimizing Exposure Risks
Appropriate personal protective equipment (PPE) is essential for minimizing exposure risks when handling diatomic elements. The specific PPE required will vary depending on the element being handled, the concentration, the type of procedure being performed, and the potential hazards involved.
Common types of PPE include:
- Eye Protection: Safety glasses with side shields, goggles, or face shields are crucial to protect against splashes, vapors, or projectiles.
- Skin Protection: Appropriate gloves (e.g., nitrile, neoprene, or butyl rubber) should be selected based on the specific chemical being handled. Protective clothing, such as lab coats or aprons, may also be necessary to prevent skin contact.
- Respiratory Protection: In situations where exposure to hazardous vapors or gases is possible, a respirator may be required. The type of respirator (e.g., air-purifying respirator or self-contained breathing apparatus) will depend on the concentration of the contaminant and the oxygen level in the air.
- Ventilation: Working in a well-ventilated area or using a fume hood helps minimize the risk of inhaling hazardous vapors or gases.
The selection and use of PPE must be carefully considered, and personnel must be properly trained on how to use and maintain the equipment. Regular inspections and maintenance of PPE are also crucial to ensure its effectiveness.
Diatomic Elements in Action: Environmental and Industrial Roles
Having understood the theoretical underpinnings of diatomic bonding, it is now crucial to examine how these bonding characteristics manifest in the observable properties of these elements. The physical and chemical behaviors of diatomic molecules are intrinsically linked to their molecular structure, influencing their diverse applications and production methods across various sectors.
Production Methods: Harnessing Chemical Processes
The creation of diatomic elements for industrial use is a sophisticated endeavor, relying on well-established chemical processes that exploit the unique properties of these substances.
Electrolysis: Decomposing Compounds into Elements
Electrolysis, the process of using electrical current to drive non-spontaneous chemical reactions, plays a pivotal role in the production of chlorine and hydrogen gas.
In the chlor-alkali process, for example, an electric current is passed through a solution of sodium chloride (brine). This decomposes the water and sodium chloride into chlorine gas ($Cl2$) at the anode, hydrogen gas ($H2$) at the cathode, and sodium hydroxide ($NaOH$) remains in solution.
The reactions are as follows:
- Anode (oxidation): $2Cl^− → Cl
_2 + 2e^−$
- Cathode (reduction): $2H_2O + 2e^− → H
_2 + 2OH^−$
This process not only yields chlorine, a crucial disinfectant and industrial reagent, but also generates hydrogen, a potential clean energy carrier, and sodium hydroxide, a versatile base with numerous applications.
Cryogenic Distillation: Separating Air into Its Components
The air we breathe, primarily composed of nitrogen and oxygen, serves as a vast reservoir for these essential diatomic elements.
Cryogenic distillation, a technique that exploits the differences in boiling points of various gases, is the primary method for separating nitrogen and oxygen from atmospheric air.
Air is first purified to remove impurities like carbon dioxide and water vapor. Next, it is cooled to extremely low temperatures, liquefying the gases.
The liquid air is then slowly warmed, allowing nitrogen (boiling point -196°C) to boil off first, followed by oxygen (boiling point -183°C).
This fractional distillation process yields high-purity nitrogen and oxygen, essential for various industrial and medical applications.
Industrial Applications: Driving Innovation and Sustainability
The versatility of diatomic elements is reflected in their wide-ranging applications across numerous industries.
Chemical Manufacturing: Essential Building Blocks
Diatomic elements serve as essential building blocks and reactive intermediates in the synthesis of countless chemical compounds.
Nitrogen is a crucial component in the Haber-Bosch process, which synthesizes ammonia ($NH_3$) from nitrogen and hydrogen.
Ammonia is then used to produce fertilizers, supporting global food production. Chlorine is used in the production of polyvinyl chloride (PVC), a widely used plastic material with applications in construction, healthcare, and consumer goods.
Oxygen is used as an oxidizing agent in various chemical processes, including the production of steel and the synthesis of organic compounds.
Wastewater Treatment: Ensuring Water Quality
Chlorine plays a vital role in wastewater treatment, ensuring the safety and cleanliness of our water resources.
Chlorine gas or hypochlorite solutions are added to wastewater to disinfect it, killing harmful bacteria and viruses.
This process is essential for preventing the spread of waterborne diseases and protecting public health.
However, the use of chlorine in wastewater treatment also has potential drawbacks, such as the formation of disinfection byproducts (DBPs), some of which may be harmful to human health.
Research is ongoing to develop alternative disinfection methods that minimize the formation of DBPs while effectively eliminating pathogens.
Fuel Cells and Hydrogen Fueling Stations: Powering a Sustainable Future
Hydrogen, as a diatomic molecule ($H_2$), holds immense potential as a clean and sustainable energy carrier.
Fuel cells convert the chemical energy of hydrogen into electricity, producing only water as a byproduct.
Hydrogen fuel cells are being developed for various applications, including powering vehicles, providing backup power for buildings, and generating electricity for the grid.
The development of hydrogen fueling stations is essential for supporting the widespread adoption of hydrogen-powered vehicles. These stations provide a safe and convenient way to refuel hydrogen vehicles, similar to how gasoline stations fuel conventional cars.
However, the production and distribution of hydrogen currently face challenges, including the high cost of production, the need for efficient storage and transportation methods, and the development of a robust infrastructure for hydrogen fueling.
Overcoming these challenges is crucial for realizing the full potential of hydrogen as a clean energy source.
Video: Diatomic Elements: Properties, Uses & Safety
FAQs: Diatomic Elements
Why are some elements diatomic?
Certain elements are diatomic because their individual atoms are unstable. Bonding with another atom of the same element to form a molecule results in a lower energy state and greater stability. This covalent bonding satisfies the octet rule, making these diatomic elements more stable.
What physical properties are common among diatomic elements?
Many diatomic elements are gases at room temperature and pressure. This is due to the relatively weak intermolecular forces between the diatomic molecules. Their boiling points are generally low compared to elements that form larger, more complex structures.
What are some common uses of diatomic elements?
Oxygen (O2) is essential for respiration and combustion. Nitrogen (N2) is used in fertilizers and as a coolant. Chlorine (Cl2) is a disinfectant for water. These diverse applications highlight the importance of diatomic elements across various industries.
Are all diatomic elements equally dangerous?
No, the danger levels of diatomic elements vary greatly. While oxygen is essential for life, fluorine is highly reactive and corrosive. Similarly, chlorine gas is toxic. Safety precautions depend entirely on the specific properties of each diatomic element.
So, next time you hear about oxygen keeping us alive or chlorine disinfecting your pool, remember the fascinating world of diatomic elements. They might seem simple as bonded pairs, but their properties, uses, and safety considerations are anything but! Hopefully, this article has shed some light on these essential players in the chemical world.